- DOI: 10.4324/9780203097267.CH30
- Corpus ID: 148510510

Research on Teaching and Learning of Nature of Science
- Norman G. Lederman , J. Lederman
- Published 3 July 2014
- Education, Environmental Science
231 Citations
Teaching and learning nature of science in elementary classrooms, teaching and learning nature of scientific knowledge: is it déjà vu all over again.
- Highly Influenced
A Critical Review of Students’ and Teachers’ Understandings of Nature of Science
Teaching and learning of nature of science and scientific inquiry: building capacity through systematic research-based professional development, what we teach in science, and what learners learn: a gap that needs bridging, teaching and learning of nature of scientific knowledge and scientific inquiry: building capacity through systematic research-based professional development, experimenting matters: learning and assessing science skills in primary education, views from the chalkface, using core science ideas to teach aspects of nature of science in the elementary grades, related papers.
Showing 1 through 3 of 0 Related Papers
Breadcrumbs Section. Click here to navigate to respective pages.

Research on Teaching and Learning of Nature of Science
DOI link for Research on Teaching and Learning of Nature of Science
Click here to navigate to parent product.
Volumes have been written arguing why NOS is an important educational objective. Simply put, understanding NOS is often defended as being a critical component of scientifi c literacy (Lederman & Lederman, 2011; NGSS Lead States, 2013; NSTA, 1982). For more elaborated rationales, the reader is referred to Driver, Leach, Millar, and Scott (1996) and Lederman (2007).
- Privacy Policy
- Terms & Conditions
- Cookie Policy
- Taylor & Francis Online
- Taylor & Francis Group
- Students/Researchers
- Librarians/Institutions
Connect with us
Registered in England & Wales No. 3099067 5 Howick Place | London | SW1P 1WG © 2024 Informa UK Limited
- Publications
- Conferences & Events
- Professional Learning
- Science Standards
- Awards & Competitions
- Instructional Materials
- Free Resources
- American Rescue Plan
- For Preservice Teachers
- NCCSTS Case Collection
- Science and STEM Education Jobs
- Interactive eBooks+
- Digital Catalog
- Regional Product Representatives
- e-Newsletters
- Bestselling Books
- Latest Books
- Popular Book Series
- Prospective Authors
- Web Seminars
- Exhibits & Sponsorship
- Conference Reviewers
- National Conference • Denver 24
- Leaders Institute 2024
- National Conference • New Orleans 24
- Submit a Proposal
- Latest Resources
- Professional Learning Units & Courses
- For Districts
- Online Course Providers
- Schools & Districts
- College Professors & Students
- The Standards
- Teachers and Admin
- eCYBERMISSION
- Toshiba/NSTA ExploraVision
- Junior Science & Humanities Symposium
- Teaching Awards
- Climate Change
- Earth & Space Science
- New Science Teachers
- Early Childhood
- Middle School
- High School
- Postsecondary
- Informal Education
- Journal Articles
- Lesson Plans
- e-newsletters
- Science & Children
- Science Scope
- The Science Teacher
- Journal of College Sci. Teaching
- Connected Science Learning
- NSTA Reports
- Next-Gen Navigator
- Science Update
- Teacher Tip Tuesday
- Trans. Sci. Learning
MyNSTA Community
- My Collections
Position Statement
Nature of Science
Share Start a Discussion
Introduction
Nature of science (NOS) is a critical component of scientific literacy that enhances students’ understandings of science concepts and enables them to make informed decisions about scientifically-based personal and societal issues. NOS is derived not only from the eight science practices delineated in the Framework for K–12 Science Education (2012), but also from decades of research supporting the various forms of systematic gathering of information through direct and indirect observations of the natural world and the testing of this information by the various research methods used in science, such as descriptive, correlational, and experimental designs. All science educators and those involved with science teaching and learning should have a shared accurate view of nature of scientific knowledge, and recognize that NOS should be taught explicitly alongside science and engineering practices, disciplinary core ideas, and crosscutting concepts.
It is important to know that this new iteration of NOS improves upon the previous NSTA position statement on this topic (NSTA 2000) that used the label “nature of science,” which included a combination of characteristics of scientific knowledge (NOS) and scientific inquiry. It demonstrated the common conflation of how scientific knowledge is developed and its characteristics. Since the recent NSTA position statement on science practices, previously referred to as “inquiry” (NSTA 2018), clearly delineates how knowledge is developed in science, a more appropriate label for the focus of this position statement would be “nature of scientific knowledge” (NOSK). This would clarify the difference between how knowledge is developed from the characteristics of the resulting knowledge. Clearly the two are closely related, but they are different (Lederman & Lederman 2014). However, introducing a new label (i.e., NOSK), given that the NGSS refers to the characteristics of scientific knowledge as NOS, would create more confusion. It will be clear that the discussion of NOS here is about the characteristics of scientific knowledge. Additionally, the word “the” is removed preceding NOS to avoid implying that a single set of knowledge characteristics exists.
Why Learn About Nature of Science?
Understanding of NOS is a critical component of scientific literacy. It enhances students’ understandings of science concepts and enables them to make informed decisions about scientifically-based personal and societal issues. Although NOS has been viewed as an important educational outcome for science students for more than 100 years, it was Showalter’s (1974) work that galvanized NOS as an important construct within the overarching framework of scientific literacy. Admittedly, the phrase scientific literacy had been discussed by numerous others before Showalter (e.g., Dewey 1916; Hurd 1958; National Education Association 1918, 1920; National Society for the Study of Education 1960; among others), but it was his work that clearly delineated the dimensions of scientific literacy in a manner that could easily be translated into objectives for science curricula. NOS and science processes (now known as inquiry or practices) were clearly emphasized as equally important as “traditional” science subject matter and should also be taught explicitly, just as is done with other science subject matter (Bybee 2013). The attributes of a scientifically literate individual were later reiterated and elaborated upon by the National Science Teachers Association (NSTA 1982).
Declarations
The National Science Teaching Association endorses the proposition that science, along with its methods, explanations, and generalizations, must be the sole focus of instruction in science classes to the exclusion of all nonscientific or pseudoscientific methods, explanations, generalizations, and products.
NSTA makes the following declarations for science educators to support teaching NOS . The following premises, as well as the terminology (e.g., tentative, subjective, etc.) of nature of science, are critical and developmentally appropriate (for precollege students). They should be understood by all students by the time they graduate high school. The understandings are elaborated slightly beyond the items listed in the Next Generation Science Standards ( NGSS ).
- Scientific knowledge is simultaneously reliable and subject to change. Having confidence in scientific knowledge is reasonable, while also realizing that such knowledge may be abandoned or modified in light of new evidence or a re-conceptualization of prior evidence and knowledge. The history of science reveals both evolutionary and revolutionary changes. With new evidence and interpretation, old ideas are replaced or supplemented by newer ones. Because scientific knowledge is partly the result of inference, creativity, and subjectivity, it is subject to change (AAAS 1993; Kuhn 1962).
- Although no single universal step-by-step scientific method captures the complexity of doing science, a number of shared values and perspectives characterize a scientific approach to understanding nature. Among these are a demand for naturalistic explanations supported by empirical evidence that are, at least in principle, testable against the natural world. Other shared elements include observations, rational argument, inference, skepticism, peer review, and reproducibility of the work. This characteristic of science is also a component of the idea that “science is a way of knowing” as distinguished from other ways of knowing (Feyerabend 1975; Moore 1993; NGSS Lead States 2013).
- In general, all scientific knowledge is a combination of observations and inferences (Chalmers 1999; Gould 1981). For example, students of all ages pay attention to weather forecasts. Weather forecasters make observations, and their forecasts are inferences. All science textbooks have a picture of the atom, but the picture is really an inference from observable data of how matter behaves.
- Creativity is a vital, yet personal, ingredient in the production of scientific knowledge. It is a component of science as a human endeavor (Bronowski 1956; Hoffman & Torrence 1993; Kuhn 1962).
- Subjectivity is an unavoidable aspect of scientific knowledge. Because “science is a human endeavor,” it is subject to the functions of individual human thinking and perceptions. Although objectivity is always desired in the interpretation of data, some subjectivity is unavoidable and often beneficial (Chalmers 1999; Gould 1981; Laudan 1977).
- Science, by definition, is limited to naturalistic methods and explanations, and as such, is precluded from using supernatural elements in the production of scientific knowledge. This is a component of the recognition that scientific knowledge is empirically based (Hoffman & Torrence 1993).
- A primary goal of science is the formation of theories and laws, which are terms with very specific meanings:
- Laws are generalizations or universal relationships related to the way that some aspect of the natural world behaves under certain conditions. They describe relationships among what has been observed in the natural world. For example, Boyle’s Law describes the relationship between pressure and volume of a gas at a constant temperature (Feynman 1965; Harre 1983; National Academy of Sciences 1998).
