

Environmental Impacts of Urban Growth
Research summary.
We explore and quantify the manifold impacts of urbanization on ecosystems and the services they provide.
In determining the effects of urbanization on the environment we draw data from weather stations, field interviews, satellite images, and governmental records. We develop new algorithms for processing this data, apply spatial statistical analysis to discover trends, and use coupled human–environment system models to predict future impacts.
Recent Findings
The conversion of Earth’s land surface to urban uses is one of the most irreversible human impacts on the global biosphere. It hastens the loss of highly productive farmland, affects energy demand, alters the climate, modifies hydrologic and biogeochemical cycles, fragments habitats, and reduces biodiversity ( Seto et al., 2011 ) We see these effects on multiple levels. Future urbanization will, for example, pose direct threats to high-value ecosystems: the highest rates of land conversion over the next few decades will likely take place in biodiversity hotspots that were relatively undisturbed by urban development in 2000 ( Seto et al., 2012 ). Within cities, the nature of urban growth is also an important determinant of urban dwellers’ vulnerability to environmental stress ( Güneralp and Seto, 2008 ).
The environmental impacts of urban expansion reach far beyond urban areas themselves. In rapidly urbanizing areas, agriculture intensifies on remaining undeveloped land and is likely to expand to new areas, putting pressure on land resources ( Jiang et al., 2013 ). Furthermore, urban areas change precipitation patterns at scales of hundreds of square kilometers ( Kaufman et al., 2007 ) . Urban expansion will affect global climate as well. Direct loss in vegetation biomass from areas with high probability of urban expansion is predicted to contribute about 5% of total emissions from tropical deforestation and land-use change ( Seto et al., 2012 ). The scope and scale of these impacts is yet to be fully researched. Although many studies have described how urbanization affects CO 2 emissions and heat budgets, effects on the circulation of water, aerosols, and nitrogen in the climate system are only beginning to be understood ( Seto & Shepherd, 2009 ).
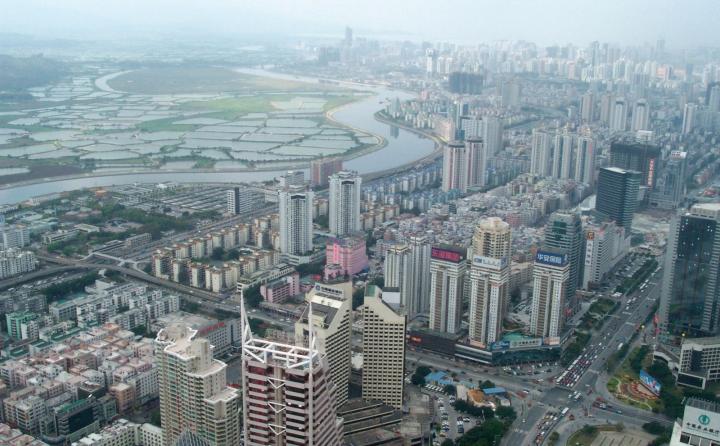
Related Publications
Global sustainability challenges, from maintaining biodiversity to providing clean air and water, are closely interconnected yet often separately studied and managed. Systems integration—holistic approaches to integrating various components of coupled human and natural systems—is critical to understand socioeconomic and environmental interconnections and to create sustainability solutions. Recent advances include the development and quantification of integrated frameworks that incorporate ecosystem services, environmental footprints, planetary boundaries, human-nature nexuses, and telecoupling. Although systems integration has led to fundamental discoveries and practical applications, further efforts are needed to incorporate more human and natural components simultaneously, quantify spillover systems and feedbacks, integrate multiple spatial and temporal scales, develop new tools, and translate findings into policy and practice. Such efforts can help address important knowledge gaps, link seemingly unconnected challenges, and inform policy and management decisions.
The aggregate potential for urban mitigation of global climate change is insufficiently understood. Our analysis, using a dataset of 274 cities representing all city sizes and regions worldwide, demonstrates that economic activity, transport costs, geographic factors, and urban form explain 37% of urban direct energy use and 88% of urban transport energy use. If current trends in urban expansion continue, urban energy use will increase more than threefold, from 240 EJ in 2005 to 730 EJ in 2050. Our model shows that urban planning and transport policies can limit the future increase in urban energy use to 540 EJ in 2050 and contribute to mitigating climate change. However, effective policies for reducing urban greenhouse gas emissions differ with city type. The results show that, for affluent and mature cities, higher gasoline prices combined with compact urban form can result in savings in both residential and transport energy use. In contrast, for developing-country cities with emerging or nascent infrastructures, compact urban form, and transport planning can encourage higher population densities and subsequently avoid lock-in of high carbon emission patterns for travel. The results underscore a significant potential urbanization wedge for reducing energy use in rapidly urbanizing Asia, Africa, and the Middle East.
We examine the impacts of urbanization on agricultural land loss in India from 2001 to 2010. We combined a hierarchical classification approach with econometric time series analysis to reconstruct land-cover change histories using time series MODIS 250 m VI images composited at 16-day intervals and night time lights ( NTL ) data. We compared estimates of agricultural land loss using satellite data with agricultural census data. Our analysis highlights six key results. First, agricultural land loss is occurring around smaller cities more than around bigger cities. Second, from 2001 to 2010, each state lost less than 1% of its total geographical area due to agriculture to urban expansion. Third, the northeastern states experienced the least amount of agricultural land loss. Fourth, agricultural land loss is largely in states and districts which have a larger number of operational or approved SEZs . Fifth, urban conversion of agricultural land is concentrated in a few districts and states with high rates of economic growth. Sixth, agricultural land loss is predominantly in states with higher agricultural land suitability compared to other states. Although the total area of agricultural land lost to urban expansion has been relatively low, our results show that since 2006, the amount of agricultural land converted has been increasing steadily. Given that the preponderance of India’s urban population growth has yet to occur, the results suggest an increase in the conversion of agricultural land going into the future.
That urban and rural places are connected through trade, people, and policies has long been recognized. The urban land teleconnections (ULT) framework aims advancing conventional conceptualizations of urbanization and land. The conceptual framework thus opens way to identify and examine the processes that link urbanization dynamics and associated land changes that are not necessarily colocated. In this paper, we review recent literature on four manifestations of urbanization that, along the lines of the ULT framework, highlight the importance of process-based conceptualizations of urbanization and land along a continuum of land systems. We then discuss potential approaches to improve the knowledge base on how and where urbanization is driving land change.
China’s urbanization has resulted in significant changes in both agricultural land and agricultural land use. However, there is limited understanding about the relationship between the two primary changes occurring to China’s agricultural land – the urban expansion on agricultural land and agricultural land use intensity. The goal of this paper is to understand this relationship in China using panel econometric methods. Our results show that urban expansion is associated with a decline in agricultural land use intensity. The area of cultivated land per capita, a measurement about land scarcity, is negatively correlated with agricultural land use intensity. We also find that GDP in the industrial sector negatively affects agricultural land use intensity. GDP per capita and agricultural investments both positively contribute to the intensification of agricultural land use. Our results, together with the links between urbanization, agricultural land, and agricultural production imply that agricultural land expansion is highly likely with continued urban expansion and that pressures on the country’s natural land resources will remain high in the future.
Urban areas consume more than 66% of the world’s energy and generate more than 70% of global greenhouse gas emissions. With the world’s population expected to reach 10 billion by 2100, nearly 90% of whom will live in urban areas, a critical question for planetary sustainability is how the size of cities affects energy use and carbon dioxide (CO 2 ) emissions. Are larger cities more energy and emissions efficient than smaller ones? Do larger cities exhibit gains from economies of scale with regard to emissions? Here we examine the relationship between city size and CO 2 emissions for U.S. metropolitan areas using a production accounting allocation of emissions. We find that for the time period of 1999–2008, CO 2 emissions scale proportionally with urban population size. Contrary to theoretical expectations, larger cities are not more emissions efficient than smaller ones.
Remote sensing offers unique perspectives to study the relationship between urban systems and climate change because it provides spatially explicit and synoptic views of the landscape that are available at multiple grains, extents, and over time. While remote sensing has made significant advances in the study of urban areas, especially urban heat island and urban land change, there are myriad unanswered science and policy questions to which remote sensing science could contribute. Here we identify several key opportunities for remote sensing science to increase our understanding of the relationships between urban systems and climate change.
While there is consensus that urbanization is one of the major trends of the 21st century in developing countries, there is debate as to whether urbanization will increase or decrease vulnerability to droughts. Here we examine the relationship between urbanization and water vulnerability for a fast-growing city, Chennai, India, using a coupled human–environment systems (CHES) modeling approach. Although the link between urbanization and water vulnerability is highly site-specific, our results show some generalizable factors exist. First, the urban transformation of the water system is decentralized as irrigation wells are converted to domestic wells by private individuals, and not by the municipal authority. Second, urban vulnerability to water shortages depends on a combination of several factors: the formal water infrastructure, the rate and spatial pattern of land use change, adaptation by households and the characteristics of the ground and surface water system. Third, vulnerability is dynamic, spatially variable and scale dependent. Even as household investments in private wells make individual households less vulnerable, over time and cumulatively, they make the entire region more vulnerable. Taken together, the results suggest that in order to reduce vulnerability to water shortages, there is a need for new forms of urban governance and planning institutions that are capable of managing both centralized actions by utilities and decentralized actions by millions of households.
Urbanization will place significant pressures on biodiversity across the world. However, there are large uncertainties in the amount and location of future urbanization, particularly urban land expansion. Here, we present a global analysis of urban extent circa 2000 and probabilistic forecasts of urban expansion for 2030 near protected areas and in biodiversity hotspots. We estimate that the amount of urban land within 50 km of all protected area boundaries will increase from 450 000 km 2 circa2000 to 1440 000 ± 65 000 km 2 in 2030. Our analysis shows that protected areas around the world will experience significant increases in urban land within 50 km of their boundaries. China will experience the largest increase in urban land near protected areas with 304 000 ± 33 000 km 2 of new urban land to be developed within 50 km of protected area boundaries. The largest urban expansion in biodiversity hotspots, over 100 000 ± 25 000 km 2 , is forecasted to occur in South America. Uncertainties in the forecasts of the amount and location of urban land expansion reflect uncertainties in their underlying drivers including urban population and economic growth. The forecasts point to the need to reconcile urban development and biodiversity conservation strategies.
Urbanization is a demographic, economic, and land transformation process. Building construction and operation are integral aspects of urban land use change and contribute to material and energy resources consumption and the resulting carbon dioxide (CO 2 ) emissions in urban areas. In this paper, we ask two questions regarding the urbanization process: 1) Do the land, material, and energy use efficiencies associated with the construction and operation of buildings increase over time? 2) Do the gains in resource use efficiencies offset the increases in resource demands due to the magnitude of urbanization? To answer these questions, we use a systematic approach similar to a material flow analysis and apply it to the Pearl River Delta, a rapidly urbanizing region in China. We use a combination of satellite data and official statistics to evaluate changes in urban population density and building density from 1988 to 2008. Both density measures decrease from 1988 to 2003; after 2003, building density increases while population density continues to decline. We also track the indirect impacts of urban land expansion on material and energy demands and associated CO 2 emissions using concrete and heating/cooling as proxies for building construction and operation, respectively. Throughout the study period, structural changes and efficiency gains decrease the demand per unit floor area for both building materials and energy. However, the efficiency gains are outstripped by the magnitude of urban expansion, therefore leading to an increase in the demand for resources and CO 2 emissions per capita. Our results show that focusing only on gains in efficiency for individual buildings without considering the scale of urban expansion results in underestimate of the cumulative energy, material, and greenhouse gas emissions impacts of urbanization. We emphasize the distinction between the rates versus the accumulations of these impacts over spatial and temporal scales. We discuss the relevance of the Environmental Kuznets approaches to tackling environmental impacts that are cumulative in nature and may lead to irreversible changes in the environment. We conclude that tracking the energy, materials, and emissions impacts of urbanization requires a multi-scale approach that ranges from the individual building to the urban region.
Urban land-cover change threatens biodiversity and affects ecosystem productivity through loss of habitat, biomass, and carbon storage. However, despite projections that world urban populations will increase to nearly 5 billion by 2030, little is known about future locations, magnitudes, and rates of urban expansion. Here we develop spatially explicit probabilistic forecasts of global urban land-cover change and explore the direct impacts on biodiversity hotspots and tropical carbon biomass. If current trends in population density continue and all areas with high probabilities of urban expansion undergo change, then by 2030, urban land cover will increase by 1.2 million km 2 , nearly tripling the global urban land area circa 2000. This increase would result in considerable loss of habitats in key biodiversity hotspots, with the highest rates of forecasted urban growth to take place in regions that were relatively undisturbed by urban development in 2000: the Eastern Afromontane, the Guinean Forests of West Africa, and the Western Ghats and Sri Lanka hotspots. Within the pan-tropics, loss in vegetation biomass from areas with high probability of urban expansion is estimated to be 1.38 PgC (0.05 PgC yr −1 ), equal to ∼5% of emissions from tropical deforestation and land-use change. Although urbanization is often considered a local issue, the aggregate global impacts of projected urban expansion will require significant policy changes to affect future growth trajectories to minimize global biodiversity and vegetation carbon losses.
This paper introduces urban land teleconnections as a conceptual framework that explicitly links land changes to underlying urbanization dynamics. We illustrate how three key themes that are currently addressed separately in the urban sustainability and land change literatures can lead to incorrect conclusions and misleading results when they are not examined jointly: the traditional system of land classification that is based on discrete categories and reinforces the false idea of a rural–urban dichotomy; the spatial quantification of land change that is based on place-based relationships, ignoring the connections between distant places, especially between urban functions and rural land uses; and the implicit assumptions about path dependency and sequential land changes that underlie current conceptualizations of land transitions. We then examine several environmental “grand challenges” and discuss how urban land teleconnections could help research communities frame scientific inquiries. Finally, we point to existing analytical approaches that can be used to advance development and application of the concept.
Aedes aegypti is implicated in dengue transmission in tropical and subtropical urban areas around the world. Ae. aegypti populations are controlled through integrative vector management. However, the efficacy of vector control may be undermined by the presence of alternative, competent species. In Puerto Rico, a native mosquito, Ae. mediovittatus , is a competent dengue vector in laboratory settings and spatially overlaps with Ae. aegypti . It has been proposed that Ae. mediovittatus may act as a dengue reservoir during inter-epidemic periods, perpetuating endemic dengue transmission in rural Puerto Rico. Dengue transmission dynamics may therefore be influenced by the spatial overlap of Ae. mediovittatus , Ae. aegypti , dengue viruses, and humans. We take a landscape epidemiology approach to examine the association between landscape composition and configuration and the distribution of each of these Aedes species and their co-occurrence. We used remotely sensed imagery from a newly launched satellite to map landscape features at very high spatial resolution. We found that the distribution of Ae. aegypti is positively predicted by urban density and by the number of tree patches, Ae. mediovittatus is positively predicted by the number of tree patches, but negatively predicted by large contiguous urban areas, and both species are predicted by urban density and the number of tree patches. This analysis provides evidence that landscape composition and configuration is a surrogate for mosquito community composition, and suggests that mapping landscape structure can be used to inform vector control efforts as well as to inform urban planning.
Editorial overview written for issue of Current Opinion in Environmental Sustainability entitled “Human settlements and industrial systems.” The overview discusses the confluence of urbanization and global environmental change and introduces articles found in the issue.
Contemporary urbanization differs from historical patterns of urban growth in terms of scale, rate, location, form, and function. This review discusses the characteristics of contemporary urbanization and the roles of urban planning, governance, agglomeration, and globalization forces in driving and shaping the relationship between urbanization and the environment. We highlight recent research on urbanization and global change in the context of sustainability as well as opportunities for bundling urban development efforts, climate mitigation, and adaptation strategies to create synergies to transition to sustainability. We conclude with an analysis of global greenhouse gas emissions under different scenarios of future urbanization growth and discuss their implications.
In 2008, the global urban population exceeded the nonrural population for the first time in history, and it is estimated that by 2050, 70% of the world population will live in urban areas, with more than half of them concentrated in Asia. Although there are projections of future urban population growth, there is significantly less information about how these changes in demographics correspond with changes in urban extent. Urban land-use and land-cover changes have considerable impacts on climate. It has been well established that the urban heat island effect is more significant during the night than day and that it is affected by the shape, size, and geometry of buildings as well as the differences in urban and rural gradients. Recent research points to mounting evidence that urbanization also affects cycling of water, carbon, aerosols, and nitrogen in the climate system. This review highlights advances in the understanding of urban land-use trends and associated climate impacts, concentrating on peer-reviewed papers that have been published over the last two years.
In lieu of an abstract, the following is a chapter excerpt:
China is home to one-fifth of the world’s population and that population is increasingly urban. The landscape is also urbanizing. Although there are studies that focus on specific elements of urban growth, there is very little empirical work that incorporates feedbacks and linkages to assess the interactions between the dynamics of urban growth and their environmental impacts. In this study, we develop a system dynamics simulation model of the drivers and environmental impacts of urban growth, using Shenzhen, South China, as a case study. We identify three phases of urban growth and develop scenarios to evaluate the impact of urban growth on several environmental indicators: land use, air quality, and demand for water and energy. The results show that all developable land will be urban by 2020 and the increase in the number of vehicles will be a major source of air pollution. Demand for water and electricity will rise, and the city will become increasingly vulnerable to shortages of either. The scenarios also show that there will be improvements in local environmental quality as a result of increasing affluence and economic growth. However, the environmental impacts outside of Shenzhen may increase as demands for natural resources increase and Shenzhen pushes its manufacturing industries out of the municipality. The findings may also portend to changes other cities in China and elsewhere in the developing world may experience as they continue to industrialize.
Remote sensing data have been proposed as a potential tool for monitoring environmental treaties. However, to date, satellite images have been used primarily for visualization, but not for systematic monitoring of treaty compliance. In this paper, we present a methodology to operationalize the use of satellite imagery to assess the impact of the Ramsar Convention on Wetlands. The approach uses time series analysis of landscape pattern metrics to assess land cover conditions before and after designation of Ramsar status to monitor compliance with the Convention. We apply the methodology to two case studies in Vietnam and evaluate the success of Ramsar using four metrics: (1) total mangrove extent; (2) mangrove fragmentation; (3) mangrove density; and (4) aquaculture extent. Results indicate that the Ramsar Convention did not slow the development of aquaculture in the region, but total mangrove extent has remained relatively constant, primarily due to replanting efforts. Yet despite these restoration efforts, the mangroves have become fragmented and survival rates for replanting efforts are low. The methodology is cost effective and especially useful to evaluate Ramsar sites that rely mainly on self-reporting methods and where third parties are not actively involved in the monitoring process. Finally, the case study presented in this paper demonstrates that with the appropriate satellite record, in situ measurements and field observations, remote sensing is a promising technology that can help monitor compliance with international environmental agreements.
The authors establish the effect of urbanization on precipitation in the Pearl River Delta of China with data from an annual land use map (1988–96) derived from Landsat images and monthly climate data from 16 local meteorological stations. A statistical analysis of the relationship between climate and urban land use in concentric buffers around the stations indicates that there is a causal relationship from temporal and spatial patterns of urbanization to temporal and spatial patterns of precipitation during the dry season. Results suggest an urban precipitation deficit in which urbanization reduces local precipitation. This reduction may be caused by changes in surface hydrology that extend beyond the urban heat island effect and energy-related aerosol emissions.
The ability to predict spatial patterns of species richness using a few easily measured environmental variables would facilitate timely evaluation of potential impacts of anthropogenic and natural disturbances on biodiversity and ecosystem functions. Two common hypotheses maintain that faunal species richness can be explained in part by either local vegetation heterogeneity or primary productivity. Although remote sensing has long been identified as a potentially powerful source of information on the latter, its principal application to biodiversity studies has been to develop classified vegetation maps at relatively coarse resolution, which then have been used to estimate animal diversity. Although classification schemes can be delineated on the basis of species composition of plants, these schemes generally do not provide information on primary productivity. Furthermore, the classification procedure is a time- and labour-intensive process, yielding results with limited accuracy. To meet decision-making needs and to develop land management strategies, more efficient methods of generating information on the spatial distribution of faunal diversity are needed. This article reports on the potential of predicting species richness using single-date Normalized Difference Vegetation Index (NDVI) derived from Landsat Thematic Mapper (TM). We use NDVI as an indicator of vegetation productivity, and examine the relationship of three measures of NDVI—mean, maximum, and standard deviation—with patterns of bird and butterfly species richness at various spatial scales. Results indicate a positive correlation, but with no definitive functional form, between species richness and productivity. The strongest relationships between species richness of birds and NDVI were observed at larger sampling grains and extent, where each of the three NDVI measures explained more than 50% of the variation in species richness. The relationship between species richness of butterflies and NDVI was strongest over smaller grains. Results suggest that measures of NDVI are an alternative approach for explaining the spatial variability of species richness of birds and butterflies.
We have compared the official estimates of agricultural land and rates of agricultural land conversion with those derived from Landsat thematic mapper satellite images for 10 counties in the Pearl River Delta, which is one of the fastest-developing regions in China. Ground- based field assessments verify the high accuracy of our techniques in estimating the area of agricultural land and its change through time. Our results indicate that there is significantly more agricultural land than reported in official statistics. Although this underreporting is well documented, particularly using coarse resolution (1-km) satellite data sets, our study is the first to use high-resolution satellite imagery to quantify this bias.

An official website of the United States government
The .gov means it’s official. Federal government websites often end in .gov or .mil. Before sharing sensitive information, make sure you’re on a federal government site.
The site is secure. The https:// ensures that you are connecting to the official website and that any information you provide is encrypted and transmitted securely.
- Publications
- Account settings
Preview improvements coming to the PMC website in October 2024. Learn More or Try it out now .
- Advanced Search
- Journal List
- Int J Environ Res Public Health

Impact of Urbanization on Ecosystem Health: A Case Study in Zhuhai, China
1 School of Life and Environmental Science, Minzu University of China, Beijing 100081, China; moc.kooltuo@4991naniuC (N.C.); nc.ude.cum@43210381 (R.H.)
2 Centre for Spatial Analysis and Policy, School of Geography, University of Leeds; Leeds LS2 9JT, UK
Chen-Chieh Feng
3 Department of Geography, National University of Singapore, Singapore 117570, Singapore; [email protected]
The past decades have witnessed rapid urbanization around the world. This is particularly evident in Zhuhai City, given its status as one of the earliest special economic zones in China. After experiencing rapid urbanization for decades, the level of ecosystem health (ESH) in Zhuhai City has become a focus of attention. Assessments of urban ESH and spatial correlations between urbanization and ESH not only reveal the states of urban ecosystems and the extent to which urbanization affected these ecosystems, but also provide new insights into sustainable eco-environmental planning and resource management. In this study, we assessed the ESH of Zhuhai City using a selected set of natural, social and economic indicators. The data used include Landsat Thematic Mapper images and socio-economic data of 1999, 2005, 2009 and 2013. The results showed that the overall ESH value and ecosystem service function have been on the decline while Zhuhai City has continued to become more urbanized. The total ESH health level trended downward and the area ratio of weak and relatively weak health level increased significantly, while the areas of well and relatively well healthy state decreased since 1999. The spatial correlation analysis shows a distinct negative correlation between urbanization and ESH. The degree of negative correlation shows an upward trend with the processes of urban sprawl. The analysis results reveal the impact of urbanization on urban ESH and provide useful information for planners and environment managers to take measures to improve the health conditions of urban ecosystems.
1. Introduction
Urbanization is one of the main forces driving environmental and ecological change [ 1 ]. Characterized by population aggregation, urban expansion and economic development [ 2 , 3 , 4 ], urbanization has resulted in conversions of ecological land to constructed land, and as a result the conversions of natural ecosystems into human-dominated and coupled human-nature ecosystems [ 5 , 6 , 7 ]. Specifically, urbanization disrupts the flows of materials, energy and information and the structures and functions of ecosystems [ 8 ]. Nonetheless, the increasing population and urban development demand greater ecological services for the sustainability of social development [ 9 ], which gives rise to a mutually affecting relationship between urbanization and urban eco-environment [ 10 ]. Thus, monitoring the state of ecosystems and quantifying the effects of urbanization on urban ecosystems have become an important means for effective urban landscape planning and eco-environmental policy making.
The meaning of health in this context was extended from medical science to describe the state of regional ecosystems in the 1980s [ 11 , 12 ]. The change motivated a new concept, urban ecosystem health, which assists urban environment managers with integrating ecological, economic, social and human health factors and includes not only the health and integrity of natural and built environments, but also of urban residents and the whole society [ 13 , 14 , 15 ]. This research topic was driven by extensive public concern and decades of progress in ecosystem health research [ 16 , 17 , 18 ]. However, due to the complexity of ecosystems, it is challenging to develop a precise operational definition and find a uniform index system to evaluate their health conditions [ 19 ]. To address this issue, Costanza [ 20 ] provided the concept of ecosystem health (ESH), which was defined as the ability of an ecosystem to keep its original state and maintain its organizational structure. In addition, a healthy ecosystem can recover with its self-regulating processes after being disturbed by human activities [ 20 ]. ESH can be measured by using the indices related to three main aspects, i.e., vigor, resilience and organization [ 21 ]. These indices can assist environment managers to assess ecosystem states, and are conducive to the sustainable development of ecosystems. Vigor means that a system is active and has plenty of energy to maintain its health; organization refers to different components that exist between which some relationships can be found that which can make the system more stable and effective; and resilience describes whether a system can recover from interference and maintain its stable structure [ 22 ]. Adding to the notion of ESH by Costanza [ 20 ], Myneni [ 23 ] highlighted that ESH is comprehensive and multi-scale.
Recently, researchers have provided many methods for ESH assessment. For instance, landscape metrics were used to assess whether an ecosystem is health in a city landscape [ 13 ], and five indicators associated with ecosystem pressure and response were selected to describe estuary ecosystem health [ 24 ]. Nevertheless, among these methods, many studies have continued to use the framework of vigor-organization-resilience to investigate ecosystem health. Spatial correlations exhibited in these indices can exert crucial influences on ecological processes at the regional scale, which is the common scale at which ESH studies were conducted and environmental protection plans were made. However, in urban areas, some studies only selecting the indicators of vigor, organization and resilience when researching the regional ecosystem health. Specifically, a healthy regional ecosystem provides a range of valuable services sustainably, which is a primary design goal for ecological engineering benefit both humans and the entire natural world [ 25 ]. Thus a comprehensive method considering the four indicators of vigor, organization, resilience and ecosystem services were selected by researchers [ 25 , 26 , 27 ] to obtain better assessment results in various regions.
Land use could also cause massive changes in ESH largely, and many studies have discussed the influence of the relationship between land use changes and ESH. Restoration projects such as the Grain to Green Program have made great contribution to solving environmental problems including soil erosion, flooding and desertification. Other researchers have investigated the influence of ecological protection on ESH by combining remote sensing images and geographic information system (GIS) techniques. However, most studies paid attention to the changes of ESH but fell short of assessing the effects of urbanization on urban ESH. For example, Liao et al. [ 26 ] assessed the relationship between changes in ESH and land use changes. Wang et al. [ 28 ] assessed the regional ecological health of Xiamen City, China. Both studies did not explore the effects of urbanization on ESH. Peng et al. [ 25 ] discussed the relationship between urbanization and changes of regional ESH levels in the early stage of the Chinese economic reform (1978–2005), but they did not consider the spatial patterns of regional ESH levels due to urbanization. In short, existing studies leave two gaps that require attention. First, there is a need to examine the clustering patterns between ESH and urbanization, especially at the local scale. Spatial correlations are commonly found between ESH and their drivers (including urbanization), leading to biases in the results obtained by ordinary least squares (OLS) and geographically weighted regression. Therefore, other statistical techniques dealing with spatial autocorrelation must be employed. Second, previous studies have focused on the regional scale, with administrative districts as the usual unit of spatial analysis. Analysis on this scale is underlain by a process aggregating locally collected data into meso-or macro-levels. This may limit the practical applicability of ESH in local urban landscape planning [ 29 ].
Additionally, remote sensing images are also instrumental in assessing and investigating ESH across space and time at temporal and spatial scales in an area [ 30 , 31 ]. For example, Liao et al. [ 22 ] built a pressure-state-response framework using remote sensing data and ecological service values in an assessment of regional ESH. Sun et al. [ 27 ] measured the ecosystem health of the Liaohe River Delta in China by combining remote sensing images, GIS technology and ecosystem services at the watershed scale.
The novelty of this study stems from the use of time series land use data from 1999, 2005, 2009 and 2013 and four main factors of vigor, organization, resilience and ecosystem services (ESV) to assess urban ESH. In addition, the clustering patterns between ESH and urbanization have been analyzed for their spatial auto correlative characteristics at the local scale. Compared with previous studies, of which the analyses were performed at the regional scale or using administrative districts, this study investigated the spatial relationships between ESH and urbanization at the local scale, which provides fine-grained recommendations for future urban ecological planning. Zhuhai City is studied because it has experienced continual and rapid urbanization, because it is designated as one of the special economic zones (SEZs) in China for its economic reform campaign in 1980 [ 32 ]. Since then, Zhuhai City has experienced more than 30 years of rapid urbanization, which has resulted in a dramatic decline in environmental quality and the destruction of natural landscape [ 33 ]. The specific aims of the study include the following: (1) assessing and quantifying the ESH and urbanization level in Zhuhai City using remote sensing images, gross domestic product (GDP) data and population data, (2) investigating spatial correlations between ESH and three kinds of urbanization and (3) assessing the spatial dependence of ESH on urbanization based on spatial regression models (SRMs), including spatial lag model (SLM) and spatial error model (SEM).
2. Materials and Methods
2.1. study area and data source, 2.1.1. study area.
Zhuhai City is one of the major cities of Guangdong Province in southern China. It is situated on the west side of the Pearl River Estuary ( Figure 1 ). The climate in Zhuhai is subtropical-monsoon-maritime. The urban land, population and economy experienced fast growth in the past three decades [ 32 ]. In 2013, the total land area of Zhuhai City was 1711 km 2 . The population increased more than fourfold, from 0.36 million in 1979 to 1.56 million in 2010. As a result, the economic structure in Zhuhai has undergone a fundamental shift. Agriculture and fisheries were the main industries in the early 1980s, but with the development of economy, transportation, high-tech, secondary and tertiary industries gradually made great contributions to the GDP, transforming Zhuhai into a modern city [ 34 ].