- Theories are inferred explanations of some aspect of the natural world. They provide explanations for what has been stated in scientific laws. Theories do not become laws even with additional evidence; they explain laws. However, not all scientific laws have accompanying explanatory theories (Feynman 1965; Harre 1983; Mayr 1988; National Academy of Sciences 1998; Ruse 1998).
- be internally consistent and compatible with the best available evidence;
- be successfully tested against a wide range of applicable phenomena and evidence; and
- possess appropriately broad and demonstrable effectiveness in further research (Kuhn 1962; Lakatos 1983; Popper 1968).
- Contributions to science can be made and have been made by people the world over. As a consequence, science does not occur in a vacuum. It affects society and cultures, and it is affected by the society and culture within which it occurs (AAAS 1993; Showalter 1974).
- The scientific questions asked, the observations made, and the conclusions in science are to some extent influenced by the existing state of scientific knowledge, the social and cultural context of the researcher, and the observer’s experiences and expectations. Again, scientific knowledge is partially subjective and socially and culturally embedded (Lederman & Lederman 2014; NSTA 2000).
These premises combined provide the foundation for how scientific knowledge is formed and are foundational to nature of science. The NGSS (2013) lists the following eight components of NOS. Given the previous discussion about the differences between how knowledge is developed and what is done with that knowledge as scientific practice, items 1, 5, and 6 are arguably more aligned with science practices (or inquiry) than characteristics of scientific knowledge. Practices and knowledge are obviously entangled in the real world and in classroom instruction, yet it is important for teachers of science to know the difference between science practices and the characteristics of scientific knowledge to best lead students to a comprehensive understanding of nature of science. Items 5 and 7 are a bit vague for concrete use in K–12 classrooms. Consequently, a more concrete discussion of what these items mean was provided in the previous section.
NSTA recommends that by the time they graduate from high school, students should understand the following concepts related to NOS:
- Scientific Investigations Use a Variety of Methods;
- Scientific Knowledge Is Based on Empirical Evidence;
- Scientific Knowledge Is Open to Revision in Light of New Evidence;
- Science Models, Laws, Mechanisms, and Theories Explain Natural Phenomena;
- Science Is a Way of Knowing;
- Scientific Knowledge Assumes an Order and Consistency in Natural Systems;
- Science Is a Human Endeavor; and
- Science Addresses Questions About the Natural and Material World.
Concluding Remarks
NOS (i.e., the characteristics of scientific knowledge as derived from how it is produced) has long been recognized as a critical component of scientific literacy. It is necessary knowledge for students to make informed decisions with respect to the ever-increasing scientifically-based personal and societal issues. The research clearly indicates that for students to learn about NOS, it must be planned for and assessed just like any of the instructional goals focusing on science and engineering practices, disciplinary core ideas, and crosscutting concepts (Lederman 2007; Lederman & Lederman 2014). It is not learned by chance, simply by doing science. NOS is best understood by students if it is explicitly addressed within the context of students’ learning of science and engineering practices, disciplinary core ideas, and crosscutting concepts. “Explicit” does not mean that the teacher should lecture about NOS. Rather, it refers to reflective discussions among students about the science concepts they are learning (Clough 2011).All aspects of NOS cannot and should not be taught in a single lesson, nor are all aspects developmentally appropriate for all grade levels. For example, understandings of the differences between theories and laws or the cultural embeddedness of science are not developmentally appropriate for K–5 students. Nevertheless, NOS should be included at all grade levels as a unifying theme for the K–12 science curriculum. All too often, NOS is only taught explicitly at the beginning of a science course, independent of any of the science content that will subsequently follow. Instead, NOS should be taught as a unifying theme with the expectation that students’ knowledge will progressively become more and more sophisticated as they progress through the K–12 curriculum.
—Adopted by the NSTA Board of Directors, January 2020
Research and Theoretical References
Abd-El-Khalick, F., and N.G. Lederman. 2000. Improving science teachers’ conceptions of the nature of science: A critical review of the literature. International Journal of Science Education 22 (7): 665–701.
American Association for the Advancement of Science (AAAS). 1993. Benchmarks for science literacy. New York: Oxford University Press.
Bronowski, J. 1956. Science and human values. New York: Harper & Row Publishers, Inc.
Bybee, R.W. 2013. Translating the NGSS for classroom imstruction. Arlington, VA: NSTA Press.
Chalmers, A.F. 1999. What is this thing called science? Queensland, AU: University of Queensland Press.
Dewey, J. 1916. Democracy and education. New York: The Free Press.
Feyerabend, P.F. 1975. Against method: Outline of an anarchistic theory of knowledge. Great Britain: Redwood, Burn Limited.
Feynman, R.P. 1965. The character of physical law. Cambridge, MA: MIT Press.
Gould, S.J. 1981. The mismeasure of man. New York: W.W. Norton & Company.
Hoffman, R., and V. Torrence. 1993. Chemistry imagined: Reflections on science. Washington, DC: Smithsonian Institution Press.
Hurd, P.D. 1958. Science literacy : 16 (1): 13–16.
Kuhn, T.S. 1962. The structure of scientific revolutions. Chicago: The University of Chicago Press.
Lakatos, I. 1983. Mathematics, science, and epistemology. Cambridge, UK: Cambridge University Press.
Laudan, L. 1977. Progress and its problems: Towards a theory of scientific growth. Berkeley, CA: University of California Press.
Lederman, N.G. 2007. Nature of science: Past, present, and future. In Handbook of research on science education, ed. S.K. Abell and N.G. Lederman, 831–880. Mahwah, NJ: Lawrence Erlbaum Associates.
Lederman, N.G., and J.S. Lederman. 2014. Research on teaching and learning of nature of science. In Handbook of research on science education, Volume II, ed. N.G. Lederman and S.K. Abell, 600–620. New York: Routledge.
Mayr, E. 1988. Toward a new philosophy in biology. Cambridge, MA: Harvard University Press.
Moore, J. 1993. Science as a way of knowing: The foundation of modern biology . Cambridge, MA: Harvard University Press.
National Education Association. 1918. Cardinal principles of secondary education: A report of the commission on the reorganization of secondary education. (U.S. Bureau of Education Bulletin No. 35). Washington, DC: U.S. Government Printing Office.
National Education Association. 1920. Reorganization of science in secondary schools: A report of the commission on the reorganization of secondary education. (U.S. Bureau of Education Bulletin No. 20). Washington, DC: U.S. Government Printing Office.
National Research Council (NRC). 2012. A framework for K–12 science education: Practices, crosscutting concepts, and core ideas. Washington, DC: National Academies Press. National Science Teachers Association. 1982. Science-technology-society: Science education for the 1980s. Washington, DC: Author.
National Science Teachers Association. 2018. Transitioning from scientific inquiry to three-dimensional teaching and learning. Arlington, VA: Author.
National Science Teachers Association. 2000. The nature of science: NSTA Position Statement . Arlington, VA: Author.
National Society for the Study of Education. 1960. Rethinking Science Education: Yearbook of the National Society for the Study of Education. Chicago: University of Chicago Press 59: 113.
NGSS Lead States. 2013. Next generation science standards: For states, by states. Washington, DC: National Academies Press. www.nextgenscience.org/next-generation-science-standards.
Popper, K.R. 1968. The logic of scientific discovery. New York: Harper & Row Publishers.
Ruse, M. (Ed.) 1998. Philosophy of biology. New York: Prometheus Books.
Showalter, V.M. 1974. What is unified science education? Program objectives and scientific literacy. Prism 2 (3–4): 1–6.
References of Teaching Resources
Bell, R.L. 2008. Teaching the nature of science through process skills: Activities for grades 3–8 . New York: Pearson.
Clough, M.P. 2011. Teaching and assessing the nature of science: How to effectively incorporate the nature of science in your classroom. The Science Teacher 78 (6): 56–60
Clough, M.P., and J.K. Olson. 2004. The nature of science: Always part of the science story. The Science Teacher 71 (9): 28–31.
Lederman, N.G., and F. Abd-El-Khalick. 1998. Avoiding de-natured science: Activities that promote understandings of the nature of science. In The nature of science in science education: Rationales and strategies , ed. W.F. McComas, 83–126. The Netherlands: Kluwer Academic Publishers.
McComas, W.F., ed. 2019. Nature of science in science instruction: Rationales and strategies . Dordrecht, The Netherlands: Springer Publishing.
National Academy of Sciences. 1998. Teaching about evolution and the nature of science . Washington, DC: National Academies Press.
Breadcrumbs Section. Click here to navigate to respective pages.

Research on Teaching and Learning of Nature of Science
DOI link for Research on Teaching and Learning of Nature of Science
Click here to navigate to parent product.
The purpose of this chapter is to update new advances in the research on the teaching and learning of nature of science since the previous Handbook of Research on Science Education (Abell & Lederman, 2007). As this is a new volume, the previous handbook will remain in print, and so a complete recapitulation of what was published previously is not necessary. However, some review of research considered in the previous handbook is needed to provide some context for the more recent work. Consequently, reference to some of the more influential studies and findings reported in the previous handbook chapter will be referenced, but an attempt is made not to reprint all that can be found in the first handbook. More than 7 years have passed, and it is debatable if anything new has been revealed relative to nature of science (NOS). One could say that there is more than 7 years of new research. On the other hand, little new about how students learn NOS or how it is best taught has been revealed. However, research on NOS does continue to be a vibrant area of concern. Alternatively, there has been much written that attempts to reconceptualize how NOS is viewed. Indeed, some of this reconceptualization can be found in the Next Generation Science Standards in the United States (NGSS Lead States, 2013), but how well this newly advocated view is consistent with the existing empirical research certainly warrants discussion. That said, this chapter will be organized around a conceptualization of the construct “nature of science” and how it is taught, learned, and assessed. In addition, there will be a discussion of recent trends regarding thinking about nature of science and how these trends may or may not help improve students’ and teachers’ understandings of nature of science.
- Privacy Policy
- Terms & Conditions
- Cookie Policy
- Taylor & Francis Online
- Taylor & Francis Group
- Students/Researchers
- Librarians/Institutions
Connect with us
Registered in England & Wales No. 3099067 5 Howick Place | London | SW1P 1WG © 2024 Informa UK Limited
- Open access
- Published: 17 July 2018
Improving science teachers’ nature of science views through an innovative continuing professional development program
- Eda Erdas Kartal ORCID: orcid.org/0000-0002-1568-827X 1 , 7 ,
- William W. Cobern 2 ,
- Nihal Dogan 3 ,
- Serhat Irez 4 ,
- Gultekin Cakmakci 5 &
- Yalcin Yalaki 6
International Journal of STEM Education volume 5 , Article number: 30 ( 2018 ) Cite this article
11k Accesses
24 Citations
1 Altmetric
Metrics details
This study describes how teachers’ nature of science (NOS) views changed throughout an innovative Continuing Professional Development (CPD) program that provided sustained support throughout the process in a collaborative and reflective environment and activities that are consistent with the current curriculum and NOS tenets integrated within. Eighteen in-service science teachers enrolled in a yearlong nature of science, Continuing Professional Development (NOS-CPD) program. Data were collected by pre/post-interviews using the Views of Nature of Science-Form C (VNOS-C) questionnaire, and a post-interview using an open-ended questionnaire developed by researchers to uncover teacher reactions to the NOS-CPD program.
The results indicated that the NOS-CPD program improved the teachers’ NOS views more effectively than previously reported short-term teacher development programs, and thus, the findings should be useful for future studies in support of the professional development of teachers.
Conclusions
The article concludes with practical advice for implementing NOS-focused, in-service teacher development programs.
The transformational change agent says: “Here is the standard, which I know is impossible, so let’s stand together and learn our way into a higher level of performance.” Robert Quinn (2000, p.164)
Equipping individuals with adequate knowledge and understanding of science and technology has become one of the main goals of national education programs (e.g., Ministry of National Education (MONE Turkey) 2004 , 2013 ; Next Generation Science Standards in the USA (NGSS Lead States) 2013 ). Calls for scientific literacy echo across many countries. These national science education programs include goals for understanding the nature of science (NOS) as an important component of scientific literacy. Although there is no NOS consensus definition (Cobern and Loving 2001 ; Lederman 1992 ) much of the science education community, nevertheless, agrees that NOS should be highlighted in the curriculum and taught to students (Lederman 1992 ). And, there are broadly accepted models of the NOS. Unfortunately, studies show that Turkish students often have inadequate views and misconceptions about NOS (Lederman and Lederman 2014 ; Ozer 2014 ; Park et al. 2013 ).
Numerous studies have tested methods for improving students’ NOS views. Although these studies show that NOS instruction can be made more effective, the studies indicate that there is room for still the improvement of students’ understanding of NOS. Moreover, NOS studies suggest that some science teachers have naive conceptions about NOS and numerous misconceptions (Akerson et al. 2009 ; Dogan and Abd-El-Khalick 2008 ; Guerra-Ramos et al. 2010 ). Teachers need to have informed NOS views since they cannot help their students understand what they themselves do not understand (Capps et al. 2012 ; Loucks-Horsley and Matsumoto 1999 ).
Thus, effective professional development opportunities are important for helping teachers to improve their understanding of NOS, and research with teachers shows that professional development programs can improve teachers’ NOS views (Akerson et al. 2009 ; Ozer 2014 ). The literature indicates that effective professional development programs have the following features:
Based on teacher needs
Designed to fit personal needs of the participating teachers (Gess-Newsome 2001 ).
Coherency with other reform initiatives
Stresses reform-oriented practices such as teacher mentoring or coaching, participating in a committee or study group (Garet et al. 2001 ) with a focus on curriculum linked activities rather than general pedagogical strategies (Cohen and Hill 2001 ).
High-quality instruction: Explicitly designed to improve teachers’ content knowledge and practices (Bertram and Loughran 2012 ; Posnanski 2010 ).
Active engagements of teachers
Based on the principles of active learning (Boone and Kahle 1998 ; Marek and Methven 1991 ).
Enhancement of both content knowledge and pedagogical content knowledge
Stresses the importance of both content and pedagogical knowledge (Shulman 1987 ).
Provision of sufficient time and other resources
Provides sufficient time in a well-organized, carefully structured, and purposefully directed environment consistent with the curricula and provides relevant resources and materials.
Sustained support: Provides continuing support that helps them overcome these challenges (Capps et al. 2012 ).
Ensuring collaboration
Provides opportunities for teacher collaboration (Putnam and Borko 1997 ).
Provision opportunity for reflection and giving feedback throughout the professional development program process
Provides opportunities for teacher reflection on what they are learning and how they will apply what they learned (Loucks-Horsley and Matsumoto 1999 ). Provides feedback to teachers using these reflective comments made by teachers, and so, these reflective comments can be a valuable tool for teacher learning and teacher change (Capps and Crawford 2013 ).
Provision of local support
Develop local support for teachers when they return to their classrooms (Kwakman 2003 ; Penuel et al. 2007 ).
Where professional development programs often fail is with the “provision of sufficient time” and “sustained support.” Researches show that long-term professional development programs are more effective than short-term programs (Dass and Yager 2009 ) because learning to teach and fundamental change in practice is not easy and takes time (Guskey and Yoon 2009 ). Too often professional development does not follow teachers back to the classroom where teachers may face some challenges and problems while translating their new understanding into performance. The limited time in these professional development programs does not allow this. Effective professional development requires supporting teachers in the transfer of what they learned into practice (Gess-Newsome 2001 ; Ozer 2014 ).
The above comments are about professional development in general. Our specific interest is professional development with respect to the NOS. Given the importance of sustained professional development support as discussed above, our study planned a CPD program that provides teachers with such support. As professional development in support of teachers’ understanding of NOS, our approach followed research findings in two important areas:
Researches have demonstrated that explicit-reflective instruction in teaching NOS is typically more effective than implicit instruction (Abd-El-Khalick and Lederman 2000 ; Khishfe and Abd-El-Khalick 2002 ).
Researches have also demonstrated that NOS instruction can be more effective when context-specific activities are used rather than generic activities (Cakmakci 2012 ; Sadler et al. 2010 ).
In addition to observing the above research-based, professional development practices, we innovated by including formative assessment and discourse analysis within our NOS-CPD program. Researches have shown that using formative assessment rather than summative assessment can improve learning (Bennett 2011 ); summative assessment often comes too late to be much help (Guskey 2000 ). On the other hand, given that teachers use a variety of communication approaches and patterns of discourse in the classroom that impact student learning (Kaya et al. 2016 ; Mortimer and Scott 2003 ; Sinclair and Coulthard 1975 ), researches indicate that teacher-student communication in the classroom needs to be examined with regard to NOS issues (Herman et al. 2013 ). It is thought that teacher awareness of their NOS discourse patterns and communication approaches can be improved by analyzing classroom discourses. For these reasons, it has been decided to use these formative assessment and discourse analysis within the teaching of the NOS in our NOS-CPD program.
Aim of the study
This study evaluated the effectiveness of an innovative NOS-CPD program with specific attention paid to how teachers’ NOS views change throughout the sustained, CPD program. The main research question is: In response to an innovative NOS-CPD program that provided sustained support, to what extent and in what ways do teachers’ NOS views change? The subquestion then is: To what extent do these changes show improvement over short-term PD programs?
The NOS-CPD program innovation
This paper reports the findings of our study on NOS-CPD program effectiveness. The NOS-CPD program was part of a large-scale Turkish teacher professional development research project intended to improve middle school in-service science teachers’ professional competences about NOS and consisted of a preparation stage and an implementation stage (see Fig. 1 ).