Location of Zhuhai City.
2.1.2. Data Source
Land use maps were gathered from remote sensing images taken in 1999, 2005, 2009 and 2013, and used to extract normalized difference vegetation index (NDVI) to measure the values of vigor, organization, resilience and ecosystem services. Population and GDP data with a spatial resolution of 1 km × 1 km were obtained from the National Science and Technology Infrastructure of China ( http://www.geodata.cn ) [ 35 ].
The aforementioned sets of remote sensing imageries were also used to extract the land use land cover types needed for this study, which include farmland, developed land, wasteland, forestland, water body, grassland and unutilized land, through supervised classification. The kappa values of the classification results are all above 8.5, suggesting that the land use land cover maps developed are of sufficient accuracy for the purpose of assessing ESH.
2.2. Assessment of Ecosystem Health
In urban areas, it is well-known that there exists coupled relationships between human and natural systems. To assess the ESH of Zhuhai City, we used the following four indices: vigor, organization, resilience and ecosystem services (ESV). The ESH is evaluated with two variables, the physical health (PH), consisting of three indices, i.e., vigor, organization, resilience [ 20 ] and the ecosystem services (ESV) of Zhuhai City. The equations for calculating ESH are as follows:
where H is the ESH of the units being assessed, PH is the physical health of the ecosystem, ESV is the ecosystem services, and V, O and R refer to the vigor, organization and resilience of spatial entities.
To capture ecosystem vigor, which refers to the ecosystem’s metabolism or primary productivity, NDVI is used because it has been proven to be effective in assessing the primary productivity of vegetation [ 36 , 37 ]. The NDVI is calculated using Landsat satellite images with multispectral bands in different times [ 38 , 39 ]. Ecosystem organization refers to the structural stability of the ecosystems. It is acknowledged that spatial patterns are essential influencing factors in the management of ecosystem processes at a landscape scale [ 40 ]. In this study, ecosystem organization is determined by examining the spatial heterogeneity in landscape patterns, which can be measured through landscape diversity using Shannon’s diversity index (SHDI) and landscape fractal dimension using the area-weighted mean patch fractal dimension (AWMPFD) [ 41 ]. In this study, the formula used to calculate the ecosystem organization is as follows:
where O is ecosystem organization of the spatial entities, SHDI is Shannon’s diversity index, AWMPFD is the index of area-weighted mean patch fractal dimension, the calculation was run using Fragstats 4.2.
Resilience is the ability of a natural ecosystem to recover to its original structure and functions after external disturbance. The formula used to calculate ecosystem resilience is as follows [ 25 ]:
where ER is the ecosystem resilience of spatial entities, A i is the area ratio of land use type i ; R i is the ecosystem resilience coefficient of land use type i and n is the number of land use types. In order to generate the comparable study results, the values of ESH in four years studied will be divided into the following five levels: well (80–100), relatively well (60–80), ordinary (40–60), relatively weak (20–40) and weak (0–20).
2.3. Quantifying Ecosystem Services
This study quantified the ecosystem services of Zhuhai by following the approaches in Costanza et al. [ 42 ] and Xie et al. [ 43 ]. We extracted the market price of cereals punished in The Guangdong Statistical year books of 1999, 2005, 2009 and 2013 available from the National Library of China official website ( http://www.nlc.cn ) [ 44 ], and calculated the average ESV of one equivalent value for Zhuhai City, which was 1539.02 yuan/(ha*a). Using the area ratio of different land use types, the total ecosystem values of each grid in the study area were derived.
2.4. Mapping Urbanization Levels
Urbanization levels can be investigated from several respects: (1) population growth, which is the main feature of the development of a modern city; (2) economic development, which is the main means of urban development; (3) the expansion of constructed land, which links directly to population growth and economic development and (4) living standards improvement, which is the result of urbanization [ 45 ]. As it is difficult to obtain accurate socioeconomic data that measures the improvement of living standards, let alone assesses their spatial heterogeneity, this study used only the first three kinds of data to represent urbanization levels: the population growth by population density (POPD; person km −2 ), economic development by density of gross domestic product (GDPD; 10 4 yuan km −2 ) and expansion of constructed land by constructed area proportion (CAP) [ 46 , 47 , 48 ].
2.5. Spatial Correlation Test
The relationship between ESH and urbanization can be investigated by using bivariate Moran’s I ( Figure 2 ). In our study, we explored the spatial correlation from two aspects, spatial clustering (positive spatial correlation) and spatial dispersion (negative spatial correlation), based on global bivariate Moran’s I and local bivariate Moran’s I (bivariate local indicators of spatial association (LISA)). Global bivariate Moran’s I mainly focus on investigating spatial correlations between ESH and urbanization on large scale or across the entire study areas such as Zhuhai City, while local bivariate Moran’s I prefer to evaluate the spatial correlations within different spatial units, such as grids in this study [ 49 ]. The formulas used to calculate Moran’s I are as follows:
where I eu and I’ eu refer to the bivariate Moran’s I for ESH and different urbanization levels, respectively; N refers to the number of grids; w ij refers to the spatial weight matrix for calculating spatial correlations between pairs of adjacent spatial units, which was generated based on queen contiguity weight with the first order neighbor in a 3 × 3 matrix [ 50 , 51 ] and z j e and z j u refer to the standardized value of ESH and urbanization indicators (GDPD, CAP and POPD) of each spatial grid using Equation (5). The values of I eu /I′ eu range from –1 to 1, indicating the neighboring grid cells have distinctive and similar values, respectively. In addition, they provide measures of the magnitude urbanization influences ESH. The larger the value is, the greater the impact of urbanization on ESH. The study carried out 999 permutations to test the significance ( p < 0.05) in this context [ 52 ].

Assessment of spatial correlation between urbanization and ecosystem health (ESH). GDPD, density of gross domestic product; CAP, constructed area proportion; POPD, population density.
Bivariate LISA produces graphical outputs including Moran scatter plots, cluster maps and corresponding significance maps to help users visualize local spatial correlations. They illustrate the relationship between the value of ESH at a given location and the average value of urbanization level at neighboring locations at a certain significance level. The four quadrants of a cluster map thus generated represent four types of local spatial autocorrelation: quadrant I (high–high type, HH) indicates high ESH values surrounded by high urbanization values; quadrant II (high–low type, HL) indicates high ESH values surrounded by low urbanization values; quadrant III (low–high type, LH) indicates low ESH values surrounded by high urbanization values and quadrant IV (low–low type, LL) indicates low ESH values surrounded by low urbanization values.
3.1. Assessment of Urban Ecosystem Health
Figure 3 shows the results of ESH values obtained for the four years studied using the five levels described in Section 2.2 . Overall, the areas categorized as weak and relatively weak increased from 1999 to 2013. More specifically, between 1999 and 2005, the proportion of the study area characterized as relatively well or better was well above 50%. After 2005, however, the ESH of the study area deteriorated. The proportion of total areas categorized as relatively well and well dropped below 50%, to 45.82% in 2013, an indication that the urban ecosystem had gradually become unhealthy with the progress of urbanization.

With different ecosystem health levels in 1999, 2005, 2009 and 2013.
The spatial patterns of the ESH levels of Zhuhai City from 1999 to 2013 are shown in Figure 4 . In 1999, the areas categorized as weak were mainly distributed in Meihua, Jida and Gongbei Town, which are the main urban areas of Zhuhai City. They were also found at the border of Jingan Town and Baijiao Town, and the aviation industry park area in the south of Zhuhai City. The areas categorized as relatively weak were mainly distributed in Doumen Town, Ganwu Town, Pingsha Town, Hongqi Town, North of Hongqi Town and Baiteng streets. The areas categorized as ordinary were scattered throughout the region of Zhuhai City, mainly around the areas of relatively weak health. The areas categorized as relatively well and well were mainly distributed in Jingan, Doumen and the border of Ganwu Town where several forest parks in these areas, such as Baizu Mountain, Zhuzi Ridge and Xinping Mountain are located. In addition, Nanshui Town in the south of Zhuhai City, Sanzao Town and the southern part of Hengqin Town also belong to relatively well and well areas. As far as the eastern part of Zhuhai City, the areas categorized as relatively well include: the College Town, the bonded area and the Nanping Science and Technology Park. These areas are distributed in the mountain areas and forest reserves in Zhuhai, so the environment in these areas was well protected by the city government of Zhuhai.

Spatial patterns of urban ecosystem health in 1999, 2005, 2009 and 2013.
In 2005, the ESH in the northeastern part of the study area had deteriorated given that the weak ESH areas level had expanded. The health states of the ordinary weak or relatively weak were further reduced. For example, the areas around Baizu Mountain in the west part of Zhuhai City experienced a decline in ESH levels in this period and the border areas with well and relatively well health of Nanping Town, Free Trade Zone and Hengqin Town also decreased into the lower ESH.
In 2009, the most obvious characteristic of urban ESH was that the areas with weak health level drastically increased, especially the areas with relatively weak health level in 2005. This result was the same as that in Figure 3 , which shows that the proportion of weak health areas increased significantly. For instance, areas categorized as weak health in the northern part of College Town, the junction of Hengqin Town and the bonded area, as well as Doumen Town, Ganwu Town, Jing’an Town and Sanzhao Town increased significantly.
Compared to other years, areas with a weak health level in 2013 were the highest, and almost all the relatively weak areas in 1999 deteriorated to weak level in 2013. The same trend was also found in other levels of ESH, e.g., the areas in the north part of the university campus, Baizu Mountains and northern areas of Nanshui Town deteriorated to weak level of health from 2009 to 2013. Overall, in 2013, only 45.82% of areas throughout Zhuhai City had a well and relatively well healthy urban ecosystem. The results suggested that it is necessary to take active protective measures in the development of Zhuhai City and impose effective regulations to protect the well and relatively well areas.
3.2. Spatial Distribution of Three Kinds of Urbanization Level
In order to verify the relationships between rapid urbanization and urban ecological health, the study mapped the GDP density (GDPD), constructed area percentage (CAP) and population density (POPD) of Zhuhai City during the study period ( Figure 5 ). It could be found that the spatial distribution of GDPD, CAP and POPD carry similar patterns and temporal trends with the progress of urbanization. The area with the highest level of urbanization was in the city center, gradually decreasing from the city center to peripheral areas. The economic urbanization as described by GDPD was highest in the eastern part of the study area, especially in the main city area, in 1999, 2005, 2009 and 2013. In addition, the economic urbanization level in the southern part of the city also drastically increased during 2009–2013. Land urbanization, which is represented by CAP, was the highest in the main city and gradually decreased to the lowest at the periphery in 1999. While similar in the spatial patterns, differences between economic and land urbanization are obvious. The areas with high land urbanization levels could be found around city parks (e.g., Bailiandong Park, Marina Park and City Park) and ecological conservation zone (e.g., Banzhang Mountain, Shijing mountain and Shihua Mountain) with the development of the city. It seems clear that the constructed area in the study area formed several centers around Jinan Town, Sanzao Town and Doumen Town instead of one center within Zhuhai City. In addition, many areas exhibited sudden drops of constructed area percentage. As for population density, the highest level was found only in Zhuhai City Center. This shows that economic development and the immigration of the population occurred later than urban land expansion in the progress of urban urbanization. It also suggests the need to pay more attention to urban sprawl in the study area in case of possible ecosystem damage.

Urbanization levels in Zhuhai City. GDPD, GDP density; CAP, constructed area proportion; POPD, population density.
3.3. Effect of Urbanization on Urban Ecosystem Health
Table 1 shows the results of Moran’s I analysis of GDP density (GDPD), population density (POPD) and constructed area proportion (CAP). All results are below 0, suggesting that urbanization measured by the three indicators had a negative impact on ESH. It can be inferred that the areas with low ESH values may be surrounded by or adjacent to areas with high urbanization. Additionally, different kind of urbanization exerted various negative effects across years.
Moran’s I between ESH and GDPD, POPD and CAP.
IUL | Year | 1999 | 2005 | 2009 | 2013 |
---|---|---|---|---|---|
GDPD | Moran’s I | –0.0484 | –0.1198 | –0.1477 | –0.2463 |
z-Value | –4.107 | –19.84 | –12.66 | –15.64 | |
-Value | 0.001 | 0.001 | 0.001 | 0.001 | |
POPD | Moran’s I | –0.0883 | –0.1654 | –0.1255 | –0.2619 |
z-Value | –7.516 | –26.04 | –8.322 | –16.49 | |
-Value | 0.001 | 0.001 | 0.001 | 0.001 | |
CAP | Moran’s I | –0.2039 | –0.2001 | –0.2738 | –0.257 |
z-Value | –15.99 | –15.87 | –21.18 | –19.66 | |
-Value | 0.001 | 0.001 | 0.001 | 0.001 |
In 1999, the largest negative impact of urbanization was found between land urbanization and ESH, indicating that urban expansion had a stronger impact than the other two types of urbanization. In 2005, a negative correlation between the three kinds of urbanization and ESH showed a similar trend as in 1999, but the degree of negative correlation between population and economic urbanization visibly increased, indicating their increased influence on the deterioration of ESH. In 2009 and 2013, it could be found that the degree of negative correlation between all kinds of urbanization and ESH continuously increased with the development of the city.
Figure 6 shows the spatial correlations between ESH and urbanization levels. The figure reveals similar clustering patterns of spatial correlation. The HH areas for GDPD and ESH were mainly concentrated in the northern and southern parts of the main areas of Zhuhai City in 1999. With the development of the urban economy, the size of HH areas decreased significantly especially, in the period 2009–2013. The HH areas for CAP and ESH only accounted for a small proportion in four years, and they were scattered across the whole study area. In 2013, the HH areas occupied only 3.08% of the total study area. The HH areas for POPD and ESH showed a similar trend with that in HH areas for GDPD and ESH from 1999 to 2013. The proportions of the kind of areas drastically decreased with the increased population.

Local indicators of spatial association (LISA) cluster maps between ESH and individual urbanization level (IUL; GDPD: GDP density; CAP: constructed areaproportion; POPD: population density; HH: high ESH and high IUL; HL: high ESH and low IUL; LH: low ESH and high IUL; LL: low ESH and low IUL).
The HL areas for GDPD and ESH were mainly distributed in the western part of Zhuhai and gradually deceased from 1999 to 2013. They occupied 20.34% of the total study area. The HL areas for CAP and ESH were scattered in the southern part in Zhuhai City in 1999 and 2005, then clustered in the southern part. The HL areas for POPD and ESH were mainly concentrated in northeastern Zhuhai in 1999, then decreased with the increased population in 2005, 2009 and 2013.
The LL areas for GDPD and ESH were distributed in the northwestern part of Zhuhai City, then decreased along with GDPD growth during the period 1999–2013. In 2013, the LL areas for GDPD and ESH occupied about 7.76% of the total study area. The LL areas for CAP and ESH were scattered across the whole study area, but decreased with the processes of the urban expansion. In addition, the LL areas only accounted for 2.82% of the total area. The LL areas for POPD and ESH were mainly distributed across the western part of the study areas and other LL areas were clustered in the southwestern part of Zhuhai City in 1999. Over time, they showed a decreasing trend with the increasing population from 2005–2013.
The LH regions for GDPD and ESH were concentrated in the major city and northeastern part of the study area throughout the study period. They then decreased with GDPD growth. By 2013, the LH regions occupied 6.78% of the total study area. The LH areas for CAP and ESH were concentrated in the main city across the whole study period. At the same time, some areas in western Zhuhai belonged to this kind of area. The LH areas for POPD and ESH showed the same trend as GDPD and ESH. They also concentrated in northeastern Zhuhai City in 2009. In 2013, they occupied 6.41% of the total study area.
3.4. Spatial Dependence of ESH on Urbanization
The results of ordinary least squares (OLS) regressions, which are presented in Table 2 , show spatial dependencies in all regressions. In addition, the spatial dependencies were more significant between the LM lag and LM error than OLS for all ES regressions, so SEM was used for GDP density (GDPD), population density (POPD) and constructed area proportion (CAP) regression in this study.
Results of ordinary least squares (OLS) regressions between ESH and IUL.
Dependent | ESH1999 | ESH2005 | ESH2009 | ESH2013 |
---|---|---|---|---|
Constant | 0.50621 | 0.620177 | 0.664083 ** | –82.5178 |
GDPD | 0.195904 ** | 0.148384 | 0.120648 * | –159.412 |
POPD | –0.00491 | –0.159545 | 0.168452 ** | –438.546 |
CAP | –0.757281 ** | –0.656092 | –0.945341 ** | 173.391 |
R | 0.199883 | 0.242939 | 0.389663 | 0.005089 |
Log likelihood | 165.233 | 176.358 | –34.5155 | –11255.2 |
AIC | –322.465 | –344.716 | 77.0309 | 22518.5 |
SC | 302.395 | –324.53 | 96.992 | 22539.3 |
Moran’s I | 20.7746 ** | 11.9284 ** | 18.0845 ** | 9.1422 ** |
Lagrange multiplier (lag) | 367.6217 ** | 127.6153 ** | 262.6840 ** | 79.0298 ** |
Robust LM (lag) | 1.0614 | 2.51 | 6.4710 * | 0.1599 |
Lagrange multiplier (error) | 419.5618 ** | 136.4934 ** | 316.9356 ** | 79.6533 ** |
Robust LM (error) | 53.0015 | 11.3888 ** | 60.7225 ** | 0.7833 |
GDPD, GDP urbanization; CAP, constructed area proportion; POPD, population urbanization; AIC, Akaike information criterion; SC, Schwarz criterion. * p -values at 5% level. ** p -values at 1% level.
The results of R 2 and log likelihood in spatial regression were higher in spatial regression models (SRMs) than OLS, and the Akaike information criterion (AIC) and Schwartz criterion (SC) values were lower in SRMs than OLS, which indicated that the results in SRMs are more reliable than that in OLS for all regressions ( Table 2 and Table 3 ). In order to obtain the relative effect of every index, we analyzed the regression coefficients. The results of the error coefficient (lambda) were significantly positive ( p < 0.01) in SEM for all regressions, indicating that the non-urbanization factors also exert positive influences on all three indicators. The coefficients between IUL and all ESH indices showed that urbanization resulted in the decline of ESH. In addition, there was an increasing trend in the absolute values of CUL coefficients between 1999 and 2005 and between 2005 and 2009, implying that the impact of urban expansion on ESH is becoming more pronounced.
Results of spatial regressions between ESH and IUL.
Dependent variables | ESH1999 | ESH2005 | ESH2009 | ESH2013 |
---|---|---|---|---|
LAMBDA | 0.613031 ** | 0.420321 ** | 0.563871 ** | 0.425095 ** |
Constant | 0.517883 | 0.611472 ** | 0.660955 ** | –110.963 |
GDPD | 0.113996 ** | 0.133592 ** | 0.094901 | –130.042 |
POPD | –0.00579 | –0.148248 ** | 0.140503 * | –557.4 |
CAP | –0.747333 ** | –0.626769 ** | –0.888831 ** | 250.482 |
R | 0.415862 | 0.325404 | 0.524507 | 0.089752 |
Log likelihood | 300.803312 | 225.35109 | 69.3103 | –11215.7 |
AIC | –593.607 | –422.702 | –130.621 | 22439.3 |
SC | –573.537 | –422.516 | –110.66 | 22460.1 |
AIC denotes Akaike information criterion. SC denotes Schwarz criterion. LAMBDA denotes spatial error term of ESH in 1999, 2005, 2009 and 2013. * The values of Pat 5% level. ** The values of Pat 1% level.
4. Discussion
4.1. esh in zhuhai city changed from 1999 to 2013.
In this study, ESH in Zhuhai City was first assessed in 1999, 2005, 2009 and 2013, then the assessment results were divided into five levels: weak, relatively weak, ordinary, relatively well and well. The changes in areas corresponding to different levels from 1999 to 2013 showed that the overall quality of urban ecosystem health decreased during the period of study, indicating that urban planners and environment managers should pay more attention to protecting ESH. Additionally, Zhuhai has experienced rapid urbanization for 30 years since the commencement of the economic reform in China. The expanded constructed area and rapidly increasing population have caused an obvious decline in ESH [ 53 ]. These results are similar to studies in other rapidly developing cities in China such as Shanghai [ 46 ], Shenzhen [ 25 ] and Beijing [ 54 ]. From the temporal perspective, the results showed a clear declining trend in ecosystem conditions from 1999 to 2013, as the areas with weak health level increased by nearly six times during this period. On the other hand, relatively weak areas showed a declining trend rather than a dramatic increase, the same trend was found for ordinary areas, and these two kinds of ESH levels accounted for the relatively low percentage in all four years. Additionally, well and relatively well areas showed a slightly decreasing trend and the proportion of them decreased about 10% of the total study area, this phenomenon indicates that the whole study area, including the four levels of ESH, was on a downward trend instead of a specific class of ESH converting to a weak health level ecological system. It signals the urgent need to protect the whole urban ecosystem rather than only pay attention to weak and relatively weak regions.
In terms of spatial dimensions, we evaluated the spatial distribution of urban ecological system health for all four years. The results show that the weak areas mainly located in the city center, urban areas and constructed areas, and these kinds of areas constantly increased from 1999 to 2013. This implies that Zhuhai paid more attention to economic development and urban expansion during the study period without considering ESH protection. In areas with less human activity, such as mountains and nature reserves, existing studies have shown that ESH always remained at the relatively high level [ 46 , 55 ]. In Zhuhai City, there are many mountains and much forest land distributed in areas such as College Town and DouMen Town with low POPD, which also plays a certain role in ESH protection, indicating that the Zhuhai government carried out related management of the ecosystem of these regions [ 32 ]. The relatively weak and ordinary areas mainly around urban areas or the city center correspond to weak level areas, therefore regions around or adjacent to urban centers need to pay attention to improving and protecting the urban ecosystem when enjoying the economic expansion of the city center.
4.2. Spatial Spillover Effect in the Relationship between ESH and Urbanization
In order to obtain a more complete understanding of the impact of urbanization on ESH, this study measured the spatial relationship between ecosystem health and urbanization. Three indicators GDPD, CAP and POPD were selected as explanatory variables to represent the main characteristics and feature of urban development, while ESH was selected as the dependent variable. In addition, this study investigated the impact of urbanization on ESH from the aspects of population growth, economic development and urban expansion. The results showed that the states of ESH was affected by the urbanization level in its neighbor areas, thus the existence of the spillover effect, which refers to a spatial externality resulting from place-based proximity, i.e., one unit enjoying benefits or incurring costs from its neighbors [ 29 ]. In this study, a decreased level of ESH in some regions may result from an increasing level of urbanization in neighboring areas according to the results in Section 3.3 . A possible reason for this may be that urban areas continue to exchange energy and materials with neighboring areas. Changes of environmental elements (e.g., precipitation, temperature and carbon dioxide) in a given location are likely to propagate to surrounding areas through natural processes such as atmospheric movement and animal migration, of the ability of the surrounding areas to provide ESs [ 46 , 56 ]. This helps us to understand why not only urban centers, but also suburban areas, were observed to have large areas of low ESH and high urbanization.
Bivariate LISA ( Figure 5 ) and the results in Table 3 show that it was not always urbanization that exerted a negative impact on ESH at the local level, indicating that researchers should further explore the relationship between ESH and urbanization because there may exist other factors such as vegetation types and roads that could contribute to changes in ESH.
4.3. Better Management of the Urban Ecological Environment by Incorporating the Spatial Relationship between ESH and Urbanization
Urban planners and environment managers are always faced with the question of how to balance the relationships among urban construction, economic development and ecological conservation. Although some solutions, such as demarcating ecological protection areas, were suggested by many studies, there are some problems. For instance, some research did not consider the influences of urbanization on environmental management. To address this concern, this study provides several recommendations for better environmentally friendly urban planning.
Four types of clustering patterns (i.e., HH, HL, LL and LH) between three variables that measure urbanization, i.e., population, economy and constructed area urbanization and ecosystem health were explored in this study. Urban planners should set areas with high ESH as ecologically friendly land use types. For instance, the urban green space including parks, forests and grass land should be classified as this kind of land use type. Areas with a high urbanization level should be given more attention to protect them from further deterioration Measures such as developing more green spaces or converting constructed areas into urban green space should be adopted. Areas with high ESH and high urbanization should be recognized as “ecological function regions”, in which the environmental friendly land use type could be encouraged. Areas with low ESH and low urbanization should be considered to give priority to building urban green spaces.
5. Conclusions
This study investigated spatiotemporal changes of urban ecosystem health and three indicators of urbanization (GDPD, CAP and POPD) in 1999, 2005, 2009 and 2013 at the urban scale. By combining remote sensing analysis, which derived CAP and ESH data, and statistical data published by the National Science and Technology Infrastructure of China, the study measured the spatial correlations between the three indicators and ESH in order to gain a better understanding of how urban ecosystems can be protected. As urban ecosystems are complex and open, they are very susceptible to the surrounding environment. Thus it is imperative to explicitly consider spatial dependencies between ESH and urbanization to better characterize urban ecosystems. The results of this study support the following conclusions. First, ESH was negatively correlated with all three types of urbanization, i.e., economic, constructed area and population urbanizations when measured for the whole study area. Nonetheless, it was found that there were four distinct kinds of patterns of local correlations according to the bivariate LISA method. The results showed that different management approaches could be developed according to the characteristics of different regions. Second, this study discovered a spillover effect in relationships between ESH and urbanization. The results of this study on the relationship between urban development level and ESH can provide practical guidance for future urban environmental protection and construction.
Acknowledgments
We are grateful for the comments of the anonymous reviewers, which greatly improved the quality of this paper.
Author Contributions
Conceptualization: N.C., L.G. and C.-C.F.; Methodology: N.C. and R.H.; Software: N.C. and R.H.; Formal Analysis: N.C.; Resources: N.C. and L.G.; Data Curation: L.G.; Writing-Original Draft Preparation: N.C.; Writing—Review and Editing: C.-C.F. and L.G.; Project Administration: L.G.; Supervision: L.G.; Funding Acquisition, L.G.
The work presented in this paper was supported by the key research project of the Chinese Ministry of Science (2017YFC0505601) and the innovation team project of the Chinese Nationalities Affairs Commission (10301-0190040129).
Conflicts of Interest
The authors declare no conflict of interest.
Thank you for visiting nature.com. You are using a browser version with limited support for CSS. To obtain the best experience, we recommend you use a more up to date browser (or turn off compatibility mode in Internet Explorer). In the meantime, to ensure continued support, we are displaying the site without styles and JavaScript.
- View all journals
- Explore content
- About the journal
- Publish with us
- Sign up for alerts
- Open access
- Published: 19 August 2022
Evaluating contributions of urbanization and global climate change to urban land surface temperature change: a case study in Lagos, Nigeria
- Liying Guo 1 ,
- Liping Di 1 ,
- Chen Zhang 1 ,
- Fei Chen 2 &
- Alamin Molla 1
Scientific Reports volume 12 , Article number: 14168 ( 2022 ) Cite this article
6412 Accesses
15 Citations
53 Altmetric
Metrics details
- Climate sciences
- Environmental sciences
This study develops a general method to evaluate the contributions of localized urbanization and global climate change to long-term urban land surface temperature (ULST) change. The method is based on the understanding that long-term annual ULST is controlled by three factors: (1) localized urbanization, (2) global climate change, and (3) interannual climate variation. Then the method removes the interannual climate fluctuations on long-term observed LST time series via linear regression and separates the contributions of urbanization and climate change to the impacts on long-term ULST via urban–rural comparison. The method is applied to Lagos, a fast-growing metropolis in the tropical West Africa, as an example for reference. Combined time-series daily daytime and nighttime MODIS Land Surface Temperature (LST) data over the years of 2003–2021 are used as the representation of land surface temperature. To avoid the potentioal interannual data biase due to uneven availability of data in the rainy seasons over years, only MODIS LST data from dry seasons are used in the study. The results are summarized as follows for Lagos: (1) long-term annual ULST is confirmed to be controlled by the three factors; (2) the proposed method can separate the contribution of the three factors to the ULST; (2) both localized urbanization and global warming are verified to contribute to the ULST increase with positive trends; (3) daytime ULST increased the most in the afternoon time at a mean rate of 1.429 °C per decade, with 0.985 °C (10 year) −1 contributed by urbanization and 0.444 °C (10 year) −1 contributed by climate warming; (4) nighttime ULST in Lagos increased the most after midnight at a rate of 0.563 °C (10 year) −1 , with 0.56 °C (10 year) −1 contributed by urbanization and 0.003 °C (10 year) −1 contributed by climate warming; and (5) urbanization is generally responsible for around 60.97% of the urban warming in Lagos. Therefore, the increasing urbaniztion-induced urban heat island effect is the major cause for more heat-related health risks and climate extremes that many urban residents are suffering. The results of this study are of useful reference for both urbanization and climate change related issues in the geo-science field.
Similar content being viewed by others
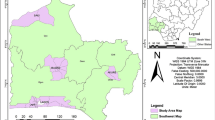
Variations in urban land surface temperature intensity over four cities in different ecological zones
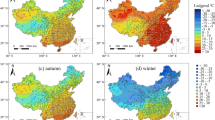
Exploring the relationship between land use/land cover and apparent temperature in China (1996–2020): implications for urban planning
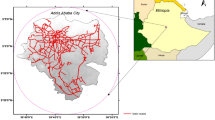
Exploring urban land surface temperature using spatial modelling techniques: a case study of Addis Ababa city, Ethiopia
Introduction.
The rapid urbanization in many developing countries over the past decades seems to have been accompanied by extensive land use and land cover changes, which potentially affect local or regional climate through altering the surface energy and water balances 1 , 2 , 3 , 4 , 5 , 6 . One of the major consequences of these modifications is the increase in land surface temperature (LST) in urban areas, which strengthens the urban heat island (UHI) effect 7 , 8 . The UHI is a localized climate phenomenon whereby urban areas experience warmer temperatures than their surrounding non-urban areas 9 , 10 . One of the major impacts of the UHI is on microclimates, which are affected by the increased atmospheric temperature within and around urban areas. UHI probably is a driver for the increasing frequency and intensity of extreme weather events in metropolises 11 , 12 .
The change of surface temperature in urban areas, especially in fast-growing cities, is influenced by three major factors: long-term global climate change, interannual climate fluctuation, and urbanization. Both global climate warming and urbanization tend to increase the temperature in urban areas, while climate fluctuation has either positive or negative impacts on the surface temperature, depending on a specific year. It has been verified that the increase in urban surface temperature is caused by the overlapping impacts of global climate change and localized microclimate change 13 . To understand the urbanization impacts on ULST, the impacts of global changes and interannual climate variation have to be removed from the long-term LST records. However, it is difficult to separate the effects of localized urbanization and global warming on ULST increase from the long-term LST observation records.
The impact of urbanization on urban thermal environments can be assessed by comparing the difference between the urban and rural LSTs 14 . Considerable studies have been conducted in different parts of the world to estimate the temporal change of remotely-sensed LST to determine the UHI effects due to urbanization 15 , 16 , 17 , 18 , 19 , 20 . The satellite remote sensing data, with its large spatiotemporal coverage, is ideal to be used to observe and compare Earth surface changes 21 . Satellite thermal infrared retrievals with continuous estimates have been used to fill gaps in surface air temperature measurements from weather stations 22 . It is well known that observations from satellite remote sensors can only derive under clear-sky weather conditions 23 . However, tropical regions are characterized by high year-round temperatures and seasonal abundant precipitation. It is hard to monitor long-term year-round LSTs in tropical regions from satellite-observed data. Insufficient observations are still a big challenge in estimating the surface meteorological environment.
This study attempts to overcome the above difficulties and challenges in evaluating the urbanization impacts on the ULST change in the context of the global clime change. Lagos, the largest city of Nigeria located in West Africa 21 , is selected as an example of a tropical city in this study. Lagos’s population had risen from 1.4 million in 1970 to 14.9 million in 2021 ( https://worldpopulationreview.com/ ) and is estimated to surpass 88 million by 2100 24 . To increase the temporal satellite-observed coverages, combined daily diurnal (daytime/nighttime) Terra/Aqua MODIS LST products are used over the past two decades in this study. The main work of this study includes (1) validating daily daytime and nighttime Terra/Aqua LST coverages; (2) generating high temporal coverage LST datasets and group time-series annual LSTs; (3) estimating the systematic LST changes by using linear regressions to remove the interannual fluctuations; (4) quantifying the long-term individual contributions from global climate change and localized urbanization to the ULST; (5) and developing a general method to evaluate the UHI effect in the context of climate change.
Results and discussions
We perform the analysis using the annual dry-season (November, December and January) mean, maximum, and minimum LSTs time series as inputs to estimate the individual contributions of global climate change and localized urbanization to the ULST change.
Urban study and rural reference sites
Lagos metropolis as a case study is located in southwestern Nigeria, on the tropical coast of West Africa 25 (Fig. 1 a). It is currently one of the most populated and one of the fast-growing cities in sub-Saharan Africa 26 . The climate in this region is typically tropical with wet and dry seasons. The wet season is from April to October and the dry season is from November to March 27 . The mean annual rainfall is nearly 2000 mm, the minimum air temperatures range averagely from 20.70 to 22.59 °C, and the maximum temperatures from 28.06 to 30.25 °C. But the high temperatures occur usually within the dry season when the average maximum temperatures range from 31.05 to 33.19 °C 28 . The maximum daytime air temperature can reach above 40 °C during the dry season 29 . The highest UHI effects have been observed happening within the dry season and thus pose a higher heat-based health risk to local residents 30 .
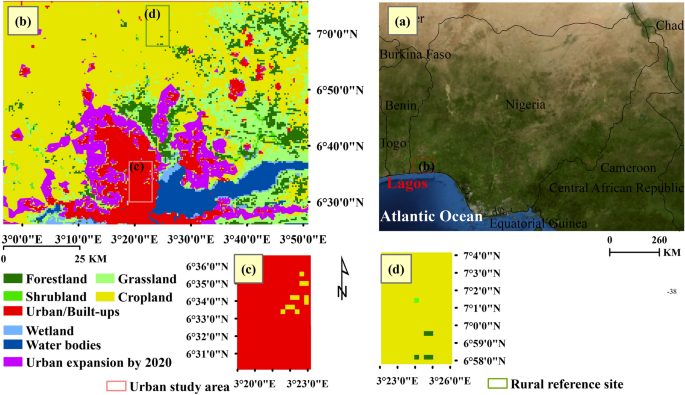
Location of study sites: ( a ) map of Nigeria with Lagos; ( b ) land cover map of Lagos area with both ( c ) urban study area and ( d ) rural reference site based on 2001.
The locations of the urbanized area and its reference site are crucial to the estimations. A large Lagos area (Fig. 1 b) with both urbanized areas and their suroundings at the size of 105 km × 73.5 km is extracted through the Google Earth Engine (GEE). We delineate a rectangular region within the city center of Lagos totaling 100 km 2 as an urban study area (Fig. 1 c), where land is covered by at least 97% impervious surface based on the land use and land cover (LULC) categories in 2001. The reference site (Fig. 1 d) is chosen within a non-urbanized area 30 km away from Lagos city, with the same size and shape as the urban study area. More than 95% of land in the reference site is covered by crop and little land-use conversion took place over the last twenty years. The reference site is selected based on the fact that it is located in a non-urban region with a similar climate zone as urban areas but far away from the impact of urbanization on LST, and its LULC have changed little over the years. In this study, LULC data are retrived from the International Geosphere-Biosphere Programme (IGBP) of MODIS MCD12Q1 product 31 , 32 , which is an annual global land cover classification product at 500-meter resolution, available from 2001 to 2020. The descriptions of IGBP land cover classes can be found in Liang et al. 33 .
The annual variations for ULSTs and RLSTs
Figure 2 depicts the annual fluctuations and their long-term linear trends as well as the corresponding linear regression models of the maximum, mean, and minimum LSTs for the period 2003–2021 over the urban area (left plots) and rural reference area (right plots) in Lagos during four observations at 10:30, 13:30, 22:30, and 01:30 local times. The results show that all LSTs of time series have the similar linear growth patterns over time. It is also found that there are interannual fluctuations in all long-term LSTs, especially in the urban area. Figure 3 illustrates the largest magnitudes of interannual variations, which are obtained by calculating the difference between the highest and lowest annual LSTs during the time period in a specific group. Generally, ULSTs exhibit the largest interannual fluctuation reaching a maximum of 4 °C difference in the daytime. RLSTs are relatively flat with only around 2 °C difference. The results confirm that the long-term ULSTs during the days have higher interannual variations than those during the nights, while no significant difference can be observed both daytime and nighttime in the RLSTs.
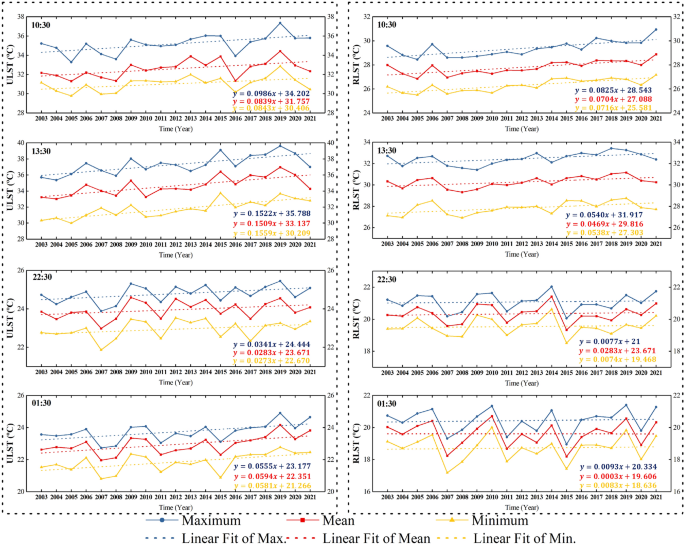
The annual fluctuations and their linear trends with separate linear regression models of the maximum, mean, and minimum LSTs for the period 2003–2021 over the urban area (left plots) and rural area (right plots) in Lagos during four observation times at 10:30, 13:30, 22:30, and 01:30. The blue, red, and yellow solid curves depict respectively the annual variations of the maximum, mean, and minimum LSTs. The straight dot lines represent their linear fits calculated by the linear regression models with the same colors for the max., mean, and min. groups. The equations at the bottom right are their linear regration models, where \(y\) means the fitted value of LST and \(x\) is the progressive time (year).