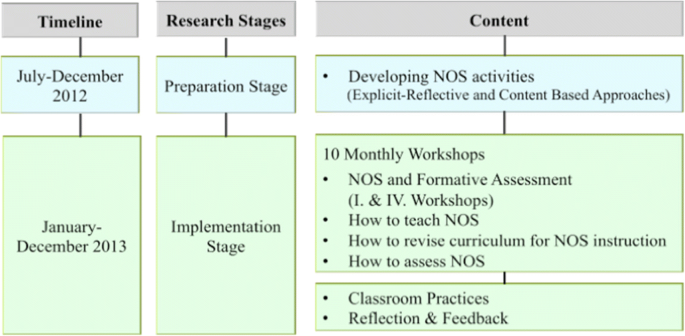
Process of the research
The NOS-CPD program consisted of NOS activities, with eight NOS themes emphasized in the activities: empirical NOS, scientific method, tentative nature of science, the nature of scientific theories and laws, inference and theoretical entities in science, the subjective and theory-laden NOS, the social and cultural embeddedness of science, and imagination and creativity in science. The themes were derived from: the general thematic structure of the VNOS-C (Lederman et al. 2002 ), the characteristics of NOS intended to be developed in this project, and the analytical frameworks used in several researches examining the understanding of various groups (e.g., students, teachers, scientists) about NOS (e.g., Irez 2006 ). The NOS-CPD program model is as follows as shown in Fig. 2 .
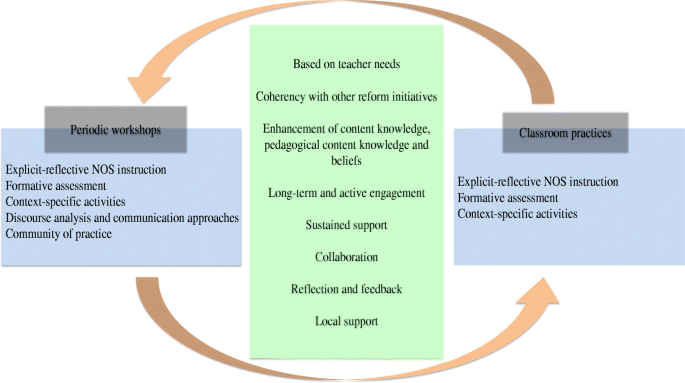
Model of the innovative NOS-CPD program
Participating teachers in the NOS-CPD program attended 10, monthly workshops, each consisting of 8 h, over the course of two semesters. The teachers were introduced in a collaborative and reflective environment to various NOS aspects and ways of using explicit instruction and formative assessment in their NOS teaching. They were also introduced to different patterns of discourse and communication approaches by analyzing video recordings in the classroom. The teachers were also provided with opportunities to develop and use various context-specific NOS activities in their own classrooms during the study. During the workshops, context-specific NOS activities were introduced to the teachers and the teachers’ opinions about the activities were taken. Teachers were asked to apply the shared activities in their classes; the next workshop allowed them to reflect on their experiences and thoughts on their practice. Activities were reorganized according to the views and suggestions from the teachers. During this process, the teachers along with the researchers collaboratively produced 57 NOS activities all meeting the project criteria. The activities are available at Dogan et al. ( 2016 ).
Each of the activities consists of four sections: introduction, implementation, guidance for classroom discussion, and formative assessment. The activity introduction provides information on the subject matter, the purpose of the activity, and specifies the NOS themes taught by the activity, and what questions the students should be able to answer after the implementation. The implementation section provides guidelines just on how to implement the activity, including points to be emphasized during the implementation of the activity. The guidance for classroom discussion section provides teachers with instructional tips on the explicit-reflective teaching of NOS. Finally, in the formative assessment section of the activity, there were sample questions that will help the teacher to formatively assess students’ NOS achievement.
Participants
Eighteen (11 female, 7 male) in-service middle school science teachers (teaching grades 5 through 8) volunteered to participate in this study. These teachers worked in 15 different schools in Turkey. They regularly attended project meetings and fulfilled all participation requirements. Thirteen of 18 (72.2%) participating teachers had previously taken a short-term course or training about the history of science and philosophy of science or NOS.
Data collection and analyses
The data were collected through interviews and analyzed using content analysis. Participant’s NOS understandings were assessed through face-to-face interviews at the beginning and end of the second stage. These interviews were semi-structured based on VNOS-C questions as developed by Abd-El-Khalick ( 1998 ). Although Abd-El-Khalick developed the original questionnaire as a paper-pencil instrument, the questions have been found appropriate for use in interviews (Irez 2006 ).
Analyses of the interviews were carried out in several steps. First, interviews were transcribed. Second, these transcripts were transferred to a qualitative data analysis program. Thirdly, teachers’ statements were grouped regarding NOS themes. At the 4th stage of the analysis, teachers’ statements about related themes were classified as naive , eclectic , and informed. Table 1 provides the “operational definitions” for the categories of naive, eclectic, and informed.
A rubric was used that developed by Irez ( 2004 ), defining each of these categories for each theme, to aid the classifying procedure (Table 2 ). In this analysis, insufficient views about relevant theme of NOS were labeled naive, views characterized by inconsistent and often conflicting statements about the NOS were labeled eclectic, and consistent views with the contemporary approaches about relevant theme of NOS were labeled informed (Irez 2004 ; Koulaidis and Ogborn 1988 ). Before classifying all teacher statements according to themes, inter-rater reliability was checked. Participant transcripts were given to two raters for independent classification. Inter-rater reliability was found to be 82%. Differences were reconciled through discussion between the raters, then all teacher statements classified. All data is reported using pseudonyms.
An improvement was observed in all participant teachers’ NOS views at the end of the innovative NOS-CPD program. Teacher’s naive views about NOS themes decreased whereas their informed views increased (Table 3 ). On the other hand, it would be expected that the pre-performances of the teachers who had previously taken courses or training about the history of science and philosophy of science or NOS would do better than those without previous experience; but as can be seen from the ratios in the table, there is no significant difference between the pre- and post-performances of the teachers who did and did not take the courses. Before the NOS-CPD program, it was seen that most of the teachers had naive views on the most of the 8-targeted NOS themes, regardless of whether they had taken courses before or not. There is also no significant difference in the increase in the performances of the two groups.
For the themes specifically targeted by the program, the percent of teachers who had naive views about these themes decreased whereas the percent of teachers with informed increased (Table 4 ).
As it is seen on the Table 4 , the theme in which the furthest progress was made as a result of the CPD program is “scientific method.” While 72% of the teachers had informed views regarding this theme before the program, the ratio was reduced to zero at the end. All of the teachers comprehended that the scientific method was not the only and universal one.
It is hard to talk about a universal method in general as scientists might have different methods even though they are working on the same subject. (Lara, post-interview )
A very considerable increase was observed in the ratio of teachers indicating that the scientific method is not composed of steps that are followed one by one and nor is it unique and universal.
In my opinion, every scientist has his own method. So it is not possible that every scientist follows the same steps in the scientific method. (Irmak, post-interview )
The “Imagination and creativity in science” theme is one of those in which a high level of success was achieved in the aftermath of the CPD program. The eclectic level to which 61% of the teachers belonged decreased to 11% after the study and the ratio of informed teachers reached to 89%. Most of the teachers comprehended that scientists use their imagination and creativity at every stage of their studies:
They might use their imagination and creativity at every stage, however they might use it more at some stages. For example, they might use their imagination/creativity less while recording data whereas they use it a lot when making an observation and maybe more when making a deduction. Still, imagination and creativity are present at every stage. (Sevgi, post-interview )
As it is seen on the table, the ratio of teachers sharing informed views about “the social and cultural embeddedness of science” theme after the program is 100%. All of the teachers underlined that science was not universal, and scientific studies might be affected from the culture and the values of the society:
The needs of a society, personal needs, religious opinion and even the languages spoken have an effect on scientific studies. (Sarp, post-interview )
The improvement achieved in the “nature of scientific theories and laws” theme as a result of the CPD program was less than expected. The percentage of teachers having naive views about the theme before the program decreased from 83 to 44%; however, the percentage of teachers having an informed views about the theme increased from 6 to only 34%. All teachers stressed that the theories might change, but some of them were persistent in their opinion that there was a hierarchical relation between theories and laws and that theories turned into unalterable laws when proved.
I think law is a proved theory. (Akin, post-interview )
One of the teachers sharing a conscious opinion after the program while she had naive views regarding the “nature of scientific theories and laws” theme before, clearly underlined her opinion regarding this subject:
I would give a very good answer to that question before; I kept the cliché sentence ‘theories are developed, proven and turn into law’ in my mind for years. However, I do think different now. There might be a mutual interaction. A law might be explained by more than one theory. (Duru, post-interview )
Discussion and conclusion
Our primary research question asked in what ways and to what extent does teachers NOS views change in response to an innovative NOS-CPD program that provided sustained support? In response, our research findings showed that the innovative NOS-CPD program improved teachers’ NOS knowledge and understanding in general. For the themes specifically targeted by the program, the percent of teachers who had naive views about all these themes decreased whereas the percent of teachers with informed increased. As a result of the innovative NOS-CPD program, the NOS theme in which the furthest progress was made is “scientific method.” Another theme in which a high level of progress was made is the “imagination and creativity in science” theme. The improvement achieved in “nature of scientific theories and laws” theme as a result of the innovative NOS-CPD program was less than expected. It is more difficult to ensure improvement in some NOS themes than others even if the direct-reflective teaching method is used. “Nature of scientific theories and laws” is one of those (Koseoglu et al. 2010 ). The researches carried out in this field claim that the educational (the need for more examples and activities in some subjects than in others), motivational (intrinsic task motivation, performance motivation, utility value, competence belief, self-efficacy, peer support, team work, work a real science research lab.), and socio-cultural (socio-cultural state of the participants, especially with respect to background and possible worldview differences, such as reluctance to accept ambiguity) factors can explain the difficulty of making an improvement in views about this theme (Mesci and Schwartz 2016 ). It is recommended to be more taken into consideration of these factors mentioned in the literature and to be emphasized in training and activities with extra examples of the NOS themes, which are relatively harder than the other themes.
Our subquestion asked to what extent was our program more effective than short-term professional development programs? In response, our research findings showed that the innovative NOS-CPD program is more successful than short-term programs improving teachers’ views of the NOS themes, especially which are difficult to change as scientific method or the nature of scientific theory and laws. When we looked at the literature, studies have generally demonstrated that short-term professional development programs are difficult to change teachers’ views on such NOS themes (Dogan et al. 2011 ; Dass and Yager 2009 ; Torff and Sessions 2008 ). For example in one study by Dogan et al. ( 2011 ) investigating the effects of a 1-week in-service training program on teachers’ views on the nature of science, it is seen that the majority of the views teachers have about NOS have not changed. In this study, it was concluded that such short-term in-service training program was not sufficient in order to be able to make a change in the opinions of teachers, such as theories and laws, where teachers were found to have quite common misconceptions in many studies. As a result of this study, researchers stated, as stated in many studies, many different techniques have to be applied for a longer time to correct such misconceptions. Koseoglu et al. ( 2011 ) have also achieved similar results in their experimental works aimed at developing a professional development package for the NOS instruction. One of the important results obtained during the study is that a long process is needed to change the opinions about the NOS. As a result of various activities and debates during the first semester, the rate of good opinions increased to 14.4 and 38.1%, respectively, and it was necessary to apply one more period in order to reach this rate to 67%. In the interim evaluations made, it was seen that most of the participants were aware of the intrinsic insights of the focused science, but inconsistent opinions were seen when their opinions were taken in different contexts. This finding has shown that even a period of education that is explicitly focused on the NOS is not sufficient to internalize the NOS. On the other hand, it has been determined in our study that having already taken a course or training about the history of science and philosophy of science or NOS does not make a difference in the pre- and post-performance of the teachers. The fact that the pre-performances of the teachers who have already taken the courses as they are in the teachers who do not take courses show that the courses they have taken do not have a lasting effect on them. Researches show that short-term professional development programs do not permanently improve teachers’ views about NOS (Akerson and Hanuscin 2007 ; Koseoglu et al. 2011 ). Thus, it is thought that this situation may have been caused by the fact that the courses that the teachers had previously attended were short-term.
Learning to teach is a slow process and note easy. Therefore, it should be taken into account that teachers may have difficulty changing their previous knowledge and misconceptions, and professional development programs should be designed for a long time with this prediction (Hayes 1995 ). In addition, the teachers’ classroom practices should be followed during and after the professional development programs, should be supported to solve the problems encountered in the integration of science with the NOS, and should be provided with the necessary materials in this process (Dogan et al. 2011 ). It is thought that teacher views about NOS may be improved in this way more permanently and internalized. Researches indicate that teaching the NOS by integrating it to other subjects within the scope of a specific lesson improves teachers’ professional competences about NOS (Schwartz 2009 ). In addition to providing sustained support, context-specific teaching materials were also provided to teachers in our implemented innovative NOS-CPD program. However, there are limited examples that will guide the teachers in this regard in the literature (Khishfe and Lederman 2003 ; Schwartz 2009 ). It is recommended that such exemplifying studies in this area should be increased. Certainly, providing enough time and material is not the only factor that effect CPD program’s effectiveness. Our implemented NOS-CPD program was innovated by including formative assessment and discourse analysis. These innovations are thought to enhance the success of our program. Beside this, as we know from the literature that CPD program’s quality is also affected by other factors (based on teachers’ needs, integration with other reform efforts, high-quality instruction, active engagement, enhancement of both content knowledge and pedagogical content knowledge, improvement of teacher beliefs, continued support, collaboration, reflection and feedback, evaluation procedures, and provision of local support). Therefore, it is recommended that these factors should not be ignored.
The effect of the factors mentioned above in the development of teachers’ NOS views is quite obvious in the literature. However, the main problem for teachers is that they have difficulty integrating what they learn in professional development programs into their classroom practices. It is thought that the use of formative assessment procedures during the teaching process and reinforcement of teaching with discourse analysis and support of teachers through feedback during the process will also improve the performances of the teachers in the classroom. We have also shown that the innovative NOS-CPD program that incorporates these features improved the teachers’ classroom practices. There were other issues that could be reported in this paper, but this paper focused especially on teacher’ NOS views. It is recommended that a professional development program in this context should investigate and report on the effect of teachers, especially on classroom practices.
Last of all, as Guskey ( 2007 ) emphasizes, there is a large research base on the professional development of teachers in the literature, but some of these researches are finding opposite findings. For example, while some research suggests that professional development activities should be teacher-specific and focus on daily classroom activities, some researches do not give importance to them and require more holistic and organizational approaches. Some experts state that professional development reforms must be initiated/carried out by teachers or school personnel. Others say they need guidance with a clear vision because they do not have the opportunity to think a wide variety of change and practice. Therefore, the biggest problem in determining the characteristics of successful professional development programs is trying to find a “single correct answer.” General prescriptions cannot provide much guidance to practitioners because “context” is a powerful influence. In one context, there may be a need for a managerial structure in another context, while teacher-led activities are in need. In other words, instead of “one correct answer” or “one correct path,” there is a collection of answers developed according to context. So the aim should be to find the most suitable mixture and to be aware that this mixture may change over time (Guskey 2007 , as cited in Bumen et al. 2012 ). Based on our literature searches and implementation, we may say that our innovative NOS-CPD program contains the one of the most suitable mixture for developing teachers’ professional competences about NOS. But of course, this program may be improved by experimenting in different contexts.
Abbreviations
Continuing Professional Development
Ministry of National Education of Turkey
Next Generation Science Standards in the United States
Nature of Science
Views of Nature of Science-Form C
Abd-El-Khalick, F (1998). The influence of history of science courses on students’ conceptions of the nature of science (unpublished doctoral dissertation) . Oregon: Oregon State University.
Google Scholar
Abd-El-Khalick, F, & Lederman, NG. (2000). Improving science teachers’ conceptions of nature of science: a critical review of the literature. International Journal of Science Education , 22 (7), 665–701.
Article Google Scholar
Akerson, VL, Cullen, TA, Hanson, DL. (2009). Fostering a community of practice through a professional development program to improve elementary teachers’ views of nature of science and teaching practice. Journal of Research in Science Teaching , 46 (10), 1090–1113.
Akerson, VL, Hanuscin, DL. (2007). Teaching nature of science through inquiry: Results of a 3-year professional development program. Journal of Research in Science Teaching, 44 (5), 653–680.
Bennett, RE. (2011). Formative assessment: a critical review. Assessment in Education: Principles, Policy & Practice , 18 (1), 5–25.
Bertram, A, & Loughran, J. (2012). Science teachers’ views on CoRes and PaP-eRs as a framework for articulating and developing pedagogical content knowledge. Research in Science Education , 42 (6), 1027–1047.
Boone, WJ, & Kahle, KB. (1998). Student perceptions of instruction, peer interest, and adult support for middle school science: differences by race and gender. Journal of Women and Minorities in Science and Engineering , 4 , 333–340.
Bumen, NT, Ates, A, Cakar, E, Ural, G, Acar, V. (2012). Teachers’ professional development in Turkish context: issues and suggestions. National Education , 41 (194), 31–50.
Cakmakci, G. (2012). Promoting pre-service teachers’ ideas about nature of science through educational research apprenticeship. Australian Journal of Teacher Education , 37 (2), 114–135.
Capps, DK, & Crawford, BA. (2013). Inquiry-based professional development: what does it take to support teachers in learning about inquiry and nature of science? International Journal of Science Education , 35 (12), 1947–1978.
Capps, DK, Crawford, BA, Constas, MA. (2012). A review of empirical literature on inquiry professional development: alignment with best practices and a critique of the findings. Journal of Science Teacher Education , 23 (3), 291–318.
Cobern, WW, & Loving, CC. (2001). Defining “science” in a multicultural world: implications for science education. Science Education , 85 (1), 50–67.