The difference in annual maximum, mean, and minimum LSTs during four observation times at 10:30, 13:30, 22:30, and 01:30 for the urban area ( a ) and rural area ( b ). The values are obtained by calculating the difference between the highest and lowest annual LSTs during the time period in a specific group.
The temporal trend of LST time series
As introduced below in the " Methods " section, the values of \({\Delta T}_{U}\) and \({\Delta T}_{G}\) can be obtained by calculating fitting values from linear regression models for the urban and reference site to remove the effects of the interannual variation and global climate change from the time series, respectively. Therefore, the specific linear regression models are generated by time-series LST groups as shown in Fig. 2 . The specific fitted LSTs for all time series are generated with the corresponding linear regression models. From the linear fitting lines, it can be observed that all LSTs have evident positive trends. The total accumulative increases for all LSTs are computed separately from the difference of the fitting value between the final year and the initial year as displayed in Fig. 4 . The results further confirm that the warming is noticeable over the time, especially during the daytime. The annual LST in the daytime during the dry season is assessed to exceed 38.68 °C in 2021with an annual maximum warming rate of 0.144 °C. What’s more, urban areas tend to suffer stronger warming than rural areas. During the daytime, the mean ULST has an accumulative warming of 2.113 °C over the 19-year period with an average annual mean warming rate of 0.111 °C. The warming trend is also observed in the rural reference area with 1.056 °C mean accumulative warming. During the nighttime, ULST is with an accumulative warming of 0.789 °C, and RLST is negligible, merely 0.088 °C over the 19-year period.
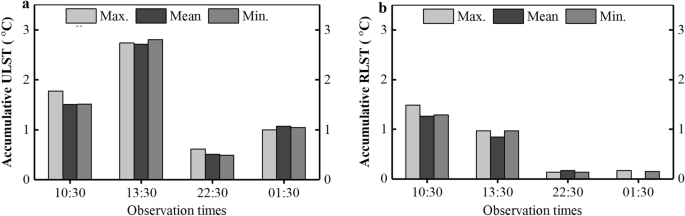
The accumulative maximum, mean, and minimum LSTs during four observation times at 10:30, 13:30, 22:30, and 01:30 for the urban ( a ) and rural area ( b ).
The contribution of localized urbanization and global warming to ULST
Table 1 shows the specific values of decadal change rates of annual mean, maximum and minimum LSTs at four observation times. From Table 1 , it is clear that both urbanization and climate warming contribute to ULSTs increase. During the daytime, the decadal change rates of \({\Delta T}_{U}\) are 0.795 °C, 0.934 °C, and 0.799 °C at 10:30 AM, and 1.430 °C, 1.442 °C, and 1.477 °C at 1:30 PM for the annual mean, maximum and minimum temperatures, respectively. The change rates of \({\Delta T}_{G}\) is 0.709 °C (10 year) −1 at 10:30 AM and around 0.489 °C (10 year) −1 at 1:30 PM. During the nighttime, the decadal change rates of \({\Delta T}_{U}\) have a higher increase in annual temperatures at 1:30 AM (around 0.546 °C) than at 10:30 PM (around 0.283 °C), while the warming rates of \({\Delta T}_{G}\) is around 0.078 °C (10 year) −1 at 10:30 PM and around 0.057 °C (10 year) −1 at 1:30 AM. In general, the annual ULST/RLST changes are all positive during the day and night, and the warming is higher during the day than at night. Comparing values of both \({\Delta T}_{U}\) and \({\Delta T}_{G}\) , it can be found that during the day, global warming contributes more to the ULST increase at 10:30 AM than at 1:30 PM.
Since the urbanization has little impact on the reference region, the \({\Delta T}\) in the rural area is considered to be approximately the \({\Delta T}_{G}\) in the urban area. With this approximation, the contribution of urban expansion to the change of urban LST ( \(\Delta {T}_{UHI}\) ) can be obtained with Eq. ( 2 ). The results, as shown in Table 1 , indicate that during the daytime urbanization contributes significantly more to urban warming in the afternoon than in the morning, and urbanization is also a dominant contributor to urban warming during the nighttime compared with global warming.
Figure 5 depicts the long-term contributions of urbanization (a) and global warming (b) on the surface temperature around at four observation times. No significant difference can be observed among mean, maximum, and minimum LSTs in their contributions. On the whole, it is estimated that urbanization contributes averagely 60.97% of the urban warming. Unlike the urban study area, which is mainly covered by impervious materials, the rural reference site is covered with crops, grasses, shrubs, and trees, which can convert a lot of incoming solar radiation to latent heat through evapotranspiration. Therefore, it’s easy to understand that the urban LST is consequently higher compared to that in the reference site 25 , 26 , 34 . The urban temperature anomaly tends to increase primarily with the changes in city morphology and characteristics of urban surface, such as density and height of buildings, and the materials of both roofing sheets and impervious surface, which also could block more re-radiation from the surface for cooling down at night 13 , 27 . The rural surfaces are comparatively simple and therefore the thermal radiation emitted experiences greater nocturnal radiative flux divergence than complex urban surfaces 35 , 36 . It generally follows why urbanization leads to the rise in temperature of the urban areas, and the stronger UHI effect is observed during the afternoon and night. The increasing urban-induced warming could cause urban residents to be uncomfortable and increase the likelihood of extreme events in the future 37 . Therefore, quantifying the impact of urbanization on UHI is of great value for future researches on how to mitigate the UHI effects and enhance the comfort of tropical dwellers living in cities.
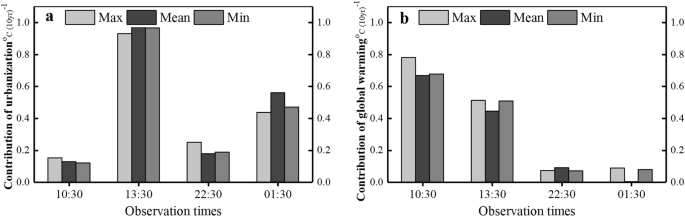
The long-term contributions of urbanization ( a ) and global warming ( b ) at four observation times.
Conclusions
In this study, we developed a general method to quantify the individual contributions of localized urbanization and global warming to ULST increase and applied the method to Lagos as an example. Daily diurnal MODIS LST products from both Terra and Acqua satellites during January, November, and December (dry season) for the period of 2003–2021 are used in the case study. We selected the city center of Lagos as the urban study area and its surrounding non-urban area as the reference site based on the land cover in 2001. The main conclusions are summarized as follows.
Long-term annual urban LST is influenced by the combined effects of localized urbanization, global climate change, and interannual climate variation. The systematic analysis is proposed to quantify and confirm their combined impacts on long-term annual dry-season ULSTs. It is of great reference for monitoring the local climate change in the context of urbanization and global climate change.
The combination of daytime and nighttime Terra/Aqua MODIS LST products is optimal for producing annual observed LST time series for the past two decades. It fills data biases derived from satellite remote sensing data in the tropics.
Linear regressions are established to remove the interannual climate fluctuations on long-term observed LST time series. Analysis indicates that the daytime magnitudes of annual dry-season ULSTs are at the highest interannual fluctuation, reaching beyond a 4 °C difference in maximum temperature and a 3 °C in mean/minimum. The nighttime interannual ULSTs fluctuate from 1.4 to 2.2 °C. In contrast, the interannual fluctuation of RLSTs is generally flat with only around a 2 °C difference.
Urban–rural comparison method has been developed to separate the contributions of urbanization and global climate change to long-term ULST impacts. The location of a reference site is crucial to the estimations. Both localized urbanization and global climate change are verified to contribute to the increase in dry-season ULSTs with positive long-term trends during the day and night. The warming is higher during the day than at night. The annual global climate change during the day is observed to have more contribution in the morning than in the afternoon with a maximum of 0.782 °C per decade around 10:30 AM local time.
Daytime LST in Lagos increased the most in the afternoon at a mean rate of 0.1429 °C per year over the past two decades, with 0.0985 °C per year contributed by urbanization and 0.0444 °C per year contributed by global climate change. Nighttime LST in Lagos increased the most after midnight at a rate of 0.0563 °C per year, with 0.056 °C per year contributed by urbanization and 0.0003 °C per year contributed by global climate change
Generally, urbanization and global climate change contributed averagely 60.97% and 39.03% of the urban warming in Lagos, respectively.
Lagos, a typical large tropical metropolis with rapid urban expansion, is ideal for studying the impacts of urbanization and climate changes on LST change. The increasing urban-induced heat in Lagos could cause urban residents to suffer more heat-based health risks and increase the extreme events. Therefore, quantifying the impact of urbanization on UHI is of great value for future researches on how to mitigate the UHI effect and enhance the comfort of tropical dwellers living in cities.
At the end, we would also like to point out the limitations of results from this study. Although the method we developed in this study is general and location-independent and can be applied to any urban areas in the world, the conclusions about warming degrees, the temperature increase rates, and the contribution percentages by the urbanization and global warming are only relevant to Lagos. This is because urbanization speed, characteristics, urban landscape are different from different urban areas and the magnitude of global warming impacts is location dependent. In the future study, we can apply the method to urban areas at different climate zones with different socioeconomic status to find out how these factors can impact the UHI effects.
Intruduction to methods
The main objective of this study is to develop a general method to estimate the contributions of localized urbanization and global climate change on ULSTs and take Lagos as a case study to apply this method for estimations. It is well known that global warming and urbanization are the two key contributors to the higher LST in cities 38 . In addition, the interannual climate fluctuation also plays a role in the annual LSTs. In order to realize the objectives of this study, we have to separate the contributions of these three factors to the annual LSTs in a city.
It can be reasonably assumed that the annual (mean, min., or maximum) LSTs will fluctuate around a constant value due to interannual climate variations if there is no global warming or urbanization. It is also assumed that both global warming and urbanization contribute linearly to the LST increase in urban areas over the years. Therefore, a linear regression of the annual LST time series will remove the contribution of interannual climate variations to the annual LST, and the linear function obtained from regression represents the long-term systematic trend of the LST for a city area, which represents the combined contributions of both the urban expansion and local impact of global warming. Although the trend of temperature increase due to global warming varies globally, the trends for the two nearby areas within the same climate regime should be similar if not the same. That is why we select a nearby area, which has been neither subjected to urbanization nor experienced a large land use/land cover change, as the reference site for quantifying the contribution of global warming. The time series of annual LSTs and the linear regression are also calculated for the reference site. The linear function obtained from the regression on the reference site represents the trend of LST change due to global warming. The trend from the reference site can be used to approximate the trend of LST change due to global climate change in urban areas. Therefore, it can be assumed that the difference in the linear functions between the urban study area and the rural reference site can be attributed to the contribution of urbanization to ULST change.
Thus, the contributions of both localized urbanization and global warming can be quantified by the following steps: (1) calculating annual time-series LSTs for both the urban study area and the reference site; (2) using linear regressions to remove the interannual variation from the time series of both the study area and the reference site. The linear functions resulting from linear regression represent the long-time trends of LSTs in the study area and the reference site, respectively; (3) For the urban study area, calculating the systematic trend of LST contributed by the combination of urban expansion and local impact of global warming as shown in Eq. ( 1 ); and (4) deriving LST increase contributed by urbanization through removing the contribution of global warming from the systematic trend of LST in the reference site as shown in Eq. ( 2 ).
Based on the discussion above, ( \(\Delta {T}_{U})\) is the combination of the UHI effect caused by urbanization and the local impact of global warming. Thus,
where \(\Delta {T}_{U}\) denotes the overall systematic ULST change from the initial year to the final year, \(\Delta {T}_{UHI}\) means the LST change due to the urbanization effect, and \({\Delta T}_{G}\) is the overall LST change due to global warming. The values of \(\Delta {T}_{UHI}\) thus could be calculated by
Therefore, to derive \(\Delta {T}_{UHI}\) , we have to obtain \({\Delta T}_{G}\) first by finding a region that is not only close enough to the city so that the local impact of global warming is similar by being within the same or similar climatic zone, but also far enough from the city so that its LST is not impacted by the urbanization in the city. This region is called the reference site, which is usually selected from the nearby rural areas. Further, this site and its immediate neighbor should have no significant land use and land cover change (LULCC) over a long period of time. As such, the systematic LST change in this site is most likely to be linked to external atmospheric forcing, i.e., global climate change. The annual LST time series for the reference region then is calculated from the MODIS LST products and the linear regression on the time series is performed to remove the interannual variability. Then, \({\Delta T}_{G}\) can be obtained by
where \({T}_{Rf}\) and \({T}_{Ri}\) are the LSTs of the reference region at the final time and the initial time of the time series, respectively, obtained through the linear regression equation.
Figure 6 provides the graphic description that explains the method in this study. In the figure, the curves represent the time series of annual specific LSTs for the urban study area (red) and the rural reference area (green). The straight dot lines are the minimum mean square error (MMSE) linear fitting obtained through linear regression as the trends of the LST time series for the urban (red) and rural areas (green). \({\Delta T}_{U}\) (in dark) is the systematic LST change during the study period for the urban area, which is the difference between the fitted value of ULST at the final time of the time series and that value at the initial time of the time series. \({\Delta T}_{G}\) (in purple) is the contribution of global climate change to \({\Delta T}_{U}\) . \({\Delta T}_{G}\) is calculated by the temperature difference between the fitted value of LST at the final time and that value at the initial time of the time series for the rural area. \(\Delta {T}_{UHI}\) (in red), the contribution of urbanization to long-term ULST change \(\boxtimes\) , is calculated by the difference between \({\Delta T}_{U}\) and \({\Delta T}_{G}\) .
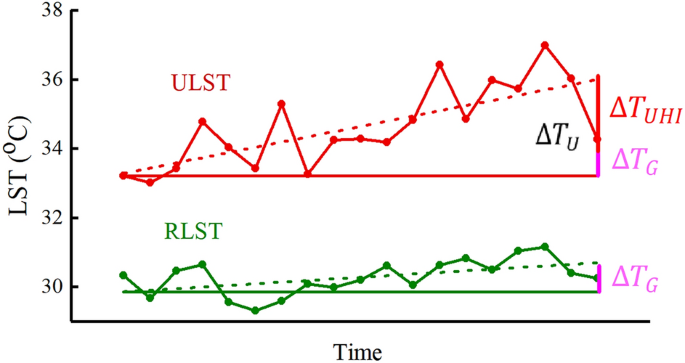
A combined diagrams to depict the methods in this study.
LST data acquistition
One effective method to measure LST is thermal remote sensing. In this study, we use two daily MODIS Land Surface Temperature & Emissivity products, MOD11A1 (version 6) and MYD11A1 (version 6), from the National Aeronautics and Space Administration (NASA). The MOD11A1 daily LST product, available from February 24, 2000, is derived from the MODIS sensor onboard the Terra satellite. And the MYD11A1 daily LST product, available from July 04, 2002, is derived from the MODIS sensor onboard the Aqua satellite. Thereby, the MODIS LSTs are compiled from January 01, 2003 to December 30, 2021. Both Terra and Aqua MODIS products provide daily global coverage ( http://modis.gsfc.nasa.gov ). They have different local overpass times, i.e., Terra descending around 10:30 and ascending around 22:30 at local time, Aqua descending around 01:30 and ascending around 13:30 at local time, which allow the two MODIS sensors to observe the Earth surface four times per day at 01:30, 10:30, 13:30, and 22:30 local time. Clouds and other atmospheric disturbances often obscure parts of or even the entire observation scene, which is a significant obstacle to continuously monitor or predict LST changes, especially in tropical regions 23 . The Terra and Aqua MODIS LST products are only captured under cloud-free conditions 32 . Therefore, data availability of LSTs may influence the accuracy of the accumulated annual LST estimations 23 , 39 .
Google Earth Engine (GEE) platform is used to access daily LST time series, convert LSTs units, and calculate the regional average LSTs for the urban study area and rural reference site. Specifically, the daily daytime and nighttime LSTs (LST_Day_1km and LST_Night_1km) are selected firstly from MOD11A1 and MYD11A1, respectively. Next, the LST values are converted from Kelvin to Celsius units using the following formula:
where \({T}_{c}\) is the temperature in Celsius (°C), \({T}_{k}\) is the scaled absolute temperature in Kelvins (K) stored in the MODIS LST products, and 0.02 is a scale factor. And then an existing GEE function is applied to calculate a single cumulative value of the mean/max./min. LSTs during the study time period. Lastly, the boundary polygons that determined the urban study area and rural reference region are uploaded to clip the corresponding LST values and then export these LSTs to Google Drive.
LST data availability
When deriving meteorological parameters from remote-sensing time series, those satellite observations are expected to be presented in good quality 40 . Due to cloud cover and weather conditions, the remotely sensed LST time-series products, especially in the tropical regions, contain both spatial and temporal gaps and missing values, which could cause undesirable uncertainties in the analysis. To increase the spatial/temporal coverage of LST in the Lagos area, combined daily diurnal MODIS LSTs from both Terra and Aqua satellites are derived in this study to generate the daytime and nighttime LST time series. Because the MYD11A1 product is available from July 2002, which is later than the available date of MOD11A1, the time period of data collection for this study is thus set to the time period from the first day of 2003 to the last day of 2021. Since the entire temporal period covers a total of 6940 days (19 years) as well as four observation times each day, the amount of remotely sensed LST images is huge to be processed and calculated. Therefore, the Google Earth Engine (GEE) ( https://earthengine.google.com/platform/ ), which is a cloud-based platform for a variety of geospatial analyses, is performed to collect and process time-series daily mean, maximum and minimum ULSTs and RLSTs from daytime and nighttime MOD11A1 and MYD11A1 products.
As introduced above, Lagos is located within a tropical climate zone characterized by high year-round temperatures and abundant seasonal precipitation. Due to frequent cloud cover in this region, satellite-observed LST data are not available for each day. Particularly during the wet seasons, LST images are only available for a few days each month. Statistics from LSTs of those few days are hardly representative of the LSTs of the month, which could result in inaccurate LSTs in month or year and further estimations 41 . To verify and validate this issue, we further count the specipic numbers of cloud-free LST images from MODIS at four observation times for each day in the years 2003–2021 for both study sites. Figure 7 depicts the total numbers of LSTs collected at four observation times for each month of the year throughout the study period across the urban area (left plots) and the rural reference site (right plots), respectively. The higher collections can be found in Jannuary, December, and November. Although satellite remote sensing is an excellent data source for monitoring the Earth surface characteristics on a large scale 42 . It is still challenging to retrieve the satellite-observed land surface characteristics in the tropics. Therefore, for this study, we only compile daily time-series LSTs in January, November, and December for the urban and reference sites to calculate the annual dry-season LSTs. For simplicity, we use the annual LSTs to represent the annual dry-season LSTs in this study. The data processing and results acquisition are in accordance with the methods discussed above.
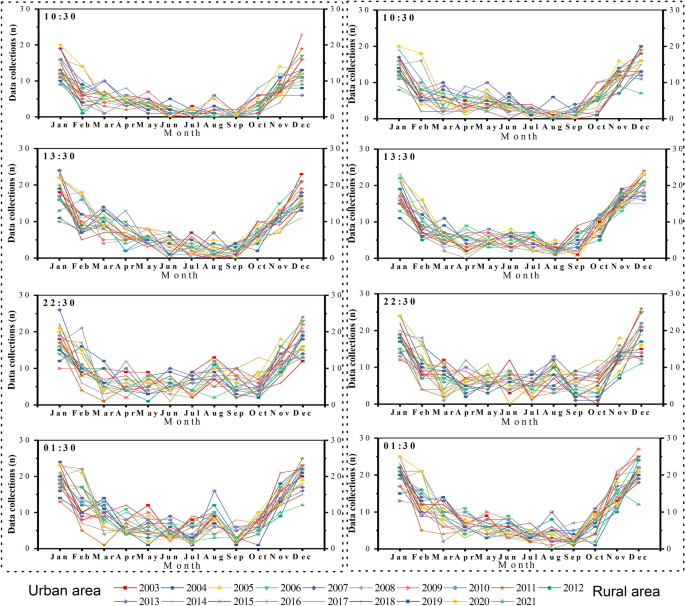
The numbers of data collection by month across the urban area (left plots) and rural reference area (right plots) for the years of 2003 to 2021 at four observation times.
All raw data used in this study, including MOD11A1 (v6), MYD11A1 (v6), and MCD12Q1 (v6), are freely available and online accessible from the following links:
https://developers.google.com/earth-engine/datasets/catalog/MODIS_061_MOD11A1?hl=en .
https://developers.google.com/s/results/earth-engine/datasets?hl=en&q=MYD11A1 .
https://developers.google.com/earth-engine/datasets/catalog/MODIS_006_MCD12Q1?hl=en .
Han, W. et al. The mechanisms and seasonal differences of the impact of aerosols on daytime surface urban heat island effect. Atmos. Chem. Phys. 20 , 6479–6493 (2020).
Article ADS CAS Google Scholar
Pongratz, J. et al. The impact of land cover change on surface energy and water balance in Mato Grosso, Brazil. Earth Interact. 10 , 1–17 (2006).
Article Google Scholar
Yu, M., Liu, Y., Dai, Y. & Yang, A. Impact of urbanization on boundary layer structure in Beijing. Clim. Change 120 , 123–136 (2013).
Article ADS Google Scholar
Guo, L., Di, L. & Tian, Q. Detecting spatio-temporal changes of arable land and construction land in the Beijing-Tianjin corridor during 2000–2015. J. Geogr. Sci. 29 , 702–718 (2019).
Liu, Y., Yan, B. & Zhou, Y. Urbanization, economic growth, and carbon dioxide emissions in China: A panel cointegration and causality analysis. J. Geogr. Sci. 26 , 131–152 (2016).
Guo, L., Di, L., Zhang, C., Lin, L. & Di, Y. Influence of urban expansion on lyme disease risk: A case study in the U.S. I-95 northeastern corridor. Cities 125 , 103633 (2022).
Shahfahad, et al. Longitudinal study of land surface temperature (LST) using mono- and split-window algorithms and its relationship with NDVI and NDBI over selected metro cities of India. Arab. J. Geosci. 13 , 19 (2020).
Dutta, D., Rahman, A., Paul, S. K. & Kundu, A. Changing pattern of urban landscape and its effect on land surface temperature in and around Delhi. Environ. Monit. Assess. 191 , 9 (2019).
Zhao, L., Lee, X., Smith, R. B. & Oleson, K. Strong contributions of local background climate to urban heat islands. Nature 511 , 216–219 (2014).
Article ADS CAS PubMed Google Scholar
Shastri, H., Barik, B., Ghosh, S., Venkataraman, C. & Sadavarte, P. Flip flop of day-night and summer-winter surface urban heat island intensity in India. Sci. Rep. 7 , 1 (2017).
Article CAS Google Scholar
O’Malley, C., Piroozfarb, P. A. E., Farr, E. R. P. & Gates, J. An investigation into minimizing urban heat island (UHI) effects: A UK perspective. Energy Procedia. 62 , 72–80 (2014).
Doan, V. Q. & Kusaka, H. Projections of urban climate in the 2050S in a fast-growing city in Southeast Asia: The Greater Ho Chi Minh City metropolitan area, Vietnam. Int. J. Climatol. 38 , 4155–4171 (2018).
Giridharan, R. & Emmanuel, R. The impact of urban compactness, comfort strategies and energy consumption on tropical urban heat island intensity: A review. Sustain. Cities Soc. 40 , 677–687 (2018).
Shahfahad, et al. Urban heat island dynamics in response to land-use/land-cover change in the coastal city of Mumbai. J. Indian Soc. Remote. 49 (9), 2227–2247 (2021).
Henits, L., Mucsi, L. & Liska, C. M. Monitoring the changes in impervious surface ratio and urban heat island intensity between 1987 and 2011 in Szeged. Hungary. Environ. Monit. Assess. 189 , 5779 (2017).
Google Scholar
Chen, T. Mapping temporal and spatial changes in land use and land surface temperature based on MODIS data. Environ. Res. 196 , 110424 (2021).
Article CAS PubMed Google Scholar
Mohammad, P. & Goswami, A. A spatio-temporal assessment and prediction of surface urban heat island intensity using multiple linear regression techniques over Ahmedabad City, Gujarat. J. Indian Soc. Remote 49 , 1091–1108 (2021).
Sharma, R., Pradhan, L., Kumari, M. & Bhattacharya, P. Assessing urban heat islands and thermal comfort in Noida city using geospatial technology. Urban Clim. 35 , 100751 (2021).
Xing, Z. et al. Estimation of daily mean land surface temperature at global scale using pairs of daytime and nighttime MODIS instantaneous observations. ISPRS J. Photogramm. 178 , 51–67 (2021).
Imhoff, M. L., Zhang, P., Wolfe, R. E. & Bounoua, L. Remote sensing of the urban heat island effect across biomes in the continental USA. Remote Sens. Environ. 114 , 504–513 (2010).
Crowley, M. A. & Cardille, J. A. Remote sensing’s recent and future contributions to landscape ecology. Curr. Landsc. Ecol. Rep. 5 , 45–57 (2020).
Hooker, J., Duveiller, G. & Cescatti, A. A global dataset of air temperature derived from satellite remote sensing and weather stations. Sci. Data. 5 , 246 (2018).
Huang, R. et al. Mapping of daily mean air temperature in agricultural regions using daytime and nighttime land surface temperatures derived from TERRA and AQUA MODIS data. Remote Sens. 7 , 8728–8756 (2015).
Hoornweg, D. & Pope, K. Population predictions for the world’s largest cities in the 21st century. Environ. Urban. 29 , 195–216 (2017).
Ojeh, V., Balogun, A. & Okhimamhe, A. Urban-rural temperature differences in Lagos. Climate. 4 , 29 (2016).
Babalola, O. S. & Akinsanola, A. A. Change detection in land surface temperature and land use land cover over Lagos Metropolis Nigeria. J. Remote Sens. GIS. 5 , 171 (2016).
Adeyeri, O. E., Akinsanola, A. A. & Ishola, K. A. Investigating surface urban heat island characteristics over Abuja, Nigeria: Relationship between land surface temperature and multiple vegetation indices. Remote Sens. Appl. Soc. Environ. 7 , 57–68 (2017).
Ayanlade, A. Variation in diurnal and seasonal urban land surface temperature: Landuse change impacts assessment over Lagos metropolitan city. Model. Earth Syst. Environ. 2 , 1–8 (2016).
Dissanayake, D., Morimoto, T., Murayama, Y., Ranagalage, M. & Handayani, H. H. Impact of urban surface characteristics and socio-economic variables on the spatial variation of land surface temperature in Lagos City, Nigeria. Sustainability 11 , 25 (2019).
Bassett, R., Young, P. J., Blair, G. S., Samreen, F. & Simm, W. The megacity Lagos and three decades of urban heat island growth. J. Appl. Meteorol. Clim. 59 , 2041–2055 (2020).
Zhao, J., Dong, Y., Zhang, M. & Huang, L. Comparison of identifying land cover tempo-spatial changes using globcover and MCD12Q1 global land cover products. Arab. J. Geosci. 13 , 16 (2020).
Sulla-Menashe, D. & Friedl, M. A. User guide to collection 6 MODIS Land cover (MCD12Q1) product. NASA EOSDIS Land Processes DAAC: Sioux Falls, SD, USA, 2018 . (2018).
Liang, D. et al. Evaluation of the consistency of MODIS land cover product (MCD12Q1) based on Chinese 30 M globeland30 datasets: A case study in Anhui Province, China. ISPRS Int. J. Geo-Inf. 4 , 2519–2541 (2015).
Zhou, J., Chen, Y., Wang, J. & Zhan, W. Maximum nighttime urban heat island (UHI) intensity simulation by integrating remotely sensed data and meteorological observations. IEEE J. Stars. 4 , 138–146 (2011).
Hertzberg, M. The night time radiative transport between the earth’s surface, its atmosphere, and free space. Energy Environ. 23 , 819–831 (2012).
Shahmohamadi, P., Che-Ani, A. I., Maulud, K. N. A., Tawil, N. M. & Abdullah, N. A. G. The impact of anthropogenic heat on formation of urban heat island and energy consumption balance. Urban Stud. Res. 2011 , 1–9 (2011).
Liu, Z. et al. Global and regional changes in exposure to extreme heat and the relative contributions of climate and population change. Sci. Rep. 7 , 43909 (2017).
Article ADS CAS PubMed PubMed Central Google Scholar
Shin, J., Kang, M. & Kim, K. R. Outdoor thermal stress changes in south korea: increasing inter-annual variability induced by different trends of heat and cold stresses. Sci. Total Environ. 805 , 150132 (2022).
Simó, G. et al. Landsat and local land surface temperatures in a heterogeneous terrain compared to MODIS values. Remote Sens. 8 , 849 (2016).
Lin, L. et al. Validation and refinement of cropland data layer using a spatial-temporal decision tree algorithm. Sci. Data. 9 , 1169 (2022).
Funk, C. et al. Exploring trends in wet-season precipitation and drought indices in wet, humid and dry regions. Environ. Res. Lett. 14 , 115002 (2019).
Lin, L. et al. Building near-real-time MODIS data fusion workflow to support agricultural decision-making applications. IEEE 2019 , 1–5 (2019).
Download references
Acknowledgements
This research is supported by a grant from NASA Inter-disciplinary Studies Program (Grant# 80NSSC20K1262). The authors would like to thank Ms. Julia Di of Standford University for proofreading the manuscript.
Author information
Authors and affiliations.
Center for Spatial Information Science and Systems, George Mason University, Fairfax, VA, 22030, USA
Liying Guo, Liping Di, Chen Zhang, Li Lin & Alamin Molla
National Center for Atmospheric Research, Boulder, CO, 80301, USA
You can also search for this author in PubMed Google Scholar
Contributions
L.D. designed the study. L.G. and L.D. analyzed and developed the first draft. C.Z. and L.L. processed MODIS data of time series. And all authors reviewed and improved the manuscript.
Corresponding author
Correspondence to Liping Di .
Ethics declarations
Competing interests.
The authors declare no competing interests.
Additional information
Publisher's note.
Springer Nature remains neutral with regard to jurisdictional claims in published maps and institutional affiliations.
Rights and permissions
Open Access This article is licensed under a Creative Commons Attribution 4.0 International License, which permits use, sharing, adaptation, distribution and reproduction in any medium or format, as long as you give appropriate credit to the original author(s) and the source, provide a link to the Creative Commons licence, and indicate if changes were made. The images or other third party material in this article are included in the article's Creative Commons licence, unless indicated otherwise in a credit line to the material. If material is not included in the article's Creative Commons licence and your intended use is not permitted by statutory regulation or exceeds the permitted use, you will need to obtain permission directly from the copyright holder. To view a copy of this licence, visit http://creativecommons.org/licenses/by/4.0/ .
Reprints and permissions
About this article
Cite this article.
Guo, L., Di, L., Zhang, C. et al. Evaluating contributions of urbanization and global climate change to urban land surface temperature change: a case study in Lagos, Nigeria. Sci Rep 12 , 14168 (2022). https://doi.org/10.1038/s41598-022-18193-w
Download citation
Received : 12 April 2022
Accepted : 08 August 2022
Published : 19 August 2022
DOI : https://doi.org/10.1038/s41598-022-18193-w
Share this article
Anyone you share the following link with will be able to read this content:
Sorry, a shareable link is not currently available for this article.
Provided by the Springer Nature SharedIt content-sharing initiative
This article is cited by
Urbanization and regional climate change-linked warming of indian cities.
- Soumya Satyakanta Sethi
Nature Cities (2024)
Using urban pasts to speak to urban presents in the Anthropocene
- Patrick Roberts
- W. Christopher Carleton
- Jürgen Renn
Interactions of urbanisation, climate variability, and infectious disease dynamics: insights from the Coimbatore district of Tamil Nadu
- Sudha Suresh
- Gowhar Meraj
Environmental Monitoring and Assessment (2023)
By submitting a comment you agree to abide by our Terms and Community Guidelines . If you find something abusive or that does not comply with our terms or guidelines please flag it as inappropriate.
Quick links
- Explore articles by subject
- Guide to authors
- Editorial policies
Sign up for the Nature Briefing: Anthropocene newsletter — what matters in anthropocene research, free to your inbox weekly.