Cohen, DK, & Hill, HC (2001). Learning policy: when state education reform works . New Haven: Yale University Press.
Book Google Scholar
Dass, PM, & Yager, RE. (2009). Professional development of science teachers: history of reform and contributions of the STS-based Iowa Chatauqua Program. Science Education Review , 8 (3), 99–111.
Dogan, N, & Abd-El-Khalick, F. (2008). Turkish grade 10 students’ and science teachers’ conceptions of nature of science: a national study. Journal of Research in Science Teaching , 45 (10), 1083–1112.
Dogan, N, Cakiroglu, J, Cavus, S, Bilican, K, Arslan, O. (2011). Developing science teachers’ nature of science views: the effect of in-service teacher education program. Hacettepe University Journal of Education , 40 , 127–139.
Dogan, N., Cakmakci, G., Irez, S., Yalaki, Y., Erdas, E., Kaya, G., Ozer, F., Han, Tosunoglu, C., Altiner, Z.B., Bala, V.G., Ertugrul, G., Sardag, M., Koylu, N.Z., Gunsever, G. (2016). Etkinliklerle Bilimin Doğasının Öğretimi . Yalcin Yalaki (Ed.), (Genişletilmiş 2. Baskı). Pegem Akademi Yayıncılık, Ankara. http://www.pegem.net/dosyalar/dokuman/191701_ekitap.pdf . Accessed 11 Dec 2016.
Garet, MS, Porter, AC, Desimone, L, Birman, BF, Yoon, KS. (2001). What makes professional development effective? Results from a national sample of teachers. American Educational Research Journal , 38 (4), 915–945.
Gess-Newsome, J (2001). The professional development of science teachers for science education reform: a review of the research . Arlington: National Science Teachers Association Press.
Guerra-Ramos, MT, Ryder, J, Leach, J. (2010). Ideas about the nature of science in pedagogically relevant contexts: insights from a situated perspective of primary teachers’ knowledge. Science Education , 94 (2), 282–307.
Guskey, TR (2000). Evaluating professional development . Thousand Oaks: Corwin Press.
Guskey, T.R. (2007). Results-oriented professional development, A. C. Onstein, E. F. Pajak, ve S. B. Ornstein, Contemporary issues in curriculum, Pearson Education, Boston. (p. 334–346)
Guskey, TR, & Yoon, KS. (2009). What works in professional development? Phi Delta Kappan , 90 (7), 495–500.
Hayes, D. (1995). In-service teacher development: some basic principles. ELT Journal , 49 , 252–261.
Herman, BC, Clough, MP, Olson, JK. (2013). Teachers’ nature of science implementation practices 2–5 years after having completed an intensive science education program. Science Education , 97 (2), 271–309.
Irez, O. S. (2004). Turkish preservice science teacher educators’ beliefs about the nature of science and conceptualizations of science education, Unpublished PhD dissertation Nottingham University , UK .
Irez, S. (2006). Are we prepared?: an assessment of preservice science teacher educators’ beliefs about nature of science. Science Education , 90 (6), 1113–1143.
Kaya, G, Sardag, M, Cakmakci, G, Dogan, N, Irez, S, Yalaki, Y. (2016). Pattern of discourse and communicative approaches used while teaching nature of science. Education & Science , 41 (185), 83–99.
Khishfe, R, & Abd-El-Khalick, F. (2002). Influence of explicit and reflective versus implicit inquiry-oriented instruction on sixth graders’ views of nature of scientific knowledge. Journal of Research in Science Teaching , 39 (7), 551–578.
Khishfe, R, & Lederman, NG (2003). The development of students’ conceptions of nature of science. Paper presented at the annual meeting of the American Educational Research Association (AERA) . Chicago.
Koseoglu, F., Altun, Y., Unlu, P., Tumay, H., Tasar, F. (2011). Bilimin Doğası Öğretimi: Bilim Felsefesi ve Bilim Tarihine Dayanarak Bilimsel Argüman Oluşturma ve Akıl Yürütme Öğretimine Yönelik Bir Öğretmen Mesleki Gelişim Paketinin Hazırlanması Projesi. Proje No: 108K086, TUBİTAK, Ankara.
Koseoglu, F, Tumay, H, Ustun, U. (2010). Developing a professional development package for nature of science instruction and discussion about its implementation for pre-service teachers. Journal of Kirsehir Education Faculty , 4 , 129–162.
Koulaidis, V, & Ogborn, J. (1988). Use of systemic networks in the development of a questionnaire. International Journal of Science Education , 10 (5), 497–509.
Kwakman, K. (2003). Factors affecting teachers’ participation in professional learning activities. Teaching and Teacher Education , 19 (2), 149–170.
Lederman, NG. (1992). Students’ and teachers’ conceptions of the nature of science: a review of the research. Journal of Research in Science Teaching , 29 , 331–359.
Lederman, NG, Abd-El-Khalick, F, Bell, RL, Schwartz, RS. (2002). Views of the nature of science questionnaire: toward valid and meaningful assessment of learner’s conceptions of the nature of science. Journal of Research in Science Teaching , 39 (6), 497–521.
Lederman, NG, & Lederman, JS (2014). Research on teaching and learning of nature of science. In NG Lederman, SK Abell (Eds.), Handbook of research on science education , (vol. II, pp. 600–620). New York: Routledge.
Loucks-Horsley, S, & Matsumoto, C. (1999). Research on professional development for teachers of mathematics and science: the state of the scene. School Science and Mathematics , 99 , 258–271.
Marek, EA, & Methven, SB. (1991). Effects of the learning cycle upon student and classroom teacher performance. Journal of Research in Science Teaching , 28 (1), 41–53.
Mesci, G, & Schwartz, RS. (2016). Changing pre-service science teachers’ views of nature of science: why some conceptions may be more easily altered than others? Research in Science Education , 1–23. https://doi.org/10.1007/s11165-015-9503-9 .
Ministry of National Education [MONE] (2004), Ankara, Turkey.
Ministry of National Education [MONE] (2013), Ankara, Turkey.
Mortimer, EF, & Scott, PH (2003). Meaning making in secondary science classrooms. Maidenhead . UK: Open University Press.
NGSS (2013). Next generation science standards: for states, by states . Washington: National Academies Press.
Ozer, F (2014). Impact of a professional development program on 5th, 6th and 7th grade students’ views of nature of science (master dissertation) . Bolu: Abant Izzet Baysal University.
Park, H, Nielsen, W, Woodruff, E. (2013). Students’ conceptions of the nature of science: perspectives from Canadian and Korean middle school students. Science Education , 23 (5), 1169–1196.
Penuel, WR, Fishman, BJ, Yamaguchi, R, Gallagher, LP. (2007). What makes professional development effective? Strategies that foster curriculum implementation. American Educational Research Journal , 44 (4), 921–958.
Posnanski, JT. (2010). Developing understanding of the nature of science within a professional development program for in-service elementary teachers: project nature of elementary science teaching. Journal of Science Teacher Education , 21 (5), 589–621.
Putnam, RT, & Borko, H (1997). Teacher learning: implications of new views of cognition. In BJ Biddle, TL Good, IF Goodson (Eds.), International handbook of teachers and teaching , (pp. 1223–1296). Dordrecht: Kluwer.
Chapter Google Scholar
Sadler, TD, Burgin, S, McKinney, L, Ponjuan, L. (2010). Learning science through research apprenticeships: a critical review of the literature. Journal of Research in Science Teaching , 47 (3), 235–256.
Schwartz, R.S. (2009). The approach and effectiveness of integrating nature of science instruction during an undergraduate biology course. Paper presented at the International History and Philosophy in Science Teaching conference. Notre Dame, IN.
Shulman, LS. (1987). Knowledge and teaching: foundations of the new reform. Harvard Educational Review , 57 , 1–22.
Sinclair, JM, & Coulthard, RM (1975). Towards an analysis of discourse: the English used by teachers and pupils . Oxford: Oxford University Press.
Torff, B, & Sessions, D. (2008). Factors associated with teachers’ attitudes about professional development. Teacher Education Quarterly , 35 (2), 123–133.
Download references
Acknowledgements
This paper is part of the professional development project supported by The Scientific and Technological Research Council of Turkey (TUBITAK) under 111K527 project number. We would like to thank TUBITAK for this support. We would also like to thank the Ministry of National Education General Directorate of Teacher Training and Development, Bolu Directorate of National Education and teachers participated voluntarily to the project from the Bolu.
Also a part of this paper presented at the National Association for Research in Science Teaching (NARST), Baltimore, USA (2016, April).
This work was supported by the Scientific and Technical Research Council of Turkey (TUBITAK) under project numbers 111K527.
Availability of data and materials
We could not share our data because of our funder’s (Scientific and Technical Research Council of Turkey) rules.
Activity book titled “Teaching Nature of Science with Activities” is the product of this research is available at project’s web site: http://www.bilimindogasi.hacettepe.edu.tr/english.html
Author information
Authors and affiliations.