An official website of the United States government
The .gov means it’s official. Federal government websites often end in .gov or .mil. Before sharing sensitive information, make sure you’re on a federal government site.
The site is secure. The https:// ensures that you are connecting to the official website and that any information you provide is encrypted and transmitted securely.
- Publications
- Account settings
- My Bibliography
- Collections
- Citation manager
Save citation to file
Email citation, add to collections.
- Create a new collection
- Add to an existing collection
Add to My Bibliography
Your saved search, create a file for external citation management software, your rss feed.
- Search in PubMed
- Search in NLM Catalog
- Add to Search
Impact of Urbanization on Ecosystem Health: A Case Study in Zhuhai, China
Affiliations.
- 1 School of Life and Environmental Science, Minzu University of China, Beijing 100081, China.
- 2 Centre for Spatial Analysis and Policy, School of Geography, University of Leeds; Leeds LS2 9JT, UKphy, University of Leeds.
- 3 Department of Geography, National University of Singapore, Singapore 117570, Singapore.
- PMID: 31779268
- PMCID: PMC6926934
- DOI: 10.3390/ijerph16234717
The past decades have witnessed rapid urbanization around the world. This is particularly evident in Zhuhai City, given its status as one of the earliest special economic zones in China. After experiencing rapid urbanization for decades, the level of ecosystem health (ESH) in Zhuhai City has become a focus of attention. Assessments of urban ESH and spatial correlations between urbanization and ESH not only reveal the states of urban ecosystems and the extent to which urbanization affected these ecosystems, but also provide new insights into sustainable eco-environmental planning and resource management. In this study, we assessed the ESH of Zhuhai City using a selected set of natural, social and economic indicators. The data used include Landsat Thematic Mapper images and socio-economic data of 1999, 2005, 2009 and 2013. The results showed that the overall ESH value and ecosystem service function have been on the decline while Zhuhai City has continued to become more urbanized. The total ESH health level trended downward and the area ratio of weak and relatively weak health level increased significantly, while the areas of well and relatively well healthy state decreased since 1999. The spatial correlation analysis shows a distinct negative correlation between urbanization and ESH. The degree of negative correlation shows an upward trend with the processes of urban sprawl. The analysis results reveal the impact of urbanization on urban ESH and provide useful information for planners and environment managers to take measures to improve the health conditions of urban ecosystems.
Keywords: comprehensive indicators; ecosystem health; remote sensing images; spatial correlation; urbanization.
PubMed Disclaimer
Conflict of interest statement
The authors declare no conflict of interest.
Location of Zhuhai City.
Assessment of spatial correlation between…
Assessment of spatial correlation between urbanization and ecosystem health (ESH). GDPD, density of…
With different ecosystem health levels…
With different ecosystem health levels in 1999, 2005, 2009 and 2013.
Spatial patterns of urban ecosystem…
Spatial patterns of urban ecosystem health in 1999, 2005, 2009 and 2013.
Urbanization levels in Zhuhai City.…
Urbanization levels in Zhuhai City. GDPD, GDP density; CAP, constructed area proportion; POPD,…
Local indicators of spatial association…
Local indicators of spatial association (LISA) cluster maps between ESH and individual urbanization…
Similar articles
- Impact of Fast Urbanization on Ecosystem Health in Mountainous Regions of Southwest China. Xiao Y, Guo L, Sang W. Xiao Y, et al. Int J Environ Res Public Health. 2020 Jan 28;17(3):826. doi: 10.3390/ijerph17030826. Int J Environ Res Public Health. 2020. PMID: 32013019 Free PMC article.
- Quantitative spatial assessment of the impact of urban growth on the landscape network of Türkiye's coastal cities. Yılmaz M, Terzi F. Yılmaz M, et al. Environ Monit Assess. 2023 Mar 14;195(4):466. doi: 10.1007/s10661-023-11084-1. Environ Monit Assess. 2023. PMID: 36914859 Review.
- A Review of Ecosystem Services Research Focusing on China against the Background of Urbanization. Fan Q, Yang X, Zhang C. Fan Q, et al. Int J Environ Res Public Health. 2022 Jul 6;19(14):8271. doi: 10.3390/ijerph19148271. Int J Environ Res Public Health. 2022. PMID: 35886123 Free PMC article. Review.
- Urbanization's Impacts on Ecosystem Health Dynamics in the Beijing-Tianjin-Hebei Region, China. Wu F, Wang X, Ren Y. Wu F, et al. Int J Environ Res Public Health. 2021 Jan 21;18(3):918. doi: 10.3390/ijerph18030918. Int J Environ Res Public Health. 2021. PMID: 33494446 Free PMC article.
- The Impact of Urbanization on Ecosystem Health in Typical Karst Areas: A Case Study of Liupanshui City, China. Zhao Y, Han R, Cui N, Yang J, Guo L. Zhao Y, et al. Int J Environ Res Public Health. 2020 Dec 24;18(1):93. doi: 10.3390/ijerph18010093. Int J Environ Res Public Health. 2020. PMID: 33374404 Free PMC article.
- Assessment of urban ecosystem health and its influencing factors: a case study of Zibo City, China. Wang X, Dong Q. Wang X, et al. Sci Rep. 2024 Apr 11;14(1):8455. doi: 10.1038/s41598-024-59103-6. Sci Rep. 2024. PMID: 38605157 Free PMC article.
- Co-Creation Hub Is the First Step for the Successful Creation of a Unified Urban Ecosystem-Kaunas City Example. Feiferytė-Skirienė A, Draudvilienė L, Stasiškienė Ž, Sosunkevič S, Pamakštys K, Daniusevičiūtė-Brazaitė L, Gurauskienė I. Feiferytė-Skirienė A, et al. Int J Environ Res Public Health. 2022 Feb 24;19(5):2609. doi: 10.3390/ijerph19052609. Int J Environ Res Public Health. 2022. PMID: 35270302 Free PMC article.
- Assessment of Ecosystem Health and Its Key Determinants in the Middle Reaches of the Yangtze River Urban Agglomeration, China. Ge F, Tang G, Zhong M, Zhang Y, Xiao J, Li J, Ge F. Ge F, et al. Int J Environ Res Public Health. 2022 Jan 11;19(2):771. doi: 10.3390/ijerph19020771. Int J Environ Res Public Health. 2022. PMID: 35055591 Free PMC article.
- Li Y., Li Y., Zhou Y., Shi Y., Zhu X. Investigation of a coupling model of coordination between urbanization and the environment. J. Environ. Manag. 2012;98:127–133. doi: 10.1016/j.jenvman.2011.12.025. - DOI - PubMed
- Gu C., Hu L., Cook I.G. China’s urbanization in 1949–2015: Processes and driving forces. Chin. Geogr. Sci. 2017;27:847–859. doi: 10.1007/s11769-017-0911-9. - DOI
- Cao H., Liu J., Fu C., Zhang W., Wang G., Yang G., Luo L. Urban Expansion and Its Impact on the Land Use Pattern in Xishuangbanna since the Reform and Opening up of China. Remote Sens. 2017;9:137. doi: 10.3390/rs9020137. - DOI
- Lv J., Yang B.D., Yang Y.J., Zhang Z.H., Chen F., Liu G.J. Spatial Patterns of China’s Major Cities and Their Evolution Mechanisms during the Past Decades of Reform and Opening Up. Procedia Eng. 2017;198:915–925. doi: 10.1016/j.proeng.2017.07.137. - DOI
- Weng Q. A remote sensing? GIS evaluation of urban expansion and its impact on surface temperature in the Zhujiang Delta, China. Int. J. Remote Sens. 2001;22:1999–2014. doi: 10.1080/713860788. - DOI
Publication types
- Search in MeSH
Related information
Linkout - more resources, full text sources.
- Europe PubMed Central
- PubMed Central
- MedlinePlus Health Information
Miscellaneous
- NCI CPTAC Assay Portal
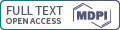
- Citation Manager
NCBI Literature Resources
MeSH PMC Bookshelf Disclaimer
The PubMed wordmark and PubMed logo are registered trademarks of the U.S. Department of Health and Human Services (HHS). Unauthorized use of these marks is strictly prohibited.
Urban economic development in Africa: A case study of Nairobi city
Subscribe to africa in focus, jacob nato , jn jacob nato policy analyst - kenya institute for public policy research and analysis @jacobnato1984 humphrey njogu , hn humphrey njogu principal policy analyst - kenya institute for public policy research and analysis @humnjogu rose ngugi , rn rose ngugi executive director - kenya institute for public policy research and analysis aloysius uche ordu , and aloysius uche ordu director - africa growth initiative , senior fellow - global economy and development , africa growth initiative @aloysiusordu ede ijjasz-vasquez ede ijjasz-vasquez nonresident senior fellow - global economy and development , africa growth initiative @ede_wbg.
February 17, 2023
Below is a viewpoint from the Foresight Africa 2023 report, which explores top priorities for the region in the coming year. Read the full chapter on Africa’s cities .

In most countries, urbanization leads to substantial productivity gains supported by scale, density, and agglomeration. Better connected people and firms lead to savings in transport and logistics, technological and information spillovers, and more efficient labor markets. However, Africa’s urbanization has not realized the full potential and benefits of such agglomeration. The economic transformation and benefits of urbanization, observed in other regions, are yet to be achieved in sub-Saharan Africa.
To understand the barriers, and unlock the economic opportunities of urbanization, the Africa Growth Initiative (AGI) at the Brookings Institution developed an “ Urban Economic Growth Framework for African cities .” The framework focuses on the three primary constraints limiting a city’s ability to benefit from agglomeration and generate productive jobs: Accessibility, the business environment, and public sector governance. The framework provides specific indicators and ways to identify these three critical constraints, with a view to inform and guide policymakers on specific actions and appropriate policies.
As a start, the AGI framework was applied to the city of Nairobi (Kenya’s capital), to analyze Nairobi’s key challenges and possible solutions for growth and employment.
Unemployment and underemployment in Nairobi are a top concern, especially as youth makeup 48 percent of the total unemployed workforce (15 to 64 years). While the labor force in Kenya has been growing at an average annual rate of about 3 percent, Nairobi needs to generate many more (and better) jobs to offer improved livelihood opportunities to its large youth demographic. At the national level, Kenya has registered good progress in creating jobs, especially in the digital and gig economy. The report recommends two areas of focus. First, in coordination with the national government, Nairobi City County needs to support the gradual formalization of the large number of informal jobs and enterprises by easing business registration and motivating registration through targeted support programs. Second, better education and skills in targeted economic sectors are required to enhance productivity and earnings. Nairobi city should ensure that tertiary institutions provide training and skills consistent with emerging technologies.
[Nairobi] city has enormous potential to achieve the benefits of urban agglomeration and create productive jobs by paying particular attention to its challenges in accessibility and infrastructure, business environment, as well as public sector governance and finance.
Furthermore, enterprise data in Nairobi shows that businesses are likely to transition from micro- to medium-, and to large enterprises as the owners’ levels of education attainment rises.
Accessibility within the city: Accessibility is vital for connecting workers to firms and firms to markets. Despite the excellent progress made on infrastructure development, there is a high concentration of unpaved roads in Nairobi’s high-density informal settlements.
Consequently, as shown in the report, most jobs are not accessible within one hour of public transport commute i.e., commuting time by bus, matatu (shared taxi), or foot. The city also has a mismatch in zoning and land use. Nairobi therefore needs a new approach to urban planning that considers population growth, infrastructure, housing, and land use. Equally important is updating the land appraisal system and creating more public spaces.
Business environment: Many businesses in the city face several challenges, including complex processes to access licenses and permits, insufficient finance, expensive land, rigid labor regulations, inefficiency in tax administration, and crime risk. For example, a business takes about 92 days to secure an electricity connection. A firm loses about KSh 2.3 million per year due to power outages on average. These are critical areas for Nairobi to enhance its business environment. Furthermore, it is essential to coordinate the implementation of business policy reforms between the national and county governments.
Public sector governance and finances: The devolution process in Kenya has given Nairobi City County a total of 14 constitutional functions. The city faces important challenges in terms of financing, despite the commendable increase in revenues and fiscal transfers from KSh 9.51 billion in FY 2013/14 to KSh 19.42 billion in FY 2020/21. Still, the city faces several financing shortfalls, from high levels of pending bills and fiscal deficits, to delays in receipt of equitable fiscal transfers. These challenges call for proper budget planning, improved budget execution, and higher levels of the city’s source revenue.
The application of the AGI Urban Economic Growth Framework to Nairobi City County shows that the city has enormous potential to achieve the benefits of urban agglomeration and create productive jobs by paying particular attention to its challenges in accessibility and infrastructure, business environment, as well as public sector governance and finance.
Related Content
James Gachanja, John Karanja, Jacob Nato, Rose Ngugi, Humphrey Njogu, Brian Nyaware, Charity Mbaka, Shadrack Mwatu, Melap Sitati
February 16, 2023
Babajide Olusola Sanwo-Olu, Peter Anyang’ Nyong’o
January 27, 2023
John Page, Jeffrey Gutman, Payce Madden, Dhruv Gandhi
September 29, 2020
Emerging Markets & Developing Economies
Global Economy and Development
Kenya Sub-Saharan Africa
Africa Growth Initiative
Afua Osei, Landry Signé
June 26, 2024
Zouera Youssoufou, Landry Signé
June 12, 2024
Online only
10:00 am - 11:30 am EDT
- Letter to the Editor
- Open access
- Published: 02 January 2020

Urbanization: a problem for the rich and the poor?
- Md Abdul Kuddus 1 , 2 , 4 ,
- Elizabeth Tynan 3 &
- Emma McBryde 1 , 2
Public Health Reviews volume 41 , Article number: 1 ( 2020 ) Cite this article
351k Accesses
171 Citations
31 Altmetric
Metrics details
Urbanization has long been associated with human development and progress, but recent studies have shown that urban settings can also lead to significant inequalities and health problems. This paper is concerned with the adverse impact of urbanization on both developed and developing nations and both wealthy and poor populations within those nations, addressing issues associated with public health problems in urban areas. The discussion in this paper will be of interest to policy makers. The paper advocates policies that improve the socio-economic conditions of the urban poor and promote their better health. Further, this discussion encourages wealthy people and nations to become better informed about the challenges that may arise when urbanization occurs in their regions without the required social supports and infrastructure.
Urbanization refers to the mass movement of populations from rural to urban settings and the consequent physical changes to urban settings. In 2019, the United Nations estimated that more than half the world’s population (4.2 billion people) now live in urban area and by 2041, this figure will increase to 6 billion people [ 1 ].
Cities are known to play multifaceted functions in all societies. They are the heart of technological development and economic growth of many nations, while at the same time serving as a breeding ground for poverty, inequality, environmental hazards, and communicable diseases [ 2 ]. When large numbers of people congregate in cities, many problems result, particularly for the poor. For example, many rural migrants who settle in an urban slum area bring their families and their domesticated animals—both pets and livestock—with them. This influx of humans and animals leads to vulnerability of all migrants to circulating communicable diseases and the potential to establish an urban transmission cycle. Further, most urban poor live in slums that are unregulated, have congested conditions, are overcrowded, are positioned near open sewers, and restricted to geographically dangerous areas such as hillsides, riverbanks, and water basins subject to landslides, flooding, or industrial hazards. All of these factors lead to the spread of communicable and non-communicable diseases, pollution, poor nutrition, road traffic, and so on [ 3 , 4 , 5 ]. The problems faced by the poor spill over to other city dwellers. As the trend to urbanization continues, this spillover effect increases and takes on a global dimension as more and more of the world’s populations are affected [ 3 ].
Some of the major health problems resulting from urbanization include poor nutrition, pollution-related health conditions and communicable diseases, poor sanitation and housing conditions, and related health conditions. These have direct impacts on individual quality of life, while straining public health systems and resources [ 6 ].
Urbanization has a major negative impact on the nutritional health of poor populations. Because they have limited financial resources and the cost of food is higher in cities, the urban poor lack nutritious diets and this leads to illness, which contributes to loss of appetite and poor absorption of nutrients among those affected. Furthermore, environmental contamination also contributes to undernutrition; street food is often prepared in unhygienic conditions, leading to outbreaks of food-borne illnesses (e.g., botulism, salmonellosis, and shigellosis) [ 6 ]. Urban dwellers also suffer from overnutrition and obesity, a growing global public health problem. Obesity and other lifestyle conditions contribute to chronic diseases (such as cancers, diabetes, and heart diseases). Although obesity is most common among the wealthy, international agencies have noted the emergence of increased weight among the middle class and poor in recent years [ 7 ].
Populations in poor nations that suffer from protein-energy malnutrition [ 8 ] have increased susceptibility to infection [ 9 ] through the impact of micronutrient deficiency on immune system development and function [ 10 ]. Around 168 million children under 5 are estimated to be malnourished and 76% of these children live in Asia [ 11 ]. At the same time, the World Health Organization is concerned that there is an emerging pandemic of obesity in poor countries that leads to non-communicable diseases such as diabetes, cardiovascular disease, cancer, hypertension, and stroke [ 12 ].
Obesity is caused by increased caloric intake and decreased physical activity [ 13 ], something historically associated with wealth. However, people in urbanized areas of developing countries are also now vulnerable to obesity due to lack of physical space, continually sitting in workplaces, and excessive energy intake and low energy expenditure. In these areas, infrastructure is often lacking, including sufficient space for recreational activities. Further, in developing countries, as in developed countries, large employers frequently place head offices in urban capitals and work is increasingly sedentary in nature [ 14 ]. Another culprit associated with the risk of developing obesity is the change in food intake that has led to the so-called nutrition transition (increased the consumption of animal-source foods, sugar, fats and oils, refined grains, and processed foods) in urban areas. For instance, in China, dietary patterns have changed concomitantly with urbanization in the past 30 years, leading to increased obesity [ 15 ]. In 2003, the World Health Organization estimated that more than 300 million adults were affected, the majority in developed and highly urbanized countries [ 16 ]. Since then, the prevalence of obesity has increased. For example, in Australia, around 28% of adults were obese in 2014–2015 [ 17 ].
Pollution is another major contributor to poor health in urban environments. For instance, the World Health Organization estimated that 6.5 million people died (11.6% of all global deaths) as a consequence of indoor and outdoor air pollution and nearly 90% of air-pollution-related deaths occurred in low- and middle-income countries [ 18 ]. Poor nutrition and pollution both contribute to a third major challenge for urban populations: communicable diseases. The poor live in congested conditions, near open sewers and stagnant water, and are therefore constantly exposed to unhealthy waste [ 6 ]. Inadequate sanitation can lead to the transmission of helminths and other intestinal parasites. Pollution (e.g., from CO 2 emission) from congested urban areas contributes to localized and global climate change and direct health problems, such as respiratory illnesses, cardiovascular diseases, and cancer for both the rich and the poor.
In addition to human-to-human transmission, animals and insects serve as efficient vectors for diseases within urban settings and do not discriminate between the rich and poor. The prevalence and impact of communicable diseases in urban settings, such as tuberculosis (TB), malaria, cholera, dengue, and others, is well established and of global concern.
National and international researchers and policy makers have explored various strategies to address such problems, yet the problems remain. For example, research on solutions for megacities has been ongoing since the early 1990s [ 19 , 20 ]. These studies have concluded that pollution, unreliable electricity, and non-functioning infrastructure are priority initiatives; nevertheless, air pollution, quality of water in cities, congestion, disaster management issues, and infrastructure are not being systematically addressed [ 19 , 20 ].
The impact of inner city transportation on health, such as road traffic, is emerging as a serious problem. Statistics show that a minimum of 10 people die every day on the railways in the city of Mumbai, India [ 21 ]. Vietnam is another example of a country that has seen a remarkable increase in road traffic accidents [ 22 ]. Improvements to the country’s infrastructure have not been able to meet the increasing growth of vehicular and human traffic on the street. Vietnam reportedly has a population of 95 million and more than 18 million motorbikes on its roads. A deliberate policy is needed to reduce accidents [ 21 ].
Although urbanization has become an irreversible phenomenon, some have argued that to resolve the problems of the city, we must tackle the root causes of the problem, such as improving the socio-economic situation of the urban poor.
Until the conditions in rural areas improve, populations will continue to migrate to urban settings. Given the challenges that rural development poses, the root causes are unlikely to be addressed in the near future. Therefore, governments and development agencies should concentrate on adapting to the challenges of urbanization, while seeking to reduce unplanned urbanization.
Some examples of policies and practices that should be considered include (i) policies that consider whole-of-life journeys, incorporating accessible employment, community participation, mobility/migration and social transition, to break generational poverty cycles; (ii) policies addressing urban environmental issues, such as planned urban space and taxes on the use of vehicles to reduce use or to encourage vehicles that use less fuel as well as encourage bicycle use, walking, and other forms of human transportation; (iii) greater cooperative planning between rural and urban regions to improve food security (e.g., subsidies for farmers providing locally produced, unprocessed and low cost food to urban centers); (iv) social protection and universal health coverage to reduce wealth disparity among urban dwellers; including introduction of programs and services for health, for example by establishing primary healthcare clinics accessible and affordable for all including those living in urban slums [ 23 ].
Availability of data and materials
Not applicable
United Nations. World urbanization prospects. New York; 2019.
McMichael AJ. The urban environment and health in a world of increasing globalization: issues for developing countries. Bulletin of the World Health Organization. 2000;78(9):1117-26.
Alirol E, Getaz L, Stoll B, Chappuis F, Loutan L. Urbanisation and infectious diseases in a globalised world. The Lancet infectious diseases. 2011;11(2):131-41.
Harpham T, Stephens C. Urbanization and health in developing countries. World health statistics quarterly Rapport trimestriel de statistiques sanitaires mondiales. 1991;44(2):62-9.
Moore M, Gould P, Keary BS. Global urbanization and impact on health. Int J hygiene and environmental health. 2003;206(4-5):269-78.
Kennedy G. Food security in the context of urban sub-Saharan Africa. Internet paper for food security, food Africa, internet forum 31 March–11 April 2003;2009.
World Health Organization. Obesity: preventing and managing the global epidemic: World Health Organization; 2000. Available from https://apps.who.int/iris/handle/10665/42330 . Accessed 4 Aug 2019.
Nour NN. Obesity in resource-poor nations. Reviews in obstetrics and gynecology. 2010;3(4):180-4.
Tomkins A, Watson F. Malnutrition and infection: a review. United Nations Administrative Committee on Coordination/Subcommittee on Nutrition. Nutrition Policy Discussion Paper. 1989(5): p. 1-107.
Schaible UE, Stefan H. Malnutrition and infection: complex mechanisms and global impacts. PLoS medicine. 2007;4(5):e115.
Ahmed F, Zareen M, Khan MR, Banu CP, Haq MN, Jackson AA. Dietary pattern, nutrient intake and growth of adolescent school girls in urban Bangladesh. Public health nutrition. 1998;1(2):83-92.
World Health Organization. Obesity and Overweight. World Health Organization, Geneva (Fact sheet no 311); 2006.
Bleich SN, Cutler D, Murray C, Adams A. Why is the developed world obese? Annual review of public health. 2008;29(1):273-95.
Arundell L, Sudholz B, Teychenne M, Salmon J, Hayward B, Healy G, et al. The impact of activity based working (ABW) on workplace activity, eating behaviours, productivity, and satisfaction. International journal of environmental research and public health. 2018;15(5):1005.
Zhang J, Wang D, Eldridge A, Huang F, Ouyang Y, Wang H, et al. Urban–rural disparities in energy intake and contribution of fat and animal source foods in Chinese children aged 4–17 years. Nutrients. 2017;9(5):526.
World Health Organization. Obesity and Overweight. Geveva: World Health Organization; 2003.
Australian Institute of Health and Welfare. Risk factors to health. Australia: Australian Institute of Health and Welfare (AIHW); 2017.
World Health Organization. World Health Organization releases country estimates on air pollution exposure and health impact. Geneva: World Health Organization; 2016.
Mavropoulos A, ISWA S, SA CE, editors. Megacities sustainable development and waste management in the 21st century. World Congress; 2010.
Fuchs RJ, Brennan E, Lo F-C, Uitto JI, Chamie J. Mega-city Growth and the Future: United Nations University Press; 1994.
Doytsher Y, Kelly P, Khouri R, McLAREN R, Potsiou C. Rapid urbanization and mega cities: The need for spatial information management. Research study by FIG commission. 2010;3.
Ngo AD, Rao C, Hoa NP, Hoy DG, Trang KTQ, Hill PS. Road traffic related mortality in Vietnam: evidence for policy from a national sample mortality surveillance system. BMC public health. 2012;12(1):561.
Abebe T. Young people: Participation and sustainable development in an urbanizing world: Un-Habitat; 2012.
Download references
Acknowledgements
The authors would like to thank the editor for his/her thoughtful comments and efforts towards improving the manuscript.
This work was conducted as a part of a PhD programme of the first authors and funded by the College of Medicine and Dentistry at the James Cook University, Australia (JCU-QLD-933347).
Author information
Authors and affiliations.
Australian Institute of Tropical Health and Medicine, James Cook University, Townsville, Australia
Md Abdul Kuddus & Emma McBryde
College of Medicine and Dentistry, James Cook University, Townsville, Australia
Graduate Research School, James Cook University, Townsville, Australia
Elizabeth Tynan
Department of Mathematics, University of Rajshahi, -6205, Rajshahi, Bangladesh
Md Abdul Kuddus
You can also search for this author in PubMed Google Scholar
Contributions
MAK planned the study, analyzed, and prepared the manuscript. ET and EM helped in the preparation of the manuscript. All authors read and approved the final manuscript.
Authors’ information
Corresponding author.
Correspondence to Md Abdul Kuddus .
Ethics declarations
Ethics approval and consent to participate, consent for publication, competing interests.
The authors declare that they have no competing interests.
Additional information
Publisher’s note.
Springer Nature remains neutral with regard to jurisdictional claims in published maps and institutional affiliations.
Rights and permissions
Open Access This article is distributed under the terms of the Creative Commons Attribution 4.0 International License ( http://creativecommons.org/licenses/by/4.0/ ), which permits unrestricted use, distribution, and reproduction in any medium, provided you give appropriate credit to the original author(s) and the source, provide a link to the Creative Commons license, and indicate if changes were made. The Creative Commons Public Domain Dedication waiver ( http://creativecommons.org/publicdomain/zero/1.0/ ) applies to the data made available in this article, unless otherwise stated.
Reprints and permissions
About this article
Cite this article.
Kuddus, M.A., Tynan, E. & McBryde, E. Urbanization: a problem for the rich and the poor?. Public Health Rev 41 , 1 (2020). https://doi.org/10.1186/s40985-019-0116-0
Download citation
Received : 02 September 2019
Accepted : 09 December 2019
Published : 02 January 2020
DOI : https://doi.org/10.1186/s40985-019-0116-0
Share this article
Anyone you share the following link with will be able to read this content:
Sorry, a shareable link is not currently available for this article.
Provided by the Springer Nature SharedIt content-sharing initiative
- Urbanization
- Public health
Public Health Reviews
ISSN: 2107-6952
- Submission enquiries: Access here and click Contact Us
- General enquiries: [email protected]
Impact of road connectivity on urbanisation: a case study of Central Brahmaputra Valley, Assam, India
- Published: 24 February 2023
- Volume 88 , pages 3923–3934, ( 2023 )
Cite this article
- Ashish Saikia ORCID: orcid.org/0000-0003-4114-7204 1 &
- Bimal Kumar Kar ORCID: orcid.org/0000-0001-8168-4560 1
296 Accesses
2 Citations
Explore all metrics
Road connectivity is among the few factors which trigger the urbanisation and development process in a region. It facilitates trade, transport, social integration, economic development, socio-economic interaction and cultural assimilation. The present paper aims at analyzing the road infrastructure of Assam with special focus on the Central Brahmaputra Valley. The significance of the study area lies in providing smooth accessibility to the East Asian countries, especially in terms of Act East Policy. The study constitutes an investigation of the growth of road length, road density and connectivity in the study area in spatio-temporal context. Here, growth in road infrastructure has been compared with the growth in urban population and towns. Spatial variation in terms of growth can clearly be seen while discussing the growth of road connectivity and urban growth. Morigaon district has experienced the highest growth in terms of both road connectivity and urban population which clearly reveals that a strong positive relationship exists between the growth of road infrastructure and urban growth. The study has the potential of becoming a guideline for the policy makers to implement action plans for increasing the degree of accessibility in the region.
This is a preview of subscription content, log in via an institution to check access.
Access this article
Price includes VAT (Russian Federation)
Instant access to the full article PDF.
Rent this article via DeepDyve
Institutional subscriptions
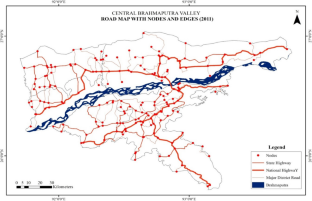
Source: The Authors
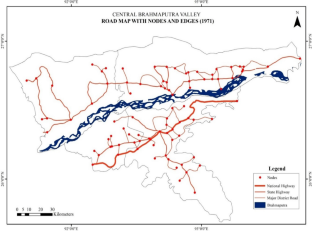
Similar content being viewed by others
Smart Transportation Systems in Smart Cities: Practices, Challenges, and Opportunities for Saudi Cities
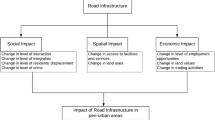
The Impact of Road Infrastructure Development Projects on Local Communities in Peri-Urban Areas: the Case of Kisumu, Kenya and Accra, Ghana

Evolution of Urban Transportation Policies in India: A Review and Analysis
Aljoufie, M., Zuidgeest, M., Brussel, M., & Van Maarseveen, M. (2013). Spatial—temporal analysis of urban growth and transportation in Jeddah City, Saudi Arabia. Cities, 31 , 57–68. https://doi.org/10.1016/j.cities.2012.04.008
Article Google Scholar
Clark, T. N., Lloyd, R., Wong, K. K., & Jain, P. (2002). Amenities drive urban growth. Journal of Urban Affairs, 24 (5), 493–515.
Das, P. (2008). Evolution of the road network in Northeast India: Drivers and brakes. Strategic Analysis, 33 (1), 101–116.
De, Prabir, India's Emerging Connectivity with Southeast Asia: Progress and Prospects (December 24, 2014). ADBI Working Paper 507. https://doi.org/10.2139/ssrn.2542510
Desai, A. R. (2005, 1948). Social background of Indian nationalism. Bombay: Popular Prakashan.
Duranton, G., & Turner, M. A. (2012). Urban growth and transportation. Review of Economic Studies, 79 (4), 1407–1440.
Foreign and Commonwealth Office. (2015). China’s regional diplomacy: Everybodyloves good neighbours, UK Government. https://www.gov.uk/government/uploads/system/uploads/attachment_data/file/446518/China_Regional_Diplomacy.pdf
Ganguly, J. B. (Ed.). (1995). Urbanization and development in north-east India: Trends and policy implications . Deep and Deep Publications.
Google Scholar
Garrison, W. L. (1960). Connectivity of the interstate highway system. Papers in Regional Science, 6 (1), 121–137.
Gogoi, B. (2013). Structural analysis of existing road networks of Assam: A transport geographical appraisal. International Journal of Scientific & Engineering Research, 4 (12), 1817–1823.
Grübler, A., & Nakićenović, N. (1991). Evolution of transport systems: past and future (Report No. RR-91-008). International Institute for Applied Systems Analysis, Laxenburg, Austria.
Guze, S. (2019). Graph theory approach to the vulnerability of transportation networks. Algorithms . https://doi.org/10.3390/A12120270
Hart, T., 2001. Transportation and the city. In: Paddison, R. (Ed.), Handbook of Urban Studies. Sage, London, pp. 102–121.
Hall, P., & Pfeiffer, U. (2013). Urban future 21: a global agenda for twenty-first century cities. Routledge.
Hurd, J., & Kerr, I. J. (2012). Indian railway history: A research handbook (pp. 3–4). Brill.
Book Google Scholar
Istrate, M. I. (2015). Assessment of Settlements’ Centrality in Botosani County using Shimbel Index. Jurnalul Practicilor Comunitare Pozitive, 15 (3), 57–69.
Jayasinghe, A. B., & Munasinghe, J. (2013). A study of the urbanizing pattern in Kegalle district, Sri Lanka with connectivity analysis. International Journal, 2 (1), 2305–1493.
Kansky, K. J. (1963). Structure of transportation networks: Relationships between network geometry and regional characteristics . The University of Chicago.
Khakha, A. (2019). Tribes and urbanisation in North East India. Economic & Political Weekly, 54 (38), 1–16.
Krugman, P. (1991). Increasing returns and economic geography. Journal of political economy, 99 (3), 483–499.
Krugman, P. (1996). The self-organizing economy. John Wiley & Sons.
Levinson, D. (2012). Network structure and city size. PLoS ONE . https://doi.org/10.1371/journal.pone.0029721
Lu, Y., & Tang, J. (2004). Fractal dimension of a transportation network and its relationship with urban growth: A study of the Dallas-Fort Worth area. Environment and Planning B: Planning and Design, 31 (6), 895–911.
Marx, K. (1974, 1853). The future results of British rule in India. In K. Marx & F. Engels (Ed.), Selected works (Vol. I, pp. 494–498). Moscow: Progress.
Nambiar, S. (2018). India’s connectivity with ASEAN: What role for Northeast India?. In Mainstreaming the Northeast in India’s Look and Act East Policy (pp. 131–158). Palgrave Macmillan, Singapore.
Pokharel, R., Bertolini, L., te Brömmelstroet, M., & Acharya, S. R. (2021). Spatio-temporal evolution of cities and regional economic development in Nepal: Does transport infrastructure matter? Journal of Transport Geography, 90 , 102904.
Rawat, D. S., & Sharma, S. (1997). The development of a road network and its impact on the growth of infrastructure: A study of almora district in the central himalaya. Mountain Research and Development, 17 (2), 117–126. https://doi.org/10.2307/3673826 .
Rodrigue, J. P., Comtois, C., & Slack, B. (2006). Transportation and geography. The Geography of Transport Systems, 1–37.
Saitluanga, B. L. (2013). Globalisation, urbanisation and spatial inequality in India with special reference to North East India. Saitluanga Space and Culture, India, 1 , 2.
Shimbel, A. (1953). Structural parameters of communication networks. The Bulletin of Mathematical Biophysics, 15 (4), 501–507. https://doi.org/10.1007/BF02476438
Srikanth, H. (2018). Look East Policy, Sub-regional Connectivity Projects and Northeast India. In Mainstreaming the Northeast in India’s Look and Act East Policy (pp. 213–231). Palgrave Macmillan, Singapore.
United Nations. (2018). 68% of the world population projected to live in urban areas by 2050, says UN | Umoja wa Mataifa. https://www.un.org/sw/desa/68-world-population-projected-live-urban-areas-2050-says-un
Xie, F., & Levinson, D. (2007). Measuring the structure of road networks. Geographical Analysis, 39 (3), 336–356.
Ziipao, R. R. (2018). Look/Act east policy, roads and market infrastructure in North–East India. Strategic Analysis, 42 (5), 476–489.
Ziipao, R. R. (2020). Roads, tribes, and identity in Northeast India. Asian Ethnicity, 21 (1), 1–21.
Download references
Acknowledgements
Not applicable.
We have not received any fund for completing the research work.
Author information
Authors and affiliations.
Department of Geography, Gauhati University, Gopinath Bordoloi Nagar, P.O., Kamrup Metro, Guwahati, Assam, 781014, India
Ashish Saikia & Bimal Kumar Kar
You can also search for this author in PubMed Google Scholar
Corresponding author
Correspondence to Ashish Saikia .
Ethics declarations
Conflict of interest.
We have no conflicts of interest to disclose.
Human and animal rights
The research does not include any experiments with animals or humans.
Additional information
Publisher's note.
Springer Nature remains neutral with regard to jurisdictional claims in published maps and institutional affiliations.
Rights and permissions
Springer Nature or its licensor (e.g. a society or other partner) holds exclusive rights to this article under a publishing agreement with the author(s) or other rightsholder(s); author self-archiving of the accepted manuscript version of this article is solely governed by the terms of such publishing agreement and applicable law.
Reprints and permissions
About this article
Saikia, A., Kar, B.K. Impact of road connectivity on urbanisation: a case study of Central Brahmaputra Valley, Assam, India. GeoJournal 88 , 3923–3934 (2023). https://doi.org/10.1007/s10708-023-10843-4
Download citation
Accepted : 06 February 2023
Published : 24 February 2023
Issue Date : August 2023
DOI : https://doi.org/10.1007/s10708-023-10843-4
Share this article
Anyone you share the following link with will be able to read this content:
Sorry, a shareable link is not currently available for this article.
Provided by the Springer Nature SharedIt content-sharing initiative
- Urbanisation
- Road connectivity
- Regression and correlation
- Road density
- Development
- Find a journal
- Publish with us
- Track your research
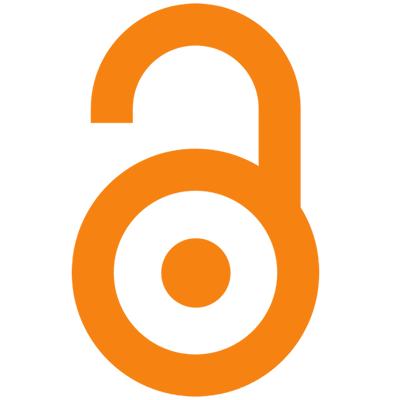
- Previous Article
- Next Article
INTRODUCTION
Overview of the study area, results and discussion, recommendations, acknowledgements, urbanisation, climate change and its impact on water quality and economic risks in a water scarce and rapidly urbanising catchment: case study of the berg river catchment.
- Article contents
- Figures & tables
- Supplementary Data
- Open the PDF for in another window
- Guest Access
- Cite Icon Cite
- Permissions
- Search Site
James D. S. Cullis , Annabel Horn , Nico Rossouw , Lloyd Fisher-Jeffes , Marlé M. Kunneke , Willem Hoffman; Urbanisation, climate change and its impact on water quality and economic risks in a water scarce and rapidly urbanising catchment: case study of the Berg River Catchment. H2Open Journal 1 January 2019; 2 (1): 146–167. doi: https://doi.org/10.2166/h2oj.2019.027
Download citation file:
- Ris (Zotero)
- Reference Manager
By 2050 it is predicted that 67% of the world population is expected to be living in urban areas, with the most rapid levels of urbanisation taking place in developing countries. Urbanisation is often directly linked to the degradation of environmental quality, including quality of water, air and noise. Concurrently, the climate is changing. Together, the negative impacts of climate change and urbanisation pose significant challenges, especially in developing countries where resources to mitigate these impacts are limited. Focusing on the Berg River Catchment in South Africa, which is experiencing increasing levels of urbanisation, the impacts of climate change, the ‘wicked problem’ of service delivery to the historically disadvantaged within a developing country, persistent infrastructure backlogs, and where high unemployment is prevalent, this paper explores the increasing water quality risks due to climate change and rapid urban development and the likely direct and indirect economic impacts that this will have on the agriculture sector, which is a key contributor to the regional and national economy. The results give support to the need to invest in risk mitigation measures including the provision of basic services, the upgrading and maintenance of wastewater treatment plants and investing in ecological infrastructure.
Countries around the world are increasingly faced with the challenge of managing the increasing risks and negative environmental impacts of climate change and urbanisation. By 2050 it is predicted that 67% of the world population is expected to be living in urban areas ( UN 2011 ), with the most rapid levels of urbanisation taking place in developing countries ( Zhang 2016 ). It is also likely, that despite our best efforts, the majority of future urban growth will be in informal areas as governments struggle to keep up with the increasing demand for basic services including water supply, sanitation and formal housing. Urbanisation is often directly linked to the degradation of environmental quality, including quality of water, air and noise ( Cullis et al . 2005 , 2018 ; Liang 2011 ; Bobylev et al . 2016 ; Zhang 2016 ).
Concurrently, it is now widely accepted that globally temperatures will increase and rainfall will become more variable, thereby affecting local climates across the world and that this can be largely attributed to human impacts ( IPCC 2014a ). Within urban areas, it is generally predicted that the increase in global temperatures associated with climate change will be exacerbated as a result of the urban heat island effect due to the modification of natural surfaces, where vegetation would have reduced heat ( IPCC 2014b ). Climate change is likely to also impact on water quantity and quality through a combination of higher temperatures and reduced freshwater flows ( Cullis et al . 2015 ). Increasing demand for water from upstream users also results in reduced fresh water flows which is further increasing the concentration of key pollutants and deteriorating water quality in rivers and associated economic risks for downstream users.
Both climate change and increasing urbanisation, particularly informal development, are likely to negatively impact on water quality of rivers which, in turn, could have significant economic impacts for the communities dependent on these rivers both for direct use, but also indirectly in terms of the water-dependent economic activities such as agriculture. Better understanding of the link between increasing water quality risks due to urban development and climate change and the associated economic impact that this has, is therefore, critical in terms of identifying the need for interventions, improved management and investments in ecological infrastructure to ensure sustainable development and to mitigate against the increasing risks associated with rapid urbanisation and climate change.
Here, we investigate these critical links by way of a case study of the Berg River Catchment in South Africa. The results of this study not only have relevance for decision-making in the Berg River Catchment, but also at a regional and national level for South Africa. The lessons learnt are also equally applicable in other developing world cities, and even in some regions of the developed world facing similar challenges of declining water quality due to increasing urbanisation and climate change.
We investigate the increasing water quality risks from urbanisation and climate change using water quality data obtained during a current three-year drought period that is the worst on record, but potentially an indicator of the future conditions under a drying climate. These data are also compared with the results of earlier studies to identify potential for long-term trends and possible cause of declining water quality. In order to investigate the economic risks associated with the observed increasing water quality risk, we investigate the impact of different water quality scenarios, including the net present value (NPV) of alternative mitigation measures at the level of individual representative farms found within the Berg River Catchment, and the overall impact that this might have on the economy both locally and nationally as a significant contributor to gross domestic product and foreign export earnings.
The Berg River Catchment, located in the Western Cape Province of South Africa provides a rare opportunity to study the potential environmental impacts/risks and their relationship to water quality and economic risks for both the urban and rural economies within a developing country context. The Berg River falls within a catchment that is experiencing increasing levels of urbanisation, the complex problems of service delivery to the historically disadvantaged informal sector, infrastructure backlogs and the prevalence of high unemployment. In this regard, the Berg River is not unique, in that many catchments face similar challenges, particularly in the developing world. As a result, the lesson learnt from this study and, in general, the lessons learnt from the Cape Town drought crisis, are globally relevant.
The Berg River in the Western Cape, South Africa, is an important water system as its upper reaches supply the City of Cape Town with a large proportion of its freshwater (two of the largest water supply dams are found in its upper reaches), while the middle reaches provide water for irrigation supporting a diversity of agriculture, as well as supporting the water needs of regional towns ( Görgens & de Clercq 2005 ). The Berg River rises in the Groot Drakenstein Mountains near the town of Franschhoek, and discharges into the Atlantic Ocean on the West Coast of South Africa near Velddrift. The Berg River is approximately 300 km in length, with the main stem approximately 160 km in length, and drains a catchment of roughly 9,000 km 2 , while traversing six local municipalities. The catchment lies in a Mediterranean climate, winter rainfall region with the average annual rainfall ranging from over 1,000 mm in the mountains in the east to 200 mm or less at the west coast. The land use in the Berg River Catchment falls primarily into four categories: agricultural (±60%), forestry (<1%), urban (±2.5%) and natural (±36%). Agricultural land use is further divided into irrigated (±7%) and dry land farming activities (±53%) ( DWAF 2004 ). While the catchment remains largely rural, it has seen an increase in population throughout the catchment (1996–2011) and an associated substantial increase in buildings (2006–2013), particularly in the middle-upper catchment around the major centres of Tulbagh, Wellington and Paarl ( Figure 1 ). Such trends are not surprising and align with the trend of increased urbanisation throughout South Africa. While the catchment has 18 waste water treatment works which treat domestic waste water, many of these are not operating optimally. In addition, the rapid rate of urbanisation and the growth of informal settlements has resulted in significant concerns in terms of the water quality impact of urban water runoff.

Population growth areas and the location of wastewater treatment works (WWTW) in the Berg River Catchment and water quality monitoring sites along the Berg River main stem used for analysis.
Poor water quality in the Berg River, resulting from poor sanitation service provision, agricultural and other practices, could have a significant impact on the regional economy. It is difficult to link economic findings to specific water quality levels, although if water quality continues to deteriorate to the point where international exports are affected, the economic consequences would be considerable. Similar challenges have been identified in neighbouring catchments such as the Breede River ( Cullis et al. 2018 ).
The concern, therefore, with the current trends of urbanisation, is that increasing populations will result in an increased impact on the river system, and because of its focus in the upper catchment the impacts will affect the entire river system. This could have an influence on the large number of agricultural users in the middle and middle-lower reaches of the Berg River who are an important component of the regional economy, in part due to the income generated from exporting produce.
The Berg River is a major water supply source to the City of Cape Town and other local and district municipalities in the region. Due to the seasonal nature of rainfall, water supply to these urban areas as well as to the farmers in the Berg River Catchment is dependent on several large dams and smaller farm dams, many of which are supplied from the Berg River itself using the river as a conveyance system for releases from the upstream dams, particularly during the summer months. The three largest dams in the catchment are the Berg River Dam, Wemmershoek Dam and Vöelvlei Dam which form part of the Western Cape Water Supply System (WCWSS). Maintaining minimum environmental flows to the Berg River estuary is also critical given the ecological importance of this estuary as well as its contribution to the sustainability of fish stocks that support the fishing industry in the region. Competition for water will affect the cost of irrigation water significantly in the future. The expectations that water availability and/or water quality can be influenced will ripple through to farm-level profitability.
Review of historical water quality and flow data for the Berg River
The water quality in the Berg River Catchment has been monitored since, at least, 1967 ( De Villiers 2007 ). Since 2005 there have been a number of studies ( Görgens & de Clercq 2005 ; Clark & Ractliffe 2007 ; Jackson et al . 2007 ; Paulse et al . 2007 ; Struyf et al . 2012 ) that have considered different aspects of water quality in the Berg River and other rivers in the region ( Cullis et al. 2018 ). These studies have utilised data sets dating back as far as 1985. Unfortunately, the available data sets have been collected by a variety of stakeholders and, as such, do not always represent the same points along the water course – which limits comparison – and are not always located near a flow gauging station – limiting the assessment of the total flux (load) of pollutants in the river system. Interestingly, there does not appear to be a single study, to date, that has considered all the different water quality parameters that have been monitored, namely, water chemistry, microbiology and metals, and yet there are similarities in trends in the conclusions reached in the different studies.
Analysis of water quality data from the Berg River Improvement Project
In addition to the review of data from previous water quality studies, water quality data obtained from the Berg River Improvement Project (BRIP) and the National Department of Water and Sanitation (DWS) were also analysed in terms of the impact of urbanisation and drought on water quality, particularly for the last three years during which the region has experienced a severe drought that almost resulted in the City of Cape Town becoming the first global city to run out of water. The BRIP was started by the Department of Environmental Affairs & Economic Development (DEA&DP) of the Western Cape Government in October 2013. Water quality samples were collected mostly at a monthly frequency. Data for the period October 2013 to October 2017 were provided for this assessment. The location of the sampling points in relation to key features along the Berg River are given in Table 1 and in Figure 2 .
List of Berg River Improvement Project (BRIP) sampling points on the Berg River
BRIP ID# . | Site description . | Lat . | Long . |
---|---|---|---|
B10 | 1 km downstream of Klein Berg confluence | −33.207516 | 18.943699 |
B11 | Berg River downstream of Wellington WWTW | −33.656089 | 18.9666 |
B12 | Berg River downstream of the Mbekweni storm water channels | −33.66682 | 18.974746 |
B13 | Berg River downstream of Paarl | −33.690659 | 18.97549 |
B14 | Berg River at Arboretum | −33.755504 | 18.972971 |
B15 | Drakenstein stream Wemmershoek Dam | −33.807679 | 19.076892 |
B17 | Franschhoek River at Rickety bridge | −33.898961 | 19.093116 |
B18 | Berg River Dam bridge | −33.882484 | 19.047197 |
B20 | Bienne Donne/Downstream of Berg Dam Pump scheme | −33.841534 | 18.9875 |
B21 | Stiebeuels River 50 m d/s of Main Road | −33.896256 | 19.098658 |
B22 | Klein Berg River at Tulbagh Nursery | −33.319134 | 19.095975 |
BRIP ID# . | Site description . | Lat . | Long . |
---|---|---|---|
B10 | 1 km downstream of Klein Berg confluence | −33.207516 | 18.943699 |
B11 | Berg River downstream of Wellington WWTW | −33.656089 | 18.9666 |
B12 | Berg River downstream of the Mbekweni storm water channels | −33.66682 | 18.974746 |
B13 | Berg River downstream of Paarl | −33.690659 | 18.97549 |
B14 | Berg River at Arboretum | −33.755504 | 18.972971 |
B15 | Drakenstein stream Wemmershoek Dam | −33.807679 | 19.076892 |
B17 | Franschhoek River at Rickety bridge | −33.898961 | 19.093116 |
B18 | Berg River Dam bridge | −33.882484 | 19.047197 |
B20 | Bienne Donne/Downstream of Berg Dam Pump scheme | −33.841534 | 18.9875 |
B21 | Stiebeuels River 50 m d/s of Main Road | −33.896256 | 19.098658 |
B22 | Klein Berg River at Tulbagh Nursery | −33.319134 | 19.095975 |