Department of Educational Sciences, Kastamonu University, Kuzeykent, 37150, Kastamonu, Turkey
Eda Erdas Kartal
Mallinson Institute for Science Education, Western Michigan University, 1903 W. Michigan Avenue, Kalamazoo, MI, 49008, USA
William W. Cobern
Department of Elementary Science Education, Abant Izzet Baysal University, Golkoy, 14280, Bolu, Turkey
Nihal Dogan
Department of Biology Education, Marmara University, Goztepe, 34722, Istanbul, Turkey
Serhat Irez
Department of Elementary Education, Hacettepe University, Beykent, 06800, Ankara, Turkey
Gultekin Cakmakci
Department of Elementary Science Education, Hacettepe University, Beykent, 06800, Ankara, Turkey
Yalcin Yalaki
Department of Educational Science, Education Faculty, Kastamonu University, City Center, 37200, Kastamonu, Turkey
You can also search for this author in PubMed Google Scholar
Contributions
EEK was primarily responsible for writing the manuscript. EEK and SI conducted the majority of the data analysis. All authors provided feedback throughout. All authors read and approved the manuscript.
Corresponding author
Correspondence to Eda Erdas Kartal .
Ethics declarations
Competing interests.
The authors declare that they have no competing interests.
Publisher’s Note
Springer Nature remains neutral with regard to jurisdictional claims in published maps and institutional affiliations.
Rights and permissions
Open Access This article is distributed under the terms of the Creative Commons Attribution 4.0 International License ( http://creativecommons.org/licenses/by/4.0/ ), which permits unrestricted use, distribution, and reproduction in any medium, provided you give appropriate credit to the original author(s) and the source, provide a link to the Creative Commons license, and indicate if changes were made.
Reprints and permissions
About this article
Cite this article.
Erdas Kartal, E., Cobern, W.W., Dogan, N. et al. Improving science teachers’ nature of science views through an innovative continuing professional development program. IJ STEM Ed 5 , 30 (2018). https://doi.org/10.1186/s40594-018-0125-4
Download citation
Received : 15 February 2018
Accepted : 29 May 2018
Published : 17 July 2018
DOI : https://doi.org/10.1186/s40594-018-0125-4
Share this article
Anyone you share the following link with will be able to read this content:
Sorry, a shareable link is not currently available for this article.
Provided by the Springer Nature SharedIt content-sharing initiative
- Nature of science
- Professional development
- Teacher education
- Science education
- Skip to primary navigation
- Skip to main content
- Skip to primary sidebar
- Skip to footer

Understanding Science
How science REALLY works...
- Understanding Science 101
- Misconceptions
- Testing ideas with evidence from the natural world is at the core of science.
- Scientific testing involves figuring out what we would expect to observe if an idea were correct and comparing that expectation to what we actually observe.
- Scientific arguments are built from an idea and the evidence relevant to that idea.
- Scientific arguments can be built in any order. Sometimes a scientific idea precedes any evidence relevant to it, and other times the evidence helps inspire the idea.
Misconception: Science proves ideas.
Misconception: Science can only disprove ideas.
Correction: Science neither proves nor disproves. It accepts or rejects ideas based on supporting and refuting evidence, but may revise those conclusions if warranted by new evidence or perspectives. Read more about it.
The core of science: Relating evidence and ideas
In this case, the term argument refers not to a disagreement between two people, but to an evidence-based line of reasoning — so scientific arguments are more like the closing argument in a court case (a logical description of what we think and why we think it) than they are like the fights you may have had with siblings. Scientific arguments involve three components: the idea (a hypothesis or theory), the expectations generated by that idea (frequently called predictions), and the actual observations relevant to those expectations (the evidence). These components are always related in the same logical way:
- What would we expect to see if this idea were true (i.e., what is our expected observation)?
- What do we actually observe?
- Do our expectations match our observations?
PREDICTIONS OR EXPECTATIONS?
When scientists describe their arguments, they frequently talk about their expectations in terms of what a hypothesis or theory predicts: “If it were the case that smoking causes lung cancer, then we’d predict that countries with higher rates of smoking would have higher rates of lung cancer.” At first, it might seem confusing to talk about a prediction that doesn’t deal with the future, but that refers to something going on right now or that may have already happened. In fact, this is just another way of discussing the expectations that the hypothesis or theory generates. So when a scientist talks about the predicted rates of lung cancer, he or she really means something like “the rates that we’d expect to see if our hypothesis were correct.”
If the idea generates expectations that hold true (are actually observed), then the idea is more likely to be accurate. If the idea generates expectations that don’t hold true (are not observed), then we are less likely to accept the idea. For example, consider the idea that cells are the building blocks of life. If that idea were true, we’d expect to see cells in all kinds of living tissues observed under a microscope — that’s our expected observation. In fact, we do observe this (our actual observation), so evidence supports the idea that living things are built from cells.
Though the structure of this argument is consistent (hypothesis, then expectation, then actual observation), its pieces may be assembled in different orders. For example, the first observations of cells were made in the 1600s, but cell theory was not postulated until 200 years later — so in this case, the evidence actually helped inspire the idea. Whether the idea comes first or the evidence comes first, the logic relating them remains the same.
Here, we’ll explore scientific arguments and how to build them. You can investigate:
Putting the pieces together: The hard work of building arguments
- Predicting the past
- Arguments with legs to stand on
Or just click the Next button to dive right in!
- Take a sidetrip
- Teaching resources
Scientific arguments rely on testable ideas. To learn what makes an idea testable, review our Science Checklist .
- Forming hypotheses — scientific explanations — can be difficult for students. It is often easier for students to generate an expectation (what they think will happen or what they expect to observe) based on prior experience than to formulate a potential explanation for that phenomena. You can help students go beyond expectations to generate real, explanatory hypotheses by providing sentence stems for them to fill in: “I expect to observe A because B.” Once students have filled in this sentence you can explain that B is a hypothesis and A is the expectation generated by that hypothesis.
- You can help students learn to distinguish between hypotheses and the expectations generated by them by regularly asking students to analyze lecture material, text, or video. Students should try to figure out which aspects of the content were hypotheses and which were expectations.
Summing up the process
Subscribe to our newsletter
- The science flowchart
- Science stories
- Grade-level teaching guides
- Teaching resource database
- Journaling tool
We're sorry but you will need to enable Javascript to access all of the features of this site.
Stanford Online
21st-century teaching & learning: data science.
Stanford Graduate School of Education
This course is designed to help anyone learn and teach with a 21st-century approach to knowledge and teaching. We hope the ideas will help you as a person and help you learn new teaching ideas to share with learners. The course includes a focus on data literacy, and shares content knowledge and teaching ideas for that area. If you are having thoughts of "I don’t know a lot about data" or "That sounds very maths-ey and I don’t like maths," don't worry -- this course is designed to be accessible to you. Whether you are a kindergarten teacher, a high school history or maths teacher, an administrator or parent, or someone just curious about data, there will be ideas for you. By taking this course, you are agreeing to participate in studies that we may conduct as part of our ongoing research into online learning.
Topics Include
- The limitless learning potential of all students and ways to communicate this information to students
- The nature of our data-filled world and the meaning of data science
- The importance of data literacy for all students
- Data analysis tools and different ways to analyze and visualize data
- The cross-curricular nature of data literacy and learning
- How to develop exciting data-filled lessons for all students
Who Should Enroll?
Teachers (K-12) or Teachers in training, Principals, Administrators, District Leaders, Instructional Coaches, School support staff, Superintendents, Policy Makers
What is the course pace?
The course will be self-paced, you can start and end the course at any time.
- $149 per person
- Group enrollment is available by purchase order, company check, credit card, or wire transfer by emailing [email protected] .
- A discounted rate is available for groups of 150 or more, at $119 per person. Please contact [email protected] for more information on groups of 150 people or more
Continuing Education Units
By completing this course, you’ll earn 3 Continuing Education Units (CEUs). CEUs cannot be applied toward any Stanford degree. CEU transferability is subject to the receiving institution’s policies.
Record of Completion
When you complete the course, you’ll receive an email with a link to download your Record of Completion. This email will be sent to the address you provide in your mystanfordconnection within 1 week of course completion.
Extending Access
If you are interested in extending access to your course for another year, please re-enroll and then send your confirmation email to [email protected] .
Please contact [email protected]
Core Competencies
- Data Science in the World
- Teaching Data Literacy
- Data Visualizations
- Mathematics of Data Science
- Engineering
- Computer Science & Security
- Business & Management
- Energy & Sustainability
- Data Science
- Medicine & Health
- Explore All
- Technical Support
- Master’s Application FAQs
- Master’s Student FAQs
- Master's Tuition & Fees
- Grades & Policies
- Graduate Application FAQs
- Graduate Student FAQs
- Graduate Tuition & Fees
- Community Standards Review Process
- Academic Calendar
- Exams & Homework FAQs
- Enrollment FAQs
- Tuition, Fees, & Payments
- Custom & Executive Programs
- Free Online Courses
- Free Content Library
- School of Engineering
- Graduate School of Education
- Stanford Doerr School of Sustainability
- School of Humanities & Sciences
- Stanford Human Centered Artificial Intelligence (HAI)
- Graduate School of Business
- Stanford Law School
- School of Medicine
- Learning Collaborations
- Stanford Credentials
- What is a digital credential?