Schematic diagram of the upper and middle Berg River showing the location of BRIP and DWS sampling points and key WWTW.
The National Chemical Monitoring Programme (NCMP) of the DWS also collects water quality data along the Berg River. Data for the period January 2013 to May 2017 were obtained from the DWS for the monitoring points ( Figure 2 ) listed in Table 2 . The sampling frequency at the NCMP points vary although samples are mostly collected at a monthly frequency.
Department of Water and Sanitation (DWS) National Chemical Monitoring Programme sampling points selected for this study to complement the BRIP water quality data set
DWS code . | Name . | Latitude . | Longitude . |
---|---|---|---|
G1H003 | G1H003Q01 At Le Mouillage La Motte on Franschhoekrivier | −33.8906 | 19.07889 |
G1H020 | G1H020Q01 At Dal Josafat Noorder Paarl on Berg River | −33.7075 | 18.97444 |
G1H036 | G1H036Q01 At Vleesbank Hermon Bridge on Berg River | −33.435 | 18.95694 |
G1H013 | G1H013Q01 At Drieheuvels on Berg River | −33.1308 | 18.86278 |
G1H031 | G1H031Q01 At Misverstand Die Brug on Berg River | −32.9969 | 18.77889 |
DWS code . | Name . | Latitude . | Longitude . |
---|---|---|---|
G1H003 | G1H003Q01 At Le Mouillage La Motte on Franschhoekrivier | −33.8906 | 19.07889 |
G1H020 | G1H020Q01 At Dal Josafat Noorder Paarl on Berg River | −33.7075 | 18.97444 |
G1H036 | G1H036Q01 At Vleesbank Hermon Bridge on Berg River | −33.435 | 18.95694 |
G1H013 | G1H013Q01 At Drieheuvels on Berg River | −33.1308 | 18.86278 |
G1H031 | G1H031Q01 At Misverstand Die Brug on Berg River | −32.9969 | 18.77889 |
The BRIP water quality data, which included parameters listed in Table 3 , were combined with the DWS data ( Table 2 ) for analysis. DWS does not routinely monitor dissolved organic carbon (DOC) and chemical oxygen demand (COD), and no Escherichia coli samples have been collected by DWS since about June 2013. The data were then analysed in terms of both spatial and temporal variation to investigate the impact of changing land use, as well as the impact of wet and dry seasons as an indication of conditions under a possible drier future climate.
Parameters analysed for in the BRIP
BRIP parameter names . | Units . | NCMP parameter names . | Units . |
---|---|---|---|
Ammonium (NH -N) | mg/L | NH -N-Diss-Water (ammonium nitrogen) | mg/L |
Alkalinity (Alk) as CaCO | mg/L | TAL-Diss-Water (total alkalinity as calcium carbonate) | mg/L |
Nitrate plus nitrite nitrogen (NO + NO -N) | mg/L | NO + NO -N-Diss-Water (nitrate + nitrite nitrogen) | mg/L |
Ortho-phosphate (PO -P) | mg/l | PO -P-Diss-Water (ortho phosphate as phosphorus) | mg/L |
Dissolved organic carbon (DOC) | mg/L C | – | – |
Electrical conductivity (EC) | mS/m (25 °C) | EC-Phys-Water (electrical conductivity) | mS/m (25 °C) |
pH | pH units (20 °C) | pH-Diss-Water (PH) | (pH units) |
Chemical oxygen demand (COD) | mg/L | – | – |
per 100 mL | – | – |
BRIP parameter names . | Units . | NCMP parameter names . | Units . |
---|---|---|---|
Ammonium (NH -N) | mg/L | NH -N-Diss-Water (ammonium nitrogen) | mg/L |
Alkalinity (Alk) as CaCO | mg/L | TAL-Diss-Water (total alkalinity as calcium carbonate) | mg/L |
Nitrate plus nitrite nitrogen (NO + NO -N) | mg/L | NO + NO -N-Diss-Water (nitrate + nitrite nitrogen) | mg/L |
Ortho-phosphate (PO -P) | mg/l | PO -P-Diss-Water (ortho phosphate as phosphorus) | mg/L |
Dissolved organic carbon (DOC) | mg/L C | – | – |
Electrical conductivity (EC) | mS/m (25 °C) | EC-Phys-Water (electrical conductivity) | mS/m (25 °C) |
pH | pH units (20 °C) | pH-Diss-Water (PH) | (pH units) |
Chemical oxygen demand (COD) | mg/L | – | – |
per 100 mL | – | – |
Determining the financial and economic impacts of declining water quality for agriculture
Farmers operate at the interface between physical-biological and financial-economic dimensions, which means that various physical quantities and environmental parameters will be important drivers. Farm management is defined as the process by which resources and situations are manipulated by the farm manager in trying, with less than full information, to achieve his goals ( Dillon 1980 ). This definition, in essence, relates to a decision-making process involving the identification and evaluation of alternatives. It would therefore be reasonable to expect that if the water quality in the Berg River continues to deteriorate, the agricultural sector will try to adapt. Adaption – such as treatment of river water – would incur additional costs which would likely be passed onto the consumer and affect the competitiveness of farmers in the international market.
Concerns around the effect of the water quality of irrigation water in 2008 strongly motivated the need to prevent further degradation of water quality in the Berg River, failing which there could be significant economic consequences if the international export market were to limit their acceptance of produce from the region. While there would be benefit to all stakeholders in managing the water quality in the river, in order to protect their livelihoods the agricultural sector could, potentially, treat water prior to use for irrigation to ensure it meets international standards. In order to assess the scale of the decision to treat irrigation water a set of ‘whole-farm budget models’ were developed for the different types of agriculture currently taking place in the Berg River Catchment.
‘Whole-farm budget models’ are simulation models that simply express how a farm operates and the financial and economic consequences of its operation. By manipulating the parameters and inputs of the model, research questions of a descriptive, causal and predictive nature can be addressed ( Steward 1993 ; Brenner & Werker 2007 ; Jakku & Thornburn 2010 ; Douthwaite & Hoffecker 2017 ). A high level overview of the models used in this study is provided in Figure 3 , and described in more detail in Hoffmann (2011) .

A graphical representation of the components of the whole-farm, multi-period budget model.
Financial modelling is typically used in studies that aim at developing and validating accurate representations of the real world, in this instance a farm using river water for irrigation, and allow for the evaluation of possible alternative outcomes ( Pannell 1996 ; Dorward et al . 1997 ; Kuehne et al . 2017 ). Budgeting is perhaps the most widely used method of financial planning. Budgeting, as a non-optimising method evaluates plans in physical and financial terms ( Nance & Sargent 2002 ; Daellenbach & McNickle 2005 ). The models provide an indication of a farm's gross margin, the net annual cash flow, the internal rate of return (IRR) on capital investment and NPV. Farm systems are complex, more because of the interrelationships within the farm structure than by the different tangible components. Assessing the risk involved with irrigation availability is therefore dependent on the integration of the various components of farming and assessment of these factors at the whole-farm level.
The impact of trends, strategies and policy options on whole-farm profitability can be assessed by using a typical, or representative farm. In essence, it is a synthetically constructed model farm based on the expected structure of a farm in a particular area ( Ash et al . 2017 ). The farm sizes and land distribution identification for the typical farms was done according to statistics obtained from industry organisations ( Hortgro 2016 ; SAWIS 2016 ; SATI 2017 ). The farm description for each area was presented to a study group of industry role-players for validation of the assumptions.
The various enterprises are integrated into the whole-farm gross margin level. Expected income and production cost as well as the calculated gross margin per hectare level are based on information provided by the study group of industry role-players. Fixed and overhead costs are subtracted to calculate a figure that would resemble net farm income. From this annual figure, the capital replacement is subtracted to give the net annual flow after capital replacement.
The model is structured to show the impact of changes in inputs or output quantities, input and output prices, fixed costs' levels, changes in land utilisation, crop replacements schedules and movable asset replacement costs. The profitability indicators, IRR and NPV are calculated on a capital budget format and thus already include capital replacements for aspects such as machinery and orchards and vineyards in normal farming cycles. The normal increase in land values is ignored. It is important to note that the IRR in this case is a real return, in other words, one should still add inflation to calculate the nominal rate of return. The discount rate that was used for the calculation of the NPV is 2.4% which is a real rate based on the Repo rate.
In this study, three water quality and on farm response scenarios were considered. The first scenario assumed the water quality in the Berg River remained acceptable for agricultural use and met international export standards and farmers would continue to operate their farms, without any changes. The second scenario assumed that the water quality in the Berg River continued to decline, and as a result farmers began to see a decrease in productivity, but the water quality no longer met international export standards. The third scenario assumed that in order to protect their livelihoods farmers installed adequate water treatment facilities to ensure the quality of water utilised for irrigation was acceptable.
The first scenario for the purpose of this excercise is the control or status quo situation, or the prefered situation. The second scenario resembles a possible situation where all the export fruit would need to be sold domestically. That is, for example, a price decrease for table grapes to domestic prices, which would make table grapes unprofitable and structurally farmers would most likely change to wine grape production or other fruits with lower quality standards or higher yields. The third scenario entails the establishment of a water purification plant. A minimum cost of R1.5 m for the whole farm is assumed as this was the cost for a private producer who installed such a system in 2017. In this scenario, the added infrastucture cost and electricity cost is increased in the whole-farm budget model used for the analysis.
Water quality trends in the Berg River Catchment
It is evident, as would be expected, that as the catchment has developed – principally through increased agriculture and urbanisation – the water quality in the Berg River has deteriorated ( DWAF 2004 ). De Villiers (2007) noted that there has been a seasonal change in certain water nutrient levels, resulting from anthropogenic factors, that exceed national and internationally accepted levels. De Villiers (2007) indicates that the two most likely anthropogenic sources of nutrients along the Berg River are agricultural runoff, including pesticides and fertilisers, and also effluent from over-loaded municipal sewage works and runoff from informal settlements. These findings were made prior to the completion of the Berg River Dam, and were accompanied by a warning that if the dam resulted in the reduction of the downstream flushing effect, it would be very likely that nutrient levels in the Berg River would significantly increase above their already unacceptably high levels.
The importance of the Berg River Improvement Project is that it has investigated whether the pollution levels have continued to increase, and has the potential to show whether the Berg River Dam has had any impact. The Berg River Dam was designed to make environmental releases, however in the height of the current drought, these have been suspended. De Villiers (2007) investigated both the pollution concentrations in the river and also the flux or total mass of these pollutants.
In light of De Villiers (2007) , it is of concern to note the findings of Struyf et al . (2012) , who investigated the changes in riparian nutrient dynamics along the Berg River. They found that nutrient concentrations in the sediments within the riparian zone reflect the concentrations of nutrient in the Berg River. Significantly, they noted that sediments close to the river had more efficient recycling and export of nutrients into the river. Struyf et al . (2012) highlighted that their study indicated that if the changes in the hydrological cycle, in particular reduced flow, and water quality levels suggested in De Villiers (2007) were to come to fruition, it would be highly likely that a number of ecosystem goods and services would be impacted – including the natural filter function of the river system. Furthermore, Struyf et al . (2012) suggest that due to the impact of the high nutrient levels on the riparian zone, it may in future become a source of nutrients for the river, further compounding the challenges in managing the river.
Paulse et al . (2007) utilised a section of the Berg River to compare different enumeration techniques for the investigation of bacterial pollution. Incidentally their study highlighted that the majority of samples fell outside of the maximum acceptable levels. While the study is not representative of the whole river, it is an indication that the river is affected by anthropogenic pollution. Jackson et al . (2007) investigated the metal contamination of a section of the Berg River. Metals are typically only found as trace elements in the environment, as such when their concentrations increase significantly they may become toxic to the surrounding environment. The study found concentrations of several metals to be consistently above the available guidelines. The sources of these pollutants were, speculatively, linked back to informal settlements and agriculture, and highlighted the need for improved service provision in informal settlements and better management of pesticides, within agriculture.
Long term, the phosphate (PO 4 3− ) levels in the Berg River indicate reason for concern. When the total at B10 (this study) is compared to the results of previous studies (B4 from De Villiers (2007) ) there is a strong long-term trend ( Figure 4 ) of increasing loads of phosphate. This trend is also present upstream of Paarl, to a lesser extent (B2 – De Villiers (2007) ; B14 – this study). If nothing is done to mitigate the increasing pollution risks, it is likely that by early 2020 (B10) and 2050 (B14), levels of phosphate will be unacceptable, while assuming the flow in the river remains constant. It is expected that there will be increasing periods where the level of phosphate will exceed the unacceptable levels. It is worth noting that B10 is lower down in the catchment and thus has a higher flow and, as such, the total acceptable flux (load) of pollutants that is acceptable would be higher. The increase in phosphate is more likely the result of urban development, rather than agriculture, as PO 4 3− does not easily leach from the soil, and evidence (discussed below) is that agriculture's impact on the nutrients in the river is decreasing, hence, addressing concerns around the compliance of wastewater treatment works (WWTWs) as well as the provision of basic sanitation services and improved stormwater management of the informal areas is critical in terms of reducing the pollution risk.

Long-term prediction of total flux of phosphate (PO 4 3− ).
Unlike the flux of phosphate, the concentrations and flux of nitrate (NO x ) along the length of the river shows no consistent trends (data not shown) and does not result in a similar long-term increasing trend when compared to the results of De Villiers (2007) . The nitrate concentration profiles do however peak during months with high runoff conditions. This is consistent with a diffuse source – which could be attributed to either agricultural runoff or diffuse urban pollution and the flushing out of the drainage systems servicing informal settlements at the start of the winter rainfall period. It is difficult to identify the specific source of nitrate pollution except to note that above Paarl (B14) there is a long-term trend indicating a reduction in the annual flux (load). The upper catchment for B14 includes a significant proportion of the agriculture, namely, vineyards and orchards, thus suggesting that agriculture in this region may be reducing their impact on the river through improved practices. It appears that downstream of B11, the flux of both phosphate and nitrate has increased since 2005. While agriculture in the catchment downstream of Paarl may be contributing to the increase, it is possible, if not likely, that the increase in pollution is due to urbanisation and its associated impacts on the catchment.
In summary, it is evident that there have been significant water quality impacts on the entire Berg River Catchment and that these have continued to deteriorate in the face of rapid urbanisation and that they need to be addressed as they present a significant economic risk to the region. If climate change (and increasing demands) results in further reduction in runoff, then the concentrations of nutrients in the river will increase with the reduced flows in the river and if the nutrient contribution to the river remains the same. This was observed during the last three years of the drought as described below.
Urban development and agricultural impacts on water quality
A vast amount of water quality data was collected as part of the study and analysed on behalf of the DEA&DP. This paper focuses on the results from only five water quality parameters, which together provide insight into the current levels of pollution in the catchment, possible causes, and potential risks and impacts of pollution. The analysis particularly compares trends along the river reach as well as the differences in the observed trends during wet and dry years which were both observed during the period.
Figures 5 and 6 provide a summary of the water quality data collected along the course of the Berg River Catchment for five key parameters that can then be used to investigate the likely consequences of urban developments, both formal and informal, along the length of the river as well as the potential impact due to the current state of the various WWTW.

Summary of water quality data for ammonia (NH 4 ), phosphates (PO 4 ), nitrates (NO 3 + NO 2 ) and salinity (EC) for the BRIP sampling locations from upstream (1) to downstream (12) for the period 2013 to 2017. The median values for each location are shown in the centre of the box which represents the upper and lower quartile. The whiskers represent the 5th and 95th percentile of the data and the other dots indicate extreme, or possible outlier results.

Microbial water quality results for the Berg River from upstream (B17) to downstream (G1H031). The median values for each location are shown in the centre of the box which represents the upper and lower quartile. The whiskers represent the 5th and 95th percentile and the other dots indicate extreme, or possible outlier results.
The results show a clear spike in NH 4 and PO 4 concentrations at the location of sampling point B12 downstream of the Mbekweni informal settlement. There is a slight increase at B13, downstream of the Paarl WWTW and the spike persists at B11 downstream of the Wellington WWTW. After this point the river flows predominantly through an area of agricultural land use and by G1H036 the NH 4 and PO 4 concentrations are reduced to normal. This highlights the importance of natural river processes in terms of reducing the pollution load and the importance of maintaining ecologically functioning river corridors. There is also a slight increase at B17 which is located downstream of an informal settlement in Franschoek.
A similar trend is not seen for nitrates, which peak at G1H036. This suggests that agricultural non-point source pollution is a greater contributor to nitrate pollution rather than urban water runoff or WWTWs. Interestingly, at B14, located upstream of Paarl, but also downstream of an area of intense agriculture, there is an indication of a long-term decrease in nitrate concentrations. This is consistent with the findings in De Villiers (2007) and could be due to improved agricultural practices including buffer strips.
Increasing salinity in the Berg River is a concern for agriculture. This is partially due to the underlying geology which consists of Malmesbury shale, which contributes significant levels of natural salinity to the catchment runoff, particularly in the lower sections of the river. It is thought that deep ploughing of these catchments has potentially resulted in additional salinity loads and there have also been concerns that the damming of the headwaters of the Berg River through the construction of the Berg River Dam in 2010 has further contributed to increasing salinity levels by reducing the amount of fresh water entering the system. However, due to the irrigation releases from the Berg River Dam during the summer months, the salinity is reduced. Towards the end of the growing season, as the irrigation releases decrease, there is an increase in the salinity concentrations The general downstream increase in salinity or electrical conductivity (EC), shown in Figure 5 and the seasonal trend is an indication that agriculture is having some impact on water quality, but as evidenced in the changes in EC through Wellington, Mbekweni and Paarl, it is highly likely that these urban and informal areas are also contributing to the increase in EC.
The E. coli levels and distribution along the water course, shown in Figure 6 , clearly highlights two potential sources of faecal pollution – (1) WWTW that are not compliant and (2) drainage from informal settlements. This conclusion is based on the following observations:
There is evidence below monitoring point B21, where the Stiebeuels River draining Langrug informal settlement joins the Berg River, that the E. coli levels are elevated. Based on the data there appears to have been a seasonal trend during 2014–2015 with the peak concentrations found during winter. Interestingly, during 2016–2017 the seasonality has reversed, with winter concentrations remaining approximately the same as during the 2014–2015 period. This may be due to the build-up of faecal waste in the drainage system draining the Langrug informal settlement – which would normally have been washed out during the winter season but has not due to the drought (2015–2017).
The releases from the Berg River Dam as well as the relatively un-impacted flows in the Dwars River appear to dilute the E. coli concentrations and contribute to an improvement in water quality until the river reaches Paarl (B20). Also due to the benefits of natural river functioning.
The increase in E. coli levels between upstream (B14) and downstream (B12) of the Paarl WWTW and the Mbekweni informal settlement is a further indication of the impact of inadequately serviced settlements and struggling WWTW, on the water quality in the catchment.
The level of compliance with microbial pollutants is of concern as these have a direct link to international standards for crop exports. The financial flows from these exports form a significant part of the local and national economy and so the quality of irrigated water from the Berg River needs to be managed. E. coli , an indication of faecal pollution, is typically at ‘tolerable’ and ‘unacceptable’ levels throughout the upper reaches of the Berg River Catchment. E. coli only shows consistently acceptable levels in the vicinity of the Misverstand Dam – well downstream of most urban areas.
The compliance summary for the WWTW along the Berg River, shown in Table 4 indicates significant long-term compliance – especially microbiological and chemical – challenges for a number of the WWTW in the upper catchment. Addressing these deficiencies through the rehabilitation and upgrading of the relevant treatment plants is critical. Upgrading the treatment plants, however, will not entirely eliminate the risk of pollution without also addressing the concerns about the non-point source pollution impacts from informal settlements. The decrease in the E. coli concentrations further downstream, however, also indicates the importance of maintaining river functioning as these natural processes do assist in mitigating the downstream risks associated with upstream development and poorly functioning WWTW. The protection and rehabilitation of instream habitats and riparian banks is therefore critical and is a primary focus of the interventions of the Berg River Improvement Project.
State of compliance of WWTW in the Berg River Catchment
WWTW name . | Capacity (ML/d) . | Monitoring compliance (%) . | Microbial compliance (%) . | Physical compliance (%) . | Chemical compliance (%) . |
---|---|---|---|---|---|
Saron | 1 | 98.58 | 75 | 94.06 | 73.91 |
Hermon | 0.3 | 100 | 0 | 0 | 0 |
Pearl Valley | 2 | 100 | 44.57 | 94.49 | 69.57 |
Paarl | 35 | 97.67 | 80.43 | 91.06 | 39.53 |
Wellington ( Wellington has not discharged into the river since 2013. Sewage is pumped to Paarl WWTW during upgrade) | 7 (currently being upgraded to 16) | 100 | 62.5 | 92.67 | 76.81 |
Gouda | 0.64 | 100 | 100 | 90.91 | 11.76 |
Wemmershoek | 5 | 90.33 | 72.73 | 83.72 | 90.91 |
Pniel | 1.315 | 97.5 | 91.67 | 82.22 | 60.42 |
WWTW name . | Capacity (ML/d) . | Monitoring compliance (%) . | Microbial compliance (%) . | Physical compliance (%) . | Chemical compliance (%) . |
---|---|---|---|---|---|
Saron | 1 | 98.58 | 75 | 94.06 | 73.91 |
Hermon | 0.3 | 100 | 0 | 0 | 0 |
Pearl Valley | 2 | 100 | 44.57 | 94.49 | 69.57 |
Paarl | 35 | 97.67 | 80.43 | 91.06 | 39.53 |
Wellington ( Wellington has not discharged into the river since 2013. Sewage is pumped to Paarl WWTW during upgrade) | 7 (currently being upgraded to 16) | 100 | 62.5 | 92.67 | 76.81 |
Gouda | 0.64 | 100 | 100 | 90.91 | 11.76 |
Wemmershoek | 5 | 90.33 | 72.73 | 83.72 | 90.91 |
Pniel | 1.315 | 97.5 | 91.67 | 82.22 | 60.42 |
Source : DWS Greendrop Drop Report (January 2018).
Drought impacts and climate change
While climate change is considered to have a major impact on the water resources of South Africa, there exists significant uncertainty regarding the specific impacts on the Berg River Catchment, particularly in terms of the potential for a decrease in runoff. Using a 10-year moving average ( Figure 7 ), there appears to be little change in the annual runoff – this could in part be ‘masked’ by how the Berg River Dam is operated and the relatively short record since its construction. It is apparent that there is significant variability in rainfall between years, and that the period 1985–1995 had higher than average runoff, but based on the available flow data it is difficult to conclusively note any climate change impacts. While there appears to be little change in runoff since 1995, indications are that in the long term it is more likely that flows in the river will decrease ( DEA 2013 ; Cullis et al . 2015 ).

Changes in the annual and 10-year moving average for flow (Mm 3 /a) in the Berg River at G1H013.
More importantly, while the long-term averages may not change, extremes may become more frequent. This could prove significant as the flux of pollutants is not directly proportional to rainfall and flow – especially for point sources such as WWTW and drainage discharges from informal settlements. As such, the concentrations of pollutants from these sources will likely increase substantially during periods of drought. This is particularly evident in the increase in all pollution parameters between upstream (B14) and downstream (B12) of the Paarl WWTW and the Mbekweni informal settlement during 2016–2017 – as the region experienced drought conditions. A comparison of the water quality results from 2013 to 2014 (wet years) vs 2016 to 2017 (dry years) shows a significant difference in the water quality concentrations indicating the potential impact the more frequent dry years could have on the overall water quality of the Berg River ( Figure 8 ). In addition, increasing temperatures due to climate change are likely to result in higher demands for water, which would result in even less streamflow being maintained in the river to assist in the dilution of the pollution impacts due to both urban and agricultural development.

Time series of selected water quality parameters at site B11, downstream of Wellington WWTW showing the impact of the drought, particularly the very low flows and high concentrations in 2017.
Economic impacts of declining water quality on the agricultural sector
The diversity of agricultural activity in the Berg River Catchment can be subdivided into a number of smaller homogeneous production areas. These include:
Franschhoek: Wine grapes, stone fruit and pome fruit
Paarl: Table grapes, wine grapes and stone fruit
Wellington: Wine grapes, table grapes and stone fruit
Swartland: Winter cereals, small stock and potatoes.
Table 5 indicates the actual and relative size of the different farming activities. The area is clearly an important producer of table grapes and wine grapes, as in this area, there are 120 out of a total of only 493 private wine cellars across the whole of South Africa. This shows the agricultural value adding that is produced within the Berg River irrigation area, particularly in terms of export earnings and tourism.
The actual and relative (to South Africa) contribution of table grape production, wine grape production and fruit production of the Berg River area
. | Actual . | % of South African total . |
---|---|---|
Table grapes (4.5 kg carton equivalent) | 15,426,175 | 23% |
Wine grapes (number of vines) | 45,876,435 | 16.17% |
Fruit | ||
Nectarines (ha) | 210 | 9.9% |
Pears (ha) | 132 | 1.1% |
. | Actual . | % of South African total . |
---|---|---|
Table grapes (4.5 kg carton equivalent) | 15,426,175 | 23% |
Wine grapes (number of vines) | 45,876,435 | 16.17% |
Fruit | ||
Nectarines (ha) | 210 | 9.9% |
Pears (ha) | 132 | 1.1% |
Sources : SATI (2017) , SAWIS (2016) and Vinpro (2017) .
The figures in Table 5 are calculated from the agricultural industry information that is published annually. It shows the importance of agricultural activity in an area where the economic activities in the area are further strongly linked to farming, such as agri-processing and tourism.
Table 6 shows the importance of agriculture as a contributor to employment and the money spent on labour, as a significant component of the expenditure on intermediaries. The potential farm-level financial impacts if irrigation water quality deteriorates would directly impact on the producers' income first of all, which would affect their ability to employ farm labour. If structural adaptations are made, such as a move towards wine grape production and away from table grape production, it will have a decreasing impact on all inputs, but especially direct employment on farm level. There would be a necessary time lag to put these physical changes into place. This is a likely scenario if water quality deteriorates to the extent that export phytosanitary standards for fruit and table grapes are not met.
The economic contribution of the main farming activities as expressed in expenditure on intermediaries (inputs), the producers' income and expenditure on labour
. | Expenditure on intermediaries . | Producers' income . | Expenditure on labour . |
---|---|---|---|
Table grapes | 454,844,220 | 570,768,475 | 285,640,510 |
Wine grapes | 575,658,720 | 813,351,000 | 205,042,320 |
Fruit | |||
Nectarines | 30,624,510 | 47,411,100 | 4,393,200 |
Pears | 26,182,068 | 35,128,632 | 3,119,556 |
. | Expenditure on intermediaries . | Producers' income . | Expenditure on labour . |
---|---|---|---|
Table grapes | 454,844,220 | 570,768,475 | 285,640,510 |
Wine grapes | 575,658,720 | 813,351,000 | 205,042,320 |
Fruit | |||
Nectarines | 30,624,510 | 47,411,100 | 4,393,200 |
Pears | 26,182,068 | 35,128,632 | 3,119,556 |
a Expenditure on labour is included in total intermediaries.
Table 7 shows the farm size and cultivated area for the typical farm in each relatively homogenous area of the Berg River. Table 8 presents the land use pattern for the typical farm for each of the relatively homogeneous areas of the Berg River. The land use patterns were identified in the same way as the farm description. Franschhoek is mostly a wine grape production area with some fruit production, while table grapes and fruit predominate in Paarl and Wellington. The Swartland farm is in the Sandveld area closer to the Atlantic Ocean and some centre-pivot irrigation is used in combination with rain-fed winter cereal and pasture farming.
Farm size and land use for the typical farm of each relatively homogenous area
. | Farm size . | Cultivated land . |
---|---|---|
Franschhoek | 150 ha | 69 ha |
Paarl | 200 ha | 69 ha |
Wellington | 130 ha | 60 ha |
Swartland | 850 ha | 822 ha |
. | Farm size . | Cultivated land . |
---|---|---|
Franschhoek | 150 ha | 69 ha |
Paarl | 200 ha | 69 ha |
Wellington | 130 ha | 60 ha |
Swartland | 850 ha | 822 ha |
Land use pattern (hectare) for each typical farm for the Berg River irrigation area
. | Franschhoek . | Paarl . | Wellington . | Swartland . |
---|---|---|---|---|
. | Ha . | Ha . | Ha . | Ha . |
Wine grapes | ||||
Chenin blanc | 9.1 | 4.3 | 5.3 | |
Colombard | 2.9 | 5.6 | 1.7 | |
Sauvignon blanc | 2.9 | 4.3 | 1.7 | |
Chardonnay | 6.6 | 5.0 | 3.8 | |
Cabernet sauvignon | 10.4 | 4.0 | 6.0 | |
Pinotage | 3.7 | 3.4 | 2.2 | |
Shiraz | 3.7 | 2.8 | 2.2 | |
Merlot | 2.1 | 1.6 | 1.2 | |
Ruby cabernet | 2.5 | 1.9 | 1.4 | |
Peaches | ||||
Keisie | 3.5 | 1.3 | 2.3 | |
Kakemas | 3.5 | 1.3 | 2.3 | |
Oom Sarel | 2.5 | 0.9 | 1.6 | |
Sandvliet | 0.8 | 0.3 | 0.5 | |
Neethlings | 0.6 | 0.2 | 0.4 | |
Malherbes | 1.0 | 0.4 | 0.6 | |
Cascade | 2.1 | 0.8 | 1.4 | |
Plums | ||||
Souvenir | 2.8 | 1.0 | 1.8 | |
Harry Pickstone | 4.1 | 1.6 | 2.7 | |
Apricots | 6.9 | 2.6 | 4.5 | |
Table grapes | ||||
Crimson seedless | 4.1 | 2.7 | ||
Red globe | 5.2 | 3.4 | ||
Sugranineteen | 4.1 | 2.7 | ||
Thomson seedless | 4.7 | 3.1 | ||
Regal | 3.9 | 2.5 | ||
Dun-ben-hanna | 2.2 | 1.4 | ||
Autumn royal | 1.7 | 1.1 | ||
Sunred seedless | 1.7 | 1.1 | ||
Rain-fed crops | ||||
Winter cereals | 603 | |||
Pastures | 149 | |||
Vegetables | ||||
Potatoes | 25 |
. | Franschhoek . | Paarl . | Wellington . | Swartland . |
---|---|---|---|---|
. | Ha . | Ha . | Ha . | Ha . |
Wine grapes | ||||
Chenin blanc | 9.1 | 4.3 | 5.3 | |
Colombard | 2.9 | 5.6 | 1.7 | |
Sauvignon blanc | 2.9 | 4.3 | 1.7 | |
Chardonnay | 6.6 | 5.0 | 3.8 | |
Cabernet sauvignon | 10.4 | 4.0 | 6.0 | |
Pinotage | 3.7 | 3.4 | 2.2 | |
Shiraz | 3.7 | 2.8 | 2.2 | |
Merlot | 2.1 | 1.6 | 1.2 | |
Ruby cabernet | 2.5 | 1.9 | 1.4 | |
Peaches | ||||
Keisie | 3.5 | 1.3 | 2.3 | |
Kakemas | 3.5 | 1.3 | 2.3 | |
Oom Sarel | 2.5 | 0.9 | 1.6 | |
Sandvliet | 0.8 | 0.3 | 0.5 | |
Neethlings | 0.6 | 0.2 | 0.4 | |
Malherbes | 1.0 | 0.4 | 0.6 | |
Cascade | 2.1 | 0.8 | 1.4 | |
Plums | ||||
Souvenir | 2.8 | 1.0 | 1.8 | |
Harry Pickstone | 4.1 | 1.6 | 2.7 | |
Apricots | 6.9 | 2.6 | 4.5 | |
Table grapes | ||||
Crimson seedless | 4.1 | 2.7 | ||
Red globe | 5.2 | 3.4 | ||
Sugranineteen | 4.1 | 2.7 | ||
Thomson seedless | 4.7 | 3.1 | ||
Regal | 3.9 | 2.5 | ||
Dun-ben-hanna | 2.2 | 1.4 | ||
Autumn royal | 1.7 | 1.1 | ||
Sunred seedless | 1.7 | 1.1 | ||
Rain-fed crops | ||||
Winter cereals | 603 | |||
Pastures | 149 | |||
Vegetables | ||||
Potatoes | 25 |
Profitability is measured in IRR and NPV in capital budgeting, which means a figure is required on the investment value. This is expressed in capital requirement for each of the typical farms and is presented in Table 9 . The land prices and farm size are the main contributors to capital requirement. The typical farm for Paarl and Wellington shows a relatively higher requirement for fixed improvements due to packing and cooling facilities. The structure of the Swartland farm is completely different with much less infrastructure, but an added livestock component.
Capital investment requirement for the typical farm for each relatively homogeneous area in the Berg River area
. | Franschhoek . | Paarl . | Wellington . | Swartland . |
---|---|---|---|---|
Land | R 33,201,000 | R 33,251,000 | R 28,774,200 | R 51,000 000 |
Fixed improvements | R 10,531,583 | R 14,398,250 | R 14,317,250 | R 2,895,000 |
Movables | R 7,284,456 | R 7,753,456 | R 8,254,592 | R 5,487,641 |
Livestock | R 964,901 | |||
Total: | R 51,017,039 | R 55,402,706 | R 51,346,042 | R 60,347,542 |
. | Franschhoek . | Paarl . | Wellington . | Swartland . |
---|---|---|---|---|
Land | R 33,201,000 | R 33,251,000 | R 28,774,200 | R 51,000 000 |
Fixed improvements | R 10,531,583 | R 14,398,250 | R 14,317,250 | R 2,895,000 |
Movables | R 7,284,456 | R 7,753,456 | R 8,254,592 | R 5,487,641 |
Livestock | R 964,901 | |||
Total: | R 51,017,039 | R 55,402,706 | R 51,346,042 | R 60,347,542 |
Table 10 shows the expected IRR and NPV for the typical farm for each relatively homogenous area. This is for scenario 1 and thus assume normal export expectations based on sustained water availability and quality. An important consideration is that the profitability for the Franschhoek farm is calculated for grape production, in other words, for the farming side and not the cellar where wine making and value adding takes place. This value-added wine production is expected to have a much higher profitability.
The expected IRR and NPV for the typical farm of each relatively homogeneous area under normal export expectations (scenario 1)
. | Expected IRR % . | Expected NPV (R/farm) . |
---|---|---|
Franschhoek | 3.16% | R 5,158,880 |
Paarl | 9.17% | R 43,424,956 |
Wellington | 7.46% | R 29,545,468 |
Swartland | 5.01% | R 22,419,280 |
. | Expected IRR % . | Expected NPV (R/farm) . |
---|---|---|
Franschhoek | 3.16% | R 5,158,880 |
Paarl | 9.17% | R 43,424,956 |
Wellington | 7.46% | R 29,545,468 |
Swartland | 5.01% | R 22,419,280 |
Table 11 shows the expected profitability, namely, IRR and NPV, for the typical farms should water quantity or quality decrease to force producers to move from table grapes to wine grapes, and at lower yields. Quality will mostly affect market access and price. The price of red wine grapes at lower yields may increase for selected red wine cultivars ( Louw 2015 ). For the Swartland, the potato area was simply decreased. The effect on the profitability of wine grape farming will possibly be worse as the infrastructure change for the setting up of additional cellars was not incorporated in the model. Producers will also need to take up shares in cellars to be able to deliver wine grapes.
The effect of decreases in quantity or quality of irrigation water on the IRR and NPV for each relatively homogeneous area of the Berg River (scenario 2)
. | Expected IRR % . | Expected NPV (R/farm) . |
---|---|---|
Franschhoek | 2.08% | −R 2,184,242 |
Paarl | 4.84% | R 16,616,521 |
Wellington | 4.36% | R 11,909,278 |
Swartland | 4.32% | R 16,612,664 |
. | Expected IRR % . | Expected NPV (R/farm) . |
---|---|---|
Franschhoek | 2.08% | −R 2,184,242 |
Paarl | 4.84% | R 16,616,521 |
Wellington | 4.36% | R 11,909,278 |
Swartland | 4.32% | R 16,612,664 |
The third scenario entails the establishment of a water purification plant to remedy the water quality deterioration. This comes at a minimum cost of R1.5 m to the private producer. In this scenario, the infrastructure cost is added and electricity cost is increased. Table 12 shows the expected IRR and NPV for the cost of water purification scenario. In all probability, the Swartland producer will either stop producing potatoes or would produce them with lower water quality.
The IRR and NPV for the typical farm for each relatively homogeneous area with a water purification system introduced to mitigate the impact of decreasing water quality in the Berg River (scenario 3)
. | Expected IRR % . | Expected NPV (R/farm) . |
---|---|---|
Franschhoek | 2.87% | R 3,360,167 |
Paarl | 8.44% | R 40,463,301 |
Wellington | 6.74% | R 26,583,814 |
Swartland | 4.92% | R 26,963,101 |
. | Expected IRR % . | Expected NPV (R/farm) . |
---|---|---|
Franschhoek | 2.87% | R 3,360,167 |
Paarl | 8.44% | R 40,463,301 |
Wellington | 6.74% | R 26,583,814 |
Swartland | 4.92% | R 26,963,101 |
The results of this analysis show the significant reduction in both IRR and NPV due to a decrease in either water quantity or water quantity. As has been explored earlier, this decrease in water quality (and quantity) is most likely as a result of the pressures of rapid urbanisation and possible climate change impacts. The impact of decreasing water quality is to reduce the expected NPV by between 25% and 60%, which would have a serious negative impact on the local economy, as well as the potential for job creation and foreign earnings due to the export nature of the agriculture sector in this region. The fact that farms in the Franschoek area could experience a negative NPV due to declining water quality is particularly concerning as this would make agriculture essentially unviable in this region. This is significant not only for the agriculture sector, but also because the wine farms in this region are a significant contributor to the local (and international) tourism and are a significant source of job creation.
Under scenario 3, the NPV and IRR are returned to similar levels as for scenario 1. This would suggest that investing in on-farm water treatment to treat the irrigation water from the Berg River is a viable investment due to the threat of declining water quality. There are however alternative mitigation options that should also be considered as these could potentially be more cost-effective. These include piping water directly from the major dams to the indiviudal farms and not using the river as a conveyance channel, or alternatively finding solutions to the causes of the water quality risk. The latter solution, while preferable, has many challenges associated with the provision of basic services in a developing country context such as South Africa, which in itself is another wicked problem ( Govender 2016 ). As has been shown in terms of the analysis of the water quality data along the length of the Berg River, the importance of maintaining functioning stream ecosystems to reduce the water quality risks is also critical.
The provision of water services in a developing country context
The provision and management of services in a developing country context is challenging, particularly in areas where there are historic infrastructure backlogs and high unemployment. Govender (2016) considers that service delivery has complexity, uncertainty and power inequalities among stakeholders. Addressing historic legacies of poor service provision and the associated backlogs is a significant challenge for any government and can create a moral dilemma. OECD (2017) highlights that while ‘wicked problems’, as first defined by Rittel & Webber (1973) , may have a number of characteristics, they are particularly difficult for governments to manage as they cannot be ‘ solved only by partial or transactional solutions, but rather require concerted, adaptive and carefully stewarded approaches ’.
The management of water pollution in the Berg River is very complex and is considered to be a ‘wicked problem’ as it has multiple conflicting interests, affects multiple levels of government and impacts on the livelihoods of a significant number of people. Additionally, solutions for any one challenge are likely to impact or cause other challenges. Managing water quality is further complicated due to the Berg River crossing multiple administrative boundaries, a varied and diverse land use within the catchment, and not least, the complexities of water chemistry and the uncertainty of the sources of pollution once they reach the river. The results of this study however show that it is critical to address these challenges as the associated financial and economic impacts of a continued decline in water quality are significant.
Economic risks from declining water quality
Louw (2012) highlights the significant economic impact that poor water quality in the Berg River could have on the economy of the regional economy, resulting from poor sanitation service provision, agriculture and other practices. While Louw (2012) did not link their economic findings to specific water quality levels, he clearly demonstrated that if water quality continues to deteriorate to the point where international exports are affected, the economic consequences would be considerable. De Villiers (2007) indicates that from 1985 to 2005 there was a trend of increasing nutrient levels in the Berg River.
Managing current and future water quality risks
Up to a point, the receiving environment, such as a river, can provide ecosystem services by reducing the pollution loads through a combination of dilution and ecological processes. In the case of riparian systems, and in particular wetland areas, this can be significant ( Turpie et al . 2010 ). However, as discussed above, the Berg River is not expected to benefit from increased runoff volumes but is expected to see an increase in total population and levels of urbanisation. This will further add to the risks around water quality, water security and economic sustainability and so the risks to the agricultural sector might therefore increase. The local (South African) context further adds to the risks of the complexities of service provision against an historical background whereby, since a system previously existed of limiting the access of indigenous people to the urban areas, there has been an influx of people since the early 1990s.
This urban population increase has created a backlog and added to the difficulties of delivering services to those who historically do not have them while concurrently meeting the demand for services from an increasing and urbanising population. The management of water quality in such a catchment is a complex, but important issue, owing to the diversity in types and sources of pollution. The management of pollution in a catchment where there are multiple municipalities involved, and pollution control measures have been set by a higher authority – such as the DWS – is particularly challenging. However, even within a single municipality where different departments have competing objectives, there can be significant challenges to implementation ( Fisher-Jeffes et al . 2012 ).
Urbanisation and climate change are likely two of the most defining occurrences of the 20th and 21st centuries. The Berg River in South Africa, like many other catchments, particularly in the developing world, falls within a catchment that is experiencing increasing levels of urbanisation, the impacts of climate change, the ‘wicked problem’ of service delivery to the historically disadvantaged within a developing country, persistent infrastructure backlogs, and where high unemployment is prevalent. Building on the previous investigations looking at water quality in the Berg River, it is evident that the state of the river requires management, and at places along the river is potentially unsuitable for irrigation.
The results of this study indicate that while agriculture may, and it is not certain, be reducing its impact on the river, the impact of urbanisation is undoubtedly increasing. The impact of urbanisation is primarily from three sources: effluent discharges from WWTW, drainage stormwater from informal settlements, and stormwater from formal fully serviced settlements. Added to this is the threat of climate change. The whole-farm costing in this paper has shown that the economic consequences of deteriorating water quality in the Berg River is potentially quite severe. The alternative, considered in this paper, was for the agricultural sector to protect the industry by investing in treating the water prior to irrigation. This too would have significant, albeit not as severe, economic consequences for the agricultural sector and while it is a possible option for mitigation, it is critical to explore other options such as improved provision of basic services or the rehabilitation and protection of natural systems that have other potential benefits.
The Berg River is not unique in that many catchments face similar challenges, particularly in the developing world. As a result, the lesson learnt from this study, and in general, the lessons learnt from the Cape Town drought crisis, are globally relevant. The intention of this study was to indicate the importance of making investments into mitigating the significant impact of declining water quality, particularly in terms of investment in the upgrading of treatment plants and the protection of ecological infrastructure.
Funding for this study was provided by the Department of Environmental Affairs and Development Planning (DEA&DP). The maps were produced by the Sub-Directorate Spatial Information Management, DEA&DP, Western Cape Government. Census data were supplied by Statistics South Africa with acknowledgement to Development Planning Intelligence Management and Research (DEA&DP). Pollution monitoring data were provided by the DEA&DP as part of the Berg River Improvement Project (BRIP) as well as from the national Department of Water and Sanitation (DWS) of South Africa.
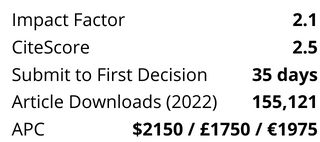
Affiliations
- EISSN 2616-6518
- Open Access
- Collections
- Subscriptions
- Subscribe to Open
- Editorial Services
- Rights and Permissions
- Sign Up for Our Mailing List
- IWA Publishing
- Republic – Export Building, Units 1.04 & 1.05
- 1 Clove Crescent
- London, E14 2BA, UK
- Telephone: +44 208 054 8208
- Fax: +44 207 654 5555
- IWAPublishing.com
- IWA-network.org
- IWA-connect.org
- Cookie Policy
- Terms & Conditions
- Get Adobe Acrobat Reader
- ©Copyright 2024 IWA Publishing
This Feature Is Available To Subscribers Only
Sign In or Create an Account
Academia.edu no longer supports Internet Explorer.
To browse Academia.edu and the wider internet faster and more securely, please take a few seconds to upgrade your browser .
Enter the email address you signed up with and we'll email you a reset link.
- We're Hiring!
- Help Center
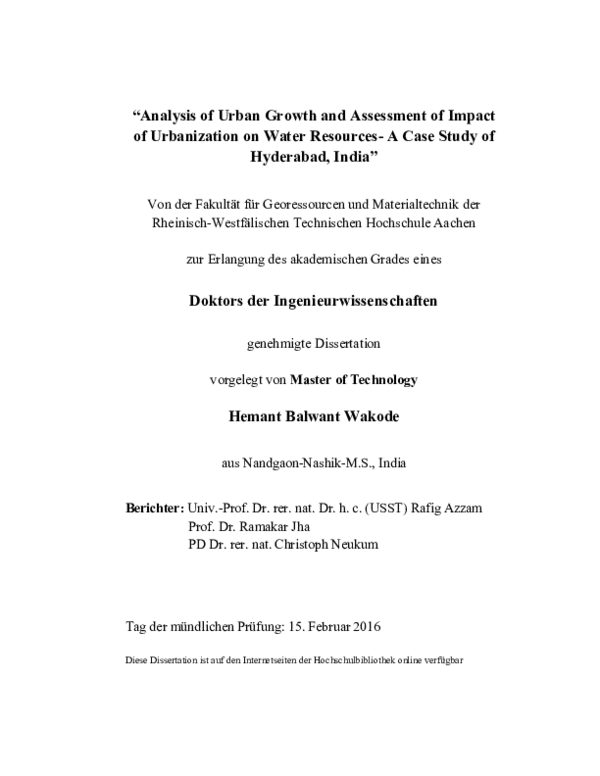
Analysis of urban growth and assessment of impact of urbanization on water resources : a case study of Hyderabad, India

Related Papers
Water Science & Technology
Abdul hameed Al Obaidy
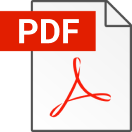
Journal of Environment …
Vaibhav Garg
Irrigation and Drainage
Daniel Van Rooijen
Editor IJER
Md. Zamal Uddin
shaina Beegam
As long as human being continues to exists, urbanization continues. Urbanization has a direct effect on environment which in turn effects the variations in hydrology. It may results from changes in the land use pattern to built-up areas resulting in runoff which ultimately leads to flood. Most of the studies revealed that land use pattern has drastically changed in which the built up area increases year after year. The land use land cover changes can be identified using GIS. Many researchers have found that urbanization has an impact on hydrological parameters such as runoff volume, discharge in drains, infiltration, interception, evapotranspiration etc. An attempt has been made to consolidate the review of literature related to impact of urbanization on hydrological parameters.
Dr. Pradip Saymote
Veena Srinivasan
World Environmental and Water Resources Congress 2012
Bhagu Chahar , Sridebi Basu
Nupoor Prasad
Lucknow is the capital city of India's most populous state Uttar Pradesh. Urban growth currently is largely on par with other cities of similar size, and is anticipated to be slightly faster in the immediate future. The better economic prospect has lead to an increase in the urban population of the city during last few years. It has lead to large-scale urban sprawl and the inherent distinctiveness of hydrological environment is being neglected in urban planning. With the expansion of the urban sprawl and the increasing population, there has been a surmounting pressure on a) natural and built drainage systems b) surface/subsurface hydrological storage units, of Lucknow. The anthropogenic factors have also contributed to the presence of heavy metals in the hydrological units of the city. Upto now the regeneration of water by nature kept the surface and subsurface water ecosystem pure, satisfying the urban and peri-urban requirements. But during last few years, increasing demands from new urban extensions as well as intense agriculture and irrigation practices in surrounding rural areas have stressed the hydrological cycle considerably, raising questions over the sustainability of the water resources in the city. This paper attempts to highlight some important environmental criteria and propose methodology to achieve it, during the design of master plan with a focus on surface and subsurface water integrity of Lucknow building upon city's natural and built environments. Changes in land use and land cover are estimated with respect to urban sprawl from year 1973 and 2005 based upon Survey of India toposheets and satellite data of IRS -1D LISS III PAN Sharpened. Drainage network map is overlaid with land use patterns drawing implications on water environment.
Loading Preview
Sorry, preview is currently unavailable. You can download the paper by clicking the button above.
RELATED PAPERS
Dr. P.A. Saymote Asst. Professor Department of Geography
Journal of Global Resources
PRERNA JASUJA
The Urban Book Series
BHAVANA UMRIKAR
International Journal of Environmental Research
Shakeel Ahmed
Asian Journal of Water, Environment and Pollution
sana mukhtar
Harsh Shukla
Environmental Modeling & Assessment
Biju George
Arunangshu Mukherjee
Jesse Stimson
International Journal of Advancements in Research & Technology
manikya reddy
juan penagos
Dean School of Architecture
Hydrological Processes
Mingfu Guan
Journal of Hydrology
Architecture Engineering and Science
Dr. Mohammad Arif Kamal
Siddharth Bhatia
Prof. Asit K . Biswas
Environmental Monitoring and Assessment
Jivesh A Tambe , Pradeep Naik
International Journal of Environmental Sciences & Natural Resources
Violeta Gesovska
Responding to urban water challenges in Southeast Asia. Introducing polycentric management approaches to create resilient, water-sensitive cities
Bernd Gutterer
Ankit Patel
GRD JOURNALS
ajer research
RELATED TOPICS
- We're Hiring!
- Help Center
- Find new research papers in:
- Health Sciences
- Earth Sciences
- Cognitive Science
- Mathematics
- Computer Science
- Academia ©2024
- International Journal of Engineering Research & Technology (IJERT)
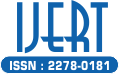
- Mission & Scope
- Editorial Board
- Peer-Review Policy
- Publication Ethics Policy
- Journal Policies
- Join as Reviewer
- Conference Partners
- Call for Papers
- Journal Statistics – 2023-2024
- Submit Manuscript
- Journal Charges (APC)
- Register as Volunteer
- Upcoming Conferences
- CONFERENCE PROCEEDINGS
- Thesis Archive
- Thesis Publication FAQs
- Thesis Publication Charges
- Author Login
- Reviewer Login
Volume 10, Issue 03 (March 2021)
A sustainable model of urbanization for indian cities, a case study of new delhi.

- Article Download / Views: 6,494
- Authors : Madhuri Agarwal , Ruchi , Farheen Alam Fakhr , Kusum Choudhary
- Paper ID : IJERTV10IS030022
- Volume & Issue : Volume 10, Issue 03 (March 2021)
- Published (First Online): 12-03-2021
- ISSN (Online) : 2278-0181
- Publisher Name : IJERT

Ar. Madhuri Agarwal1*, Ar.Ruchi2*, Ar. Farheen Alam Fakhr3* , Ar. Kusum Choudhary4*
1Gautam Buddha University, Greater Noida,
2Galgotias University, Greater Noida, 3CSIR-CBRI, Roorkee, India,
4 Galgotias University, Greater Noida.
Abstract – Today Urbanization is the most echoing word for all the cities around the globe but in simple terms Urbanization has been described as a consequence of population shift from less utilitarian areas to high utilitarian areas. The process of urbanization is directly proportional to other trends e.g., modernization, industrialization, technological advancement, infrastructure, sociological transformations, economy, planning policies and public health etc. Urbanization impacts climate, Land use pattern and transportation on a larger scale, however the debates of decades are yet to summarize the pros and cons of it. Urbanization is not just a modern threshold, but it is a phenomenon of transferring and redefining the social, cultural and historical roots of human on a universal scale. Whereas the rural tradition is the most effected aspect influenced by urban culture, the sustainable growth of urban cities is only possible when the planner would start working on synchronized policies for both the rural and urban transformations. In India many rural habitants migrate for employment and better lifestyle but the glint of urbanization fades on factual ground where a city fails to provide even the basic necessities to a human e.g. food, shelter, education and employment. The haphazard and unplanned growth of metropolitan cities has resulted in urban sprawl and over- densification. The intermural city stresses and migration from outside of the city are the two main factors that determine the positive or negative impacts of urbanization. The urbanization story of Indian cities also comes with its share of complex issues related to housing, Pollution, climate change along with inadequate provision for social and physical infrastructure. The emerging cities are located in developing countries that are experiencing rapid economic growth. In India, they are referred to as Tier II (14 million people) or Tier III (0.51 million) cities. Indias urban areas currently house about 377 million people and will have to accommodate close to 200 million more by 2030 as the countrys economic emphasis shifts from agriculture to manufacturing and services. Much of Indias growth is currently taking place on the fringes of cities. It is unplanned and without sufficient infrastructure and services. In 2010, urban pollution caused more than 620,000 premature deaths (a more than six-fold increase in a decade). Environmental degradation is costing India about US$80 billion annually 5.7% of GDP. While these are enormous challenges, Indias emerging cities are critical to the countrys economy, being expected to contribute up to 75% of national GDP by 2020. It is estimated that urbanization will generate 6 billion urban dwellers by 2050. Cities are set to be subjected to climate change.
Cities worldwide are increasing enormously plans to transform to the impact of urbanization. Policymakers and urban planners have increasingly become interested in understanding the concepts of urban resilience, vulnerability, and adaptation. These plans will have significant implications for urban dwellers as they are prone to restructure and reconfigure urban infrastructure, services and decision-making processes. This paper aims to focus on such urban issues followed by solutions which complement the principles of
Green Urbanization and sustainable development. The paper emphasizes on Urban Housing, as the shelter is the most basic but still neglected aspect of metropolitan cities, where a home becomes a dream and flyovers becomes the new normal of a home, acquiring a large part migrating population. The shortage of affordable housing entwined with rapid urbanization has resulted in informal settlements e.g. slum dwellings, unauthorized colonies and squatters etc. The working population also struggles to find accommodation in proximity to their place of employment and hence mass public transport systems are being stretched to the peripheral areas of the cities as they expand. As Indian metropolitan cities embark on the next phase of development driven by urbanization, we need to adopt more sustainable urban development practices that meet the demands and aspirations of urban lifestyle. The key focus of this paper is to develop a sustainable model of urbanization for Indian cities in general by contemplating city New Delhi as a benchmark for urban development, policies and strategies.
Keywords -Urbanization; Developing World; Indian Cities Green Urbanism; Sustainability; Shelter; Modern Housing
INTRODUCTION
India has recognized gigantic industrialization, motorization, and urbanization at once in a very short span of time, largely navigated by globalization, technological advancement, and increased world economic cooperation that has made cities thrive in multi- dimensional ways.
As per the records from the last one-decade India has the world's secondlargest urban system, after China. It is the world's largest Democracy along with the very fastest- growing nations. This makes the Indian metropolitan cities, urban centres and developing urban infrastructures more exposed and highly vulnerable to aggressive urbanization which play the twin roles of both the advantage and adversity at certain point of time.
According to the report of McKinsey Global Institute. (2010) & UN DESA 2014:
The expected growth in urban population is from 410 million in 2014 to 814 million by 2050.
As per the census report, 2011, 31% population resides in urban areas and urban centers.
The projections for 2025 predicts that 46% of Indian population will reside in cities with more than 1 million population and large densities.
By the year 2030, the number of cities will grow in numbers from 42 to 68, which will have the population more than 1 million (stated by McKinsey, 2010)
According to World Bank some facts for Urbanization in India are:
Figure 1(Source: World Bank, 2011)
The reports further specify that the following measurements are required for India to achieve and survive along with the futuristic urban growth and requirements;
A capital investment of US 1.2$ trillion
roads to be paved (Approx. 2.5 billion square meters)
700-900 million square meters of commercial and residential space to be provided.
7,400 kilometers of subways and transportation to be constructed.
Development of integrated development plans by state and center authorities.
As population or density grows in an area or a community, the city's boundaries expand (in terms of space, services and infrastructure) to accommodate the growth and facilitate the living conditions; this expansion is called sprawling, a very natural yet alarming phenomenon in terms of planning and framing policies. Nowadays we live in a built environment that tends to suffer from the spatial adverse effects of hurriedly administered technical overdose. These technological innovations and large-scale migration of people from rural areas to urban areas, which further results in degradation of the urban environment and degeneration of their mother(rural) environment. The enormous amount of land seized by urban sprawl, sometimes on the name of land acquisition and sometimes
on the name of., which are nothing but distinct processes of
environmental degradation. A region's structure of land use / land cover is the product of natural and socio-economic aspects and its usage over a period of time and as per the indigenous character of the space, when these spaces are exploited to cater a chunk of population and capitalistic benefits, degeneration phase gets initiated by certain anthropogenic activities. Activities in land use are a big issue and challenge for city/county planners, policy makers and for ecologist in planning an environmentally friendly, sustainable and growth in economicy. Delhi is one of the many megacities struggling with rapid urbanization and colossal levels of industrial, residential and transportation challenges along with
other challenges e.g., pollution, infrastructure and environment etc.
As in line with the statements from numerous cabinets of the United Nations, Delhi is predicted to end up the primary and maximum populous town in the global round 2028, and via 2050 India is anticipated to add 416 million city citizens allotted in improved numbers of urban towns.
Delhi's projected population size in 2028 is roughly 37.2 million, surpassing 36.8 million populations in Tokyo.
The important thing reason for this upward thrust within the Delhi population is due to the migration of people from rural areas looking for employment for improved living standards and inter- town tensions. Since the Delhi Government has no strategies to accommodate these people, this leads to a haphazard urbanization of capital that nullifies the sustainability principles.
This paper aims to focus on such issues within Urban India (mostly faced by Indian metropolitan cities) and propose solutions that align with the principles and concepts of Green Urbanism. The emphasis is given on urban housing, because access to an affordable home is one of the biggest challenges facing the migrant population coming to Indian cities. The shortage of affordable housing coupled with rapid urbanization has resulted in the Indian cities creating slums and unauthorized informal settlements. The working population is also struggling to find accommodation near their place of work, and as they expand, they are stretching mass public transport systems to the peripheral areas of the cities. Cities play an essential role in bringing about economic growth and prosperity, in total a house
of ones own is still a dream for many migrant and non-migrant
citizens. The sustainability of cities depends on large part on their physical, social and institutional infrastructure and development plans and processes adopted.
A very transcendentalist and reforming minister, Theodore Parker once stated that Cities acts as fireplaces of ancient civilization, Radiating light into a total dark.
As Indian cities embark on the next stage of urbanization-driven growth, we need to follow more sustainable urban development practices that meet our society's demands and expectations, contributing to a better quality of life but without any compromise and forsaken futuristic needs.
URBANIZATION IN INDIA
2.1. Physical development in urban areas
Urbanization or city float is the bodily development in urban regions due to worldwide exchange or the increasing percentage of the full populace in cities. Urbanization can describe a selected nation at a given time, i.e. The percentage of the overall populace or region in towns, or the time period may describe the growth of that percentage in time and the word urbanization can represent
the urban stage as compared to the populace as a whole, or it could represent the price at which the urban share is growing in a rustic. The urban population of India currently comprises around 30% of its total population. In line with the mc Kinsey international
institute file, Indias city population will boom to 590 million
through 2030 which is sort of double the size of the entire population in the USA. With the aid of the use of the identical 12 months., India will even have sixty-eight towns with greater than 1 million human beings in each, 13 towns with more than four million and 6 megacities with a population that exceed 10 million inhabitants. Furthermore, the cities are the drivers of the increase of the Indian economic system that is expected to be five instances huge in 2030. This creates growth in hard work-energy with 270 million human beings, with 70% coming from urban employment.
Urbanization in India is taking place at a speedier rate. In keeping with the 1901 census, the populace dwelling in city areas in India turned into 11.4%. In step with the 2001 census, this figure expanded to 28. 53% and crossed 30% as in keeping with the 2011 census, at 31.16%. As in keeping with a world populace record of 2007 through a UN kingdom, through 2030, 41% of the United States of America's populace is expected to be living in city towns with predominant challenges for survival. As according to the
World Banks prediction, India, alongside other nations e.g.,
china, Indonesia, Nigeria, and the us, will lead the world's essential city populace efflux through 2050.
Figure 2: Source: Statista (Statistics Portal)
Figure 3:Mckinsey Report on Urban India 2030
Figure 4: Urban India 1951 (Source: IIHS, Analysis of Census Data 1951, Satellite Map, Google Inc.,)
Figure 5 : Urban India 2031 (Source: IIHS, Analysis based on census of India, Satellite Map, Google Inc.)
Figure 6: Ten Largest Cities (Source: IIHS, Analysis of Census 2011 (Built Up Area), Census 2011 (Population), Planning Commission 2011 (DPP Estimates 2005-2006)
Figure 7: Distribution of Indias Population by settlement size (Urban and Rural):1951-2011(Source: IIHS Analysis based on Census 1951-2011)
Urbanization Issues and Challenges for Indian
The unplanned urbanization due to migration, coins toss various issues and problems before India as a developing country. Some of these urban sprawls, housing, squatter settlements, building greenhouse gases (e.g., Carbon dioxide, methane) climate change, pollution, infrastructure, mobility, water etc. The main considerable challenge of today in front of the visionaries is as
how the planners and technical development team can incorporate various concepts of Green Urbanism to make sustainable urbanization possible.
Urban Sprawl
In 1958, a Canadian economist, William Whyte coined an alien term as Urban sprawl, a term that has been used intensely by
later city planners and visionaries. City sprawl is defined because the deliberate/unplanned growth of a metropolitan region. The tendencies in urban sprawl are of miscellaneous sorts e.g., Land use in remote places on/across the urban fringe, gradual filling-in of the intervening spaces with similar uses, and seizing of land in step with the suitable location and availability of resources. Rapid urbanization is resulting in disorganized and unplanned towns and cities, lacking proper maintenance and strategic plans. Urban Sprawl may take various tangible/intangible forms and may boost up residential projects of high-income people seeking larger housing sites along with business activities such as
manufacturing, offices, less planned downtown areas and pop up houses.
Figure 8: Urban Sprawl in Delhi over last 38 years (Source: Earth Interactions 2016)
The pressure of an ever-developing population will become a burden at the constrained civic amenities which might be certainly collapsing with time and becomes a burden on the limited civic amenities which are virtually collapsing with time and public utilization, Hence the urban sprawl can be one of the reasons for shifting central business districts (CBDs) from one corner to another impacting the social and economic neighbourhood of a city. The expertise of growth dynamics of urban agglomerations is crucial to strategize the sustainable city developmetal making plans for futuristic cities.
Figure 9: NCR and Outgrowths in 2018, Senital S2A Mosaic, Captured 14 Feb 2018 (Source: Shrobona Karkun,Temple University
as per the basic fundamental right to shelter, Article 19( with Article 21, so that the problems of slums,
In Indian context, the major challenge for the government is to provide housing for current Urban Population and provide provisions for the projected urban population along with other basic necessities. In India we have many metro cities as well as tier two cities which are growing very rapidly because of endogenous and exogenous tensions of a city e.g., migration from surrounding areas, population shift, employment and infrastructure etc. Urbanization has many effects on the city structure both in tangible and intangible aspects. The urban escalating population has to be properly accommodated in the city
Figure 10: Worlds Largest Built-Up Urban Areas (Source: new geography)
Squatter Settlements
A squatter settlement is defined as a residential area in an urban locality inhabited by a very poor population with no access to tenured land of their own, and hence "squat" on a v acant land without any proper registration of land and ownership, either
private or public, The population residing in squatter settlement is consider as the most exploited citizens, used as voter banks without provisions of any extended facilities and with least liveability index, these people lacks the basic provisions for
NCR and Outgrowths in 2018,Senital S2A Mosaic,Captured 14 Feb 2018 ( Source: ShrobonaKarkun,Temple University)
1)(e) read squatters,
electricity, drainage, education and employment etc. .
unauthorized construction and haphazard development of fringe areas will be rectified in most of the Indian cities. The land value and cost of houses has made it nearly impossible for urban middle class to afford a shelter under their budget. Majority of lower earnings businesses are residing in chook cage sized congested residences, transit shelters, ren-baseras (night time shelters), and even the juggis under flyovers and on footpaths. Those forms of shelters are without proper power, ventilation, lighting fixtures, water supply, sewage system, and many others.
For a case, in Delhi, the modern-day predicted scarcity is of 25 million residing devices in the coming a long time. The efficient ways should be channelized by the authorities to fulfil the future requirements without neglecting with environmental factors and resources.
Figure 11: Indias Population till 2018(Source: Trading Economics)
The Squatter settlements are an inevitable phenomenon in an
urban city. It is further stated that by 2019, the expected slum population in India would be 105 billion, if the current situation is neglected and no required actions are taken by the government and other planning authorities. In a latest report by National Sample Survey Office (NSSO), the scarcity of houses can be easily identified where the government fails to cater the demand of the population. Census 2011 found that there are 40,309 identified major slum zones in India, constituting 37% of the total population. Countrywide strategies to squatter settlements have normally converted from terrible views (together with involuntary resettlement, forced eviction, benign forget about, and so forth.) to extra superb perspectives (which include, permitting and rights-based regulations, self-assist and in situ upgrading,). urban slums are growing at faster rate than ever expected, stated by The Challenge of Slums, a Global Report on Human Settlement (UN-Habitat), 2003. One billion people are living out their days in the squalor of a slum, which is one out of every three city dwellers and a sixth of the worlds population. The statement reveals that the numbers can be doubled within 30 years if radical changes are not adopted inclusively by the planners. The predicted addition counts around 300 million new urban residents by 2050 (World cities Report 2016&EmergingFutures report by UN Habitat). According to Census 2011, Delhi has 22 slum towns with total population of around 17, 85, 390.
Figure 13: Source: The graph shows the expected increase of the worlds squatter population by 2050 (Source: UNCHS HABITAT)
Environmental Concern
Urbanization in India is greater or much less developing negative impact on surroundings due to which issues like land lack of confidence, worsening water excellent, excessive air pollutants, noise, and disposal of waste are happening. One hassle is to combine land- and water use planning to offer meals and water security within the destiny (UNEP 1999). Due to the poor impact at the environment and weather trade problems like the creation of urban warmness island, modifications in air excellent index (AQI) and styles of precipitation have been taking place very often. As a result of impact of urbanisation, land resources problems like soil erosion, water table contamination and vegetative quality decrement etc. has been taking place. Also, we are facing problems of scarcity of water resources for domestic purpose and have been consistently compromising with the quality of water.
Figure 14: Emissions of CO2 and other greenhouse gases in India in 2016 (Source Netherlands Environmental Assessment Agency)
One of the major environmental concern for the environmentalist is the emission of various harmful/reactive gases with inclusion of CO2.An ecologist and geographer anastasiasvirejeva- hopkinset al. (2004) has concluded in a research that approximately more than 90% carbon emissions are produced in urban cities due to anthropogenic interventions. In case of India, the transportation sector is contributing immensely in to the green houses emission because of diesel consumption. It is further increasing due to rise in population, traffic and excessive modes for mobility. A dynamic shift from slow moving vehicles to fast moving vehicles has impacted drastically on the urban environment. Apart from it Industrial sector and waste sector also contributes in CO2 emission.
A case of Delhi: The urbanization of Delhi is observed from the beginning of the 20th Century. In 1901, 53% of the total population of Delhi was considered to urban category. The current alarm is about the country of human health within the swiftly growing metropolis of Delhi in addition to its deteriorating surroundings. Whilst the citys population has grown from 1.74 million (1951) to 16.75 million (2011) at the imply place of 1,483 sq. Km of land, it counts the density of 11,297 men and women consistent with square km. In response to the desires, there are large vehicularisation and land use alterations. Delhi ridge woodland cowl has done not meet discount targets for greenhouse gases emission in the Delhi city because of constant concrete jungle sprawl over the periods and discount in the inexperienced cover.
The traffic structure of metropolitan cities of India (e.g., Delhi, Mumbai, Kolkata, Chennai, Bangalore and Hyderabad) illustrates a substantial shift from the share of slow-moving vehicles to fast moving vehicles and public transport to private transport. The vehicle manufacturing and export-import rate has been increasing by 15-20 per cent each year. As per a recent media report (T.O.I.), Delhi is adding 965 vehicles omits transportation network every day while Bangalore is adding 500vehicles. Synchronously, more than 40 other metropolitan cities (with human population more than1million) are accounted for 35% of the vehicular population of the country. Further, 25% of the total energy (of which 98 percent comes from oil) is consumed by road/Transportation sector. Vehicles in mega-cities are estimated 70% responsible for CO2 mission. The extracted pollutants from automobile sectors are largely responsible forair born respiratory diseases and other air related diseases including lung cancer, asthma, etc.
The water crisis in India is often ascribed to the urbanization, industrialization, and human waste flowing into water sources and polluting groundwater, in addition to corruption at exclusive tiers that postpone diverse tactics and responsibilities. Water shortage in India is predicted to irritate as the general populace is anticipated to boom to at least 1.6 billion by using the year 2050. As per reports, India only possesses 4% of the worlds total fresh water. If the modern-day rate of water demand keeps, about sector of the destiny call for water could be backordered by 2030, stated with the aid of the committee on restructuring the significant water fee (cwc) and the crucial ground water board (cgwb) in 2016. Water tables are depriving in most parts of India. The contamination content of water includes various minerals e.g., arsenic, fluoride, mercury and uranium in varying content ratios, causing diseases borne by contaminated water etc.
Climate trade poses more excessive demanding situations e.g., Rates of rainfall and evapotranspiration accentuate the effects of floods and droughts. 80% of India's drinking water, nearly -thirds of irrigation desires, and 11% of the agricultural water deliver relies upon on groundwater. Meanwhile 60% of India's districts face groundwater over-exploitation. The sector bank spotlight the predicaments that the united states are going through from the past 5 many years:
163 Million Indians lacks access to safe and drinkable water.
210 Million Indians lacks access to improved sanitation.
21% of communicable diseases are due to unsafe water.
Each day in India, 500 children under the age of five die from diarrhea.
River Pollution
The rivers in India are noticeably polluted and taken into consideration risky by technical and medical requirements. As a carrier and main source of water in northern India Yamuna, Ganga, and Sabarmati is a lethal blend of pollution each risky, toxic, and natural. A research paper from IIT, Delhi, has develop a research project on a bacterium that the river Yamuna has harbored known as antibiotic-resistant or priority pathogens. These multidrug-resistant bacteria pose greatest threat to human health. Apart from water pollution the government policies should also be focused on water availability in each region and village. Several projects are in progress with an aim to provide water in the most needed areas in India. But this long-term commitment is slow. Common experience practices and training competencies will help in compensating the harm done to groundwater resources. As per the news, many skilled farmers are updating to modern technologies e.g. Irrigation techniques, drip irrigation, rainwater harvesting, Effective steps in stemming the lack of freshwater resources and so on. The collective practices and inclusive planning in the mild of inexperienced urbanism will cause frame present-day sanitation rules a good way to assist in both preserving and wisely make use of water assets.
Trash Disposal
Urbanization results in superior municipal stable waste (msw) era. Unscientific and non-organic managing of msw deteriorates the city environment and causes fitness hazards to other living organisms. As in step with the authority reports, 12 million tons of inert wastes are generated in India yearly. The generation of municipal solid waste (MSW)shows a graph directly proportional to the economic condition, urbanization, and rapid growth of population. The problem is in addition aggravated by the lack of policy controls; monetary aids in addition to human sources, educated in solid waste control practices within the sphere of series, transportation, processing, and very last disposal. While aspects like recycling, reuse, and restoration of the solid waste are grossly demanded however disorganized in most cases. Terrible sewage collection and absence of connectivity between drains and sewage treatment vegetation have worsened the state of affairs, noted the paper published in the Journal of Environmental Chemical Engineering.
The principal issue of trash disposal is exacerbating in urban areas due to the rapid populace boom, coupled with economic growth that encourages the intake of goods and waste technology. In keeping with the brand new cpcb document, in 2016, India produced a few fifty-two million heaps of waste each yr, or more or less 0.144 million heaps in step with day, of which roughly 23 percentage is processed and brought to landfills or disposed of the
use of authentic biological techniques and technologies.
Figure 18: MSWM practices in selected Indian cities (Kumaret al., 2009).
Figure 19: Prediction Plot for MSW generation, land requirement, and population from 2001 to 2051. (Source: Cogent Environmental Science)
The contemporary schooling device emphasizes minimizing the stable waste era by using adopting the policy of 4rs. That is
refuse, reuse, recycle, and reduce. The modern-day guidelines of MSWM are very uncompromising, ensuring a proper MSWM gadget. However lamentably, there may be a large hole on the ground between policy and implementation.
Consequently, there is a pressing want to bridge up these gaps. The authorities need to also emphasize the involvement of
humans and public session together with the personal region via NGOs and PPP (Public Private Partnership) projects, House to house collection should also be promoted. These types of steps would help to improve the efficiency of MSWM, working structures of different authorities, Public Awareness and public participation.
SOLUTIONS THAT ALIGN WITH PRINCIPLES OF GREEN URBANISM TO OVERCOME URBAN ISSUES AND CHALLENGES
Futuristic cities and urban dynamics frames perceptions about bringing new infrastructure, facilities and life style with social cohesion and economic sustenance, coping up with all urban demands and dilemmas but cities of tomorrow do not reside in other zolo from cities of today, the basis for futuristic urban cities
will only form by the strategies picked to tackle todays
challenges. The major challenge is that concerns exists but in isolation just like the strategies. Todays need is not just to make
a comprehensive strategic plan but an interlinked / integrated plan, to cater all the levels of pyramid.
Green Urbanism is an interdisciplinary term, it requires the collaboration of various social and professional threads e.g., Panorama architects, engineers, urban planners, ecologists, transport planners, physicists, psychologists, sociologists, economists, and other experts contributing to building a country, further to architects and concrete designers.
Green urbanism minimizes each mode to utilize strength, water, and different resources at each level, along with the embodied electricity within the extraction and transportation of constructing substances, their fabrication, incorporating the material into the constructing and, in the end, the benefit and price of their recycling whilst a character buildings existence is over. These days, the urban and architectural design also has to take into consideration whilst intervening energy-green strategies into building production, preservation, and modifications in its use inclusive of the number one energy use for its operation, along with lighting fixtures, heating, and cooling, and so forth.
The major principles of green urbanism include striple-zero frameworks (triple-bottom line) of:
zero fossil-fuel energy
zero gas emissions (aiming for low-to-no-carbon emissions).
Printed Book
Amritamasebi, R. Orloff, M. Wahba, . and Altman, A. (2016). Regenerating Urban Land. USA: World Bank Group, p. 479
Zeisel, J. (1984). Inquiry by Design: Tools for Environment-Behaviour Research. UK: Cambridge University Press, p. 241
Research Papers
Topcu, M. (2009). Accessibility Effect on Land Values. Selcuk University, Faculty of Engineering and Arhitecture, Department of Urban and Regional Planning. [online] p. 6, Available at: http://www.academicjournals.org/journal/SRE/article-full-text- pdf/AD2B05619110 [Accessed 21 Sep. 2017].
Bhan, G. 2009. This is no longer the city I once knew. Evictions, the
urban poor and the right to the city in millennial Delhi. Environment and Urbanization 21(1):127-142. http:// dx.doi.org/10.1177/0956247809103009
Padmanabhamurty, B., Bahl, H.D., 1984. Isothermal and isohyetal patterns of delhi as a sequel of urbanization, Mausam, 33, 4
Padmanabhamurty, B., Bahl, H.D., 1982. Some physical features of heat and humidity islands at Delhi Isothermal and isohyetal patterns of Delhi as a sequel of urbanization, Mausam, 33, 211-216.
Delhi Pollution Control Committee. (2015). Annual Review Report for the year 2014-15. Delhi. East Delhi Municipal Corporation. (2015). Annual Report for the year 2014-15. Delhi.
TERI (2002): Performance Measurement of Pilot Cities Tata Energy Research Institute, New Delhi, India.
https://www.thehindu.com/sci-tech/energy-and- environment/pathogens-listed-as-critical-by-who-found-in-river- (yamuna/article32315382.ece?homepage=true&utm_campaign=socia lflow)
Leave a Reply
You must be logged in to post a comment.
Information
- Author Services
Initiatives
You are accessing a machine-readable page. In order to be human-readable, please install an RSS reader.
All articles published by MDPI are made immediately available worldwide under an open access license. No special permission is required to reuse all or part of the article published by MDPI, including figures and tables. For articles published under an open access Creative Common CC BY license, any part of the article may be reused without permission provided that the original article is clearly cited. For more information, please refer to https://www.mdpi.com/openaccess .
Feature papers represent the most advanced research with significant potential for high impact in the field. A Feature Paper should be a substantial original Article that involves several techniques or approaches, provides an outlook for future research directions and describes possible research applications.
Feature papers are submitted upon individual invitation or recommendation by the scientific editors and must receive positive feedback from the reviewers.
Editor’s Choice articles are based on recommendations by the scientific editors of MDPI journals from around the world. Editors select a small number of articles recently published in the journal that they believe will be particularly interesting to readers, or important in the respective research area. The aim is to provide a snapshot of some of the most exciting work published in the various research areas of the journal.
Original Submission Date Received: .
- Active Journals
- Find a Journal
- Proceedings Series
- For Authors
- For Reviewers
- For Editors
- For Librarians
- For Publishers
- For Societies
- For Conference Organizers
- Open Access Policy
- Institutional Open Access Program
- Special Issues Guidelines
- Editorial Process
- Research and Publication Ethics
- Article Processing Charges
- Testimonials
- Preprints.org
- SciProfiles
- Encyclopedia
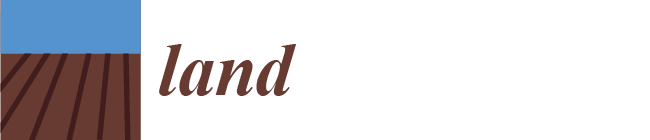
Article Menu
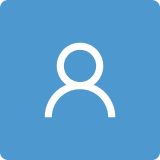
- Subscribe SciFeed
- Recommended Articles
- Google Scholar
- on Google Scholar
- Table of Contents
Find support for a specific problem in the support section of our website.
Please let us know what you think of our products and services.
Visit our dedicated information section to learn more about MDPI.
JSmol Viewer
Social media users’ visual and emotional preferences of internet-famous sites in urban riverfront public spaces: a case study in changsha, china.
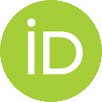
Share and Cite
Huang, Y.; Zheng, B. Social Media Users’ Visual and Emotional Preferences of Internet-Famous Sites in Urban Riverfront Public Spaces: A Case Study in Changsha, China. Land 2024 , 13 , 930. https://doi.org/10.3390/land13070930
Huang Y, Zheng B. Social Media Users’ Visual and Emotional Preferences of Internet-Famous Sites in Urban Riverfront Public Spaces: A Case Study in Changsha, China. Land . 2024; 13(7):930. https://doi.org/10.3390/land13070930
Huang, Yuanyuan, and Bohong Zheng. 2024. "Social Media Users’ Visual and Emotional Preferences of Internet-Famous Sites in Urban Riverfront Public Spaces: A Case Study in Changsha, China" Land 13, no. 7: 930. https://doi.org/10.3390/land13070930
Article Metrics
Article access statistics, further information, mdpi initiatives, follow mdpi.
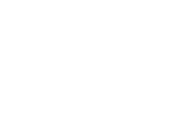
Subscribe to receive issue release notifications and newsletters from MDPI journals
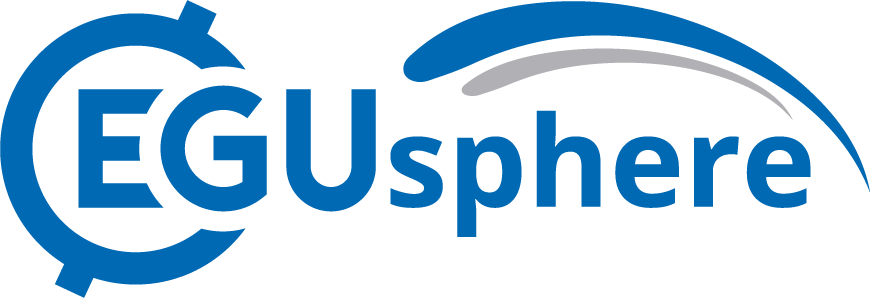
Preprint
- Preprint egusphere-2024-1163
Unheralded contributions of biogenic volatile organic compounds from urban greening to ozone pollution: a high-resolution modeling study
Abstract. Urban Green Spaces (UGS) are widely advocated for mitigating urban atmospheric environment. However, this study reveals that it can exacerbate urban ozone (O 3 ) levels under certain conditions, as demonstrated by a September 2017 study in Guangzhou, China. Utilizing the Weather Research and Forecasting Model with the Model of Emissions of Gases and Aerosols from Nature (WRF-MEGAN) and the Community Multiscale Air Quality (CMAQ) model with a high horizontal resolution (1 km), we assessed the impact of UGS-related biogenic volatile organic compound (BVOC) emissions on urban O 3 . Our findings indicate that UGS-BVOC emissions in Guangzhou amounted to 666.49 Gg, primarily from isoprene (ISOP) and terpenes (TERP). These emissions contribute ~30 % of urban ISOP concentrations and their incorporations to the model significantly reduce the underestimation against observations. The study shows improvements in simulation biases for NO 2 , from 7.01 µg/m 3 to 6.03 µg/m 3 , and for O 3 , from 7.77 µg/m 3 to -1.60 µg/m 3 . UGS-BVOC and UGS-LUCC (land use cover changes) integration in air quality models notably enhances surface monthly mean O 3 predictions by 3.6–8.0 µg/m 3 (+3.8–8.5 %) and contributes up to 18.7 µg/m 3 (+10.0 %) to MDA8 O 3 during O 3 pollution episodes. Additionally, UGS-BVOC emissions alone increase the monthly mean O 3 levels by 2.2–3.0 µg/m 3 (+2.3–3.2 %) in urban areas and contribute up to 6.3 µg/m 3 (+3.3 %) to MDA8 O 3 levels during O 3 pollution episodes. These impacts can extend to surrounding suburban and rural areas through regional transport, highlighting the need for selecting low-emission vegetation and refining vegetation classification in urban planning.
- Preprint (PDF, 21726 KB)
- Supplement (2433 KB)
- Preprint (21726 KB)
- Metadata XML

Status : open (until 06 Aug 2024)
Report abuse
Please provide a reason why you see this comment as being abusive. You might include your name and email but you can also stay anonymous.
Please provide a reason why you see this comment as being abusive.
Please confirm reCaptcha.

https://doi.org/10.5194/egusphere-2024-1163-supplement
HTML | XML | Total | Supplement | BibTeX | EndNote | |
---|---|---|---|---|---|---|
91 | 18 | 4 | 113 | 5 | 1 | 1 |
- Supplement: 5
Month | HTML | XML | Total | |
---|---|---|---|---|
Jun 2024 | 91 | 18 | 4 | 113 |
Month | HTML views | PDF downloads | XML downloads |
---|---|---|---|
Jun 2024 | 91 | 18 | 4 |
Viewed (geographical distribution)
Country | # | Views | % |
---|
Total: | 0 |
HTML: | 0 |
PDF: | 0 |
XML: | 0 |
Haofan Wang
Tianhang zhang.

IMAGES
VIDEO
COMMENTS
The aim of this paper is to look at the impact on the agricultural population in the urban fringe of both direct and indirect urbanization. Belgium was selected as a case study because the country is characterized by a strong urbanization, with a gradient from the centre towards the periphery and a long agricultural history.
Furthermore, considering the impact of urban expansion on the areas that provided a very high level of ESs, there were some new findings: 1) ... Linking ecosystem services and landscape patterns to assess urban ecosystem health: A case study in Shenzhen City, China. Landsc. Urban Plan., 143 (2015), pp. 56-68, 10.1016/j.landurbplan.2015.06.007.
In this study, we develop a system dynamics simulation model of the drivers and environmental impacts of urban growth, using Shenzhen, South China, as a case study. We identify three phases of urban growth and develop scenarios to evaluate the impact of urban growth on several environmental indicators: land use, air quality, and demand for ...
The larger the value is, the greater the impact of urbanization on ESH. The study carried out 999 permutations to test the significance (p < 0.05) in this context ... It also suggests the need to pay more attention to urban sprawl in the study area in case of possible ecosystem damage. Open in a separate window. Figure 5. Urbanization levels in ...
Previous global studies likely underestimate the impact of urbanization on water pollution because of their strong focus on single pollutants 10,16,20,22,23,24 (Fig. 1). Urbanization (e.g., sewer ...
Quantifying the impact of urban areas on climate dynamics is crucial for the prediction of precipitation forecasting at increasingly short temporal scale. ... 2006. Impacts of sewer leakage on urban groundwater: review of a case study in Germany. In: Urban groundwater management and sustainability, NATO Science Series, vol. 74. Dordrecht ...
An increase in average UIE-VG from 4 to 56% was found during urbanization of Kunming, the case study area in southwest China. ... indicates a positive indirect impact of urbanization on vegetation ...
The impact of urbanization on urban thermal environments can be assessed by comparing the difference between the urban and ... Lagos metropolis as a case study is located in southwestern ...
Abstract. The past decades have witnessed rapid urbanization around the world. This is particularly evident in Zhuhai City, given its status as one of the earliest special economic zones in China. After experiencing rapid urbanization for decades, the level of ecosystem health (ESH) in Zhuhai City has become a focus of attention.
Rapid urbanization has altered the regional hydrological processes, posing a great challenge to the sustainable development of cities. The TVGM-USWM model, a new urban hydrological model considering the nonlinear rainfall-runoff relationship and the flow routing in an urban drainage system, was developed in this study. We employed this model in the Huangtaiqiao drainage basin of Jinan City ...
This study aims to examine the disaster resilience level in the central urban area of Kunming, Yunnan-Guizhou Plateau, southwest China. Our focus lies on three representative dimensions: urban ecology, transportation, and flooding, which denote the natural, social, and combined natural-social safety resilience capacities of the study area.
Mohan M, Kandya A (2015) Impact of urbanization and land-use/land-cover change on diurnal temperature range: A case study of tropical urban airshed of India using remote sensing data. The Science of the Total Environment 506-507: 453-465. Crossref. PubMed. Google Scholar.
However, based on the complexity of urbanization's impact on the ECLU and operability of policy recommendations, this study measures the level of urbanization as the ratio of the population living in urban areas to the total population of both rural and urban areas (Wang et al., 2019).
population could reach 53 per cent by 2015, of. which 90 per cent of the growth is likely to occur. in cities of developing countries. In India, urban population increased from about 17 per cent ...
At about 4.4 percent, Africa has the fastest urbanization rate globally.Already, the region has reached 40 percent urbanization and by 2050, the number of urban residents will have doubled ...
Case studies of the impact of urban land use on rainfall using an NWP model follow a similar pattern. It is argued that sensitivity experiments are not reliable in determining the impact of urbanization on weather and climate owing to the uncertainties arising from changes in land use (Kusaka et al., ...
Urban flooding can differ significantly from rural flooding due to the influence of rapidly changing land use and rainfall patterns on runoff in urban areas. Consequently, understanding and managing urban flooding necessitate a comprehensive grasp of these influential factors. This study focuses on assessing the impact of land use and rainfall changes on runoff and flood resilience in urban ...
Urbanization has long been associated with human development and progress, but recent studies have shown that urban settings can also lead to significant inequalities and health problems. This paper is concerned with the adverse impact of urbanization on both developed and developing nations and both wealthy and poor populations within those nations, addressing issues associated with public ...
Urban Heat Island (UHI) in most cases has negative impact on health condition of the urban dwellers. However, the result in this study shows a negative phenomenon where the LST in the urban area had low intensity while the surrounding had high intensity as also discussed by Rasul et al., 2015 , Rasul et al., 2016 ).
Road connectivity is among the few factors which trigger the urbanisation and development process in a region. It facilitates trade, transport, social integration, economic development, socio-economic interaction and cultural assimilation. The present paper aims at analyzing the road infrastructure of Assam with special focus on the Central Brahmaputra Valley. The significance of the study ...
The results of this study indicate that while agriculture may, and it is not certain, be reducing its impact on the river, the impact of urbanisation is undoubtedly increasing. The impact of urbanisation is primarily from three sources: effluent discharges from WWTW, drainage stormwater from informal settlements, and stormwater from formal ...
The larger the value is, the greater the impact of urbanization on ESH. The study carried out 999 permutations to test the significance (p < 0.05) in this context ... Liu, Y.; Zhang, G.; Chen, Y. On the spatial relationship between ecosystem services and urbanization: A case study in Wuhan, China. Sci. Total Env. 2018, 637-638, 780-790.
Many researchers have found that urbanization has an impact on hydrological parameters such as runoff volume, discharge in drains, infiltration, interception, evapotranspiration etc. An attempt has been made to consolidate the review of literature related to impact of urbanization on hydrological parameters.
A case study of urbanization in India and metropolitan cities have been carried out leading to conclude on the existing causes of damage to the environment due to urbanization and preventive ...
A Sustainable Model of Urbanization for Indian Cities, A Case Study of New Delhi - written by Madhuri Agarwal , Ruchi , Farheen Alam Fakhr published on 2021/03/12 download full article with reference data and citations ... As a result of impact of urbanisation, land resources problems like soil erosion, water table contamination and vegetative ...
With the increasing online exposure of urban public spaces, the new concept of "internet-famous sites" has emerged in China. Social media users are the main contributors to this new phenomenon. To fully understand social media users' preferences in such kinds of public spaces, this article took 27 typical riverfront internet-famous sites (RIFSs) in Changsha City (China) as an example.
In general, land use and layout of streets can have a significant impact on the behavior of drivers and pedestrians. In particular, streetscape has often been overlooked that recognizing the role of streetscape on street accident in urban areas is important. The aim of this research is to investigate the influence of streetscape and land use on urban accidents that occurred in Mashhad between ...
Case study - development in an emerging country - India - Edexcel Advantages and disadvantages of rapid urbanisation India is a new emerging economy (NEE) that is experiencing rapid economic ...
Prior studies on urbanization's climate impact have often focused on impervious surfaces, with limited emphasis on UG's effect on LST through changes in evapotranspiration (ET) and Albedo. ... Imbalanced supply and demand of temperature regulation service provided by urban forests: A case study in Shenzhen. China. Ecological Indicators, 145 ...
Abstract. Urban Green Spaces (UGS) are widely advocated for mitigating urban atmospheric environment. However, this study reveals that it can exacerbate urban ozone (O3) levels under certain conditions, as demonstrated by a September 2017 study in Guangzhou, China. Utilizing the Weather Research and Forecasting Model with the Model of Emissions of Gases and Aerosols from Nature (WRF-MEGAN) and ...