- Grades and Units Information
- Our Community
- Get Course Updates


Learning Materials Utilizing Sustainability Pedagogy in Grade 8 Ecology
- Esmeth Espinola Bicol University, Southern Luzon State University
Science education in the Philippines is anchored on the United Nations (UN) goals on sustainability education, as provided in K to 12 Science Curriculum, which envisions learners to be environmentally literate, critical problem solvers, and responsible stewards of nature. However, K to 12 implementations in the Philippines faced several challenges that resulted in student’s poor awareness and knowledge about Philippine wildlife and conservation, as well as low proficiency in science. In order to enhance the proficiency and competence of students in ecology, learning materials utilising sustainability pedagogy were developed. A Descriptive-developmental research method was used to evaluate the developed learning materials (DLMs) and describe the experiences of students in the DLMs. A Four-Point Likert Scale technique was employed to evaluate the DLMs. structured journaling and thematic analysis were used to determine the experiences of students in using the DLMs in ecology. The experts’ evaluation showed that the five DLMs utilising sustainability pedagogy in grade 8 ecology passed the criteria of DepEd (Department of Education) as stipulated in the Learning Resources Management and Development System (LRMDS). Introduced features, sustainability-themed, issue-based, contextualised, and reflective likewise obtained very satisfactory result. Students found the DLMs interesting, comprehensible, promote awareness, relevant to the community’s environmental issues, and helped them to express their opinions. The study recommended that the valid and modified DLMs utilising sustainability pedagogy may be used by biology teachers in their lessons, can still be improved and further contextualised as well as used in other topics in ecology and environmental science .
Copyright (c) 2024 SEAQIS Journal of Science Education

This work is licensed under a Creative Commons Attribution 4.0 International License .
Make a Submission
Journal template.
Download Template
Information
- For Readers
- For Authors
- For Librarians
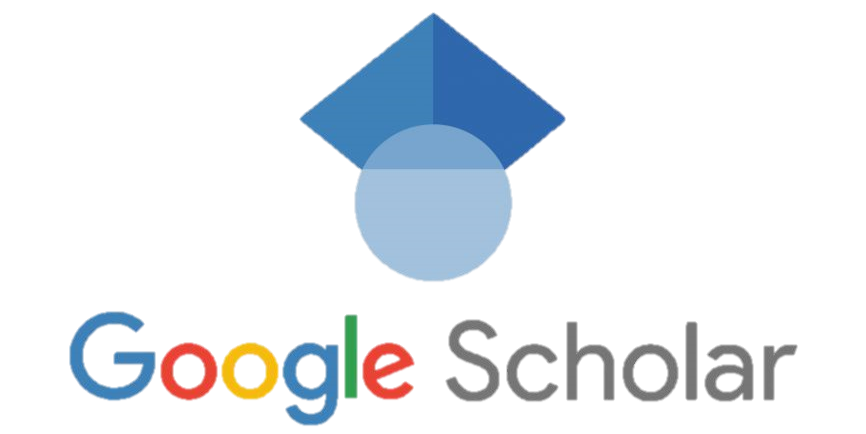
Plagiarism Checker
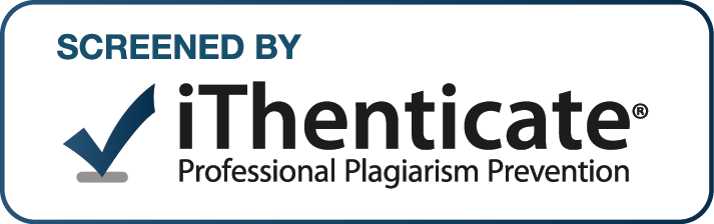
Supported by
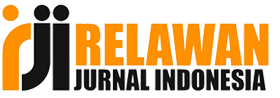
Journal Visitors
View My Stat
SEAMEO Regional Centre for QITEP in Science
Kompleks Balai Besar Guru Penggerak (BBGP) Provinsi Jawa Barat
Gedung B, Jl. Dr. Cipto No.9 Bandung 40171
West Java - Indonesia
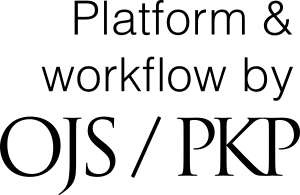
Layers of Earth's Atmosphere
Earth's atmosphere is composed of a series of layers, each with its own specific traits. Moving upward from ground level, these layers are called the troposphere, stratosphere, mesosphere, thermosphere, and exosphere. The exosphere gradually fades away into the realm of interplanetary space.
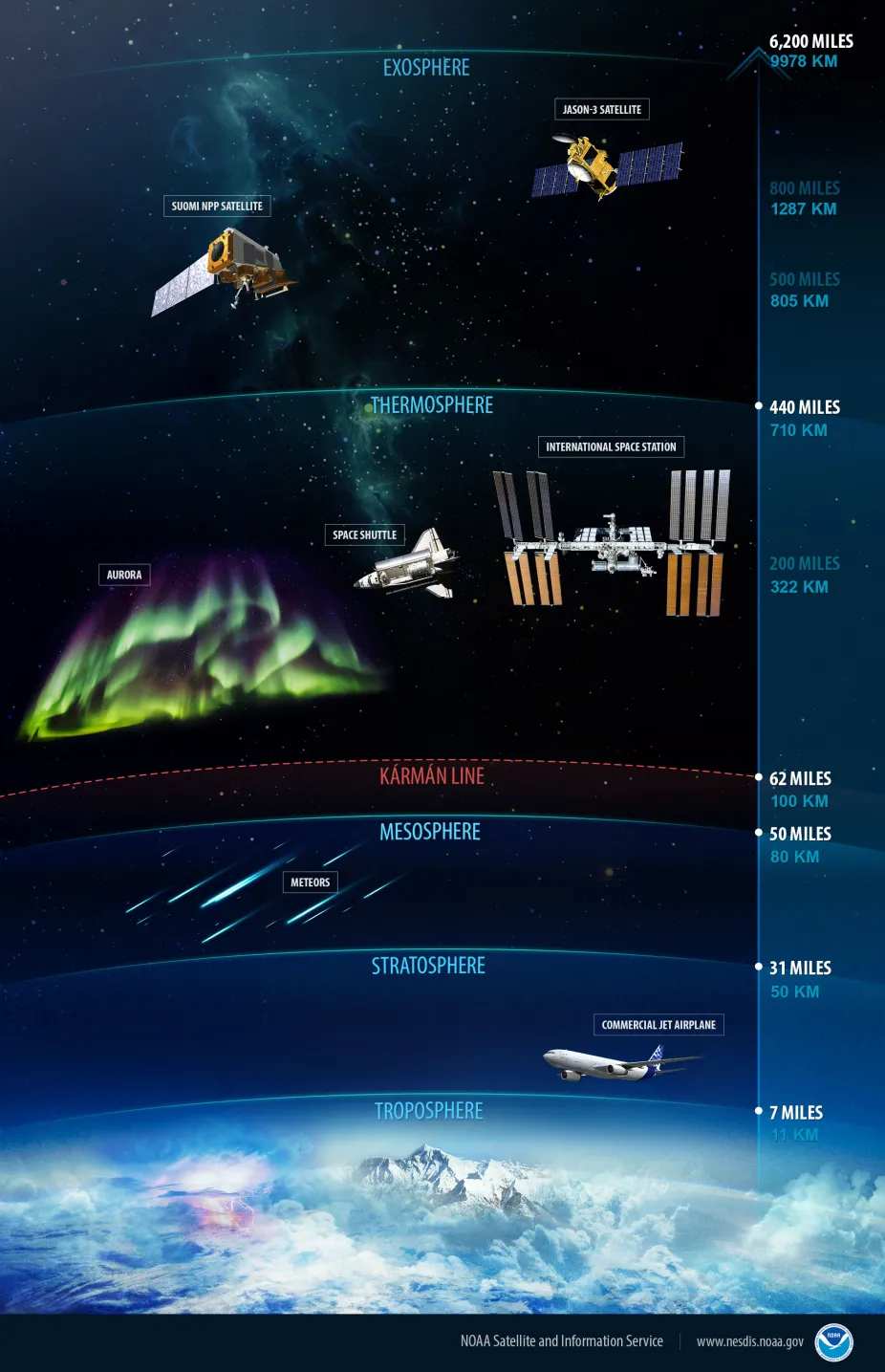
The layers of the atmosphere: the troposphere, stratosphere, mesosphere, thermosphere, and exosphere.
- Troposphere
The troposphere is the lowest layer of our atmosphere. Starting at ground level, it extends upward to about 10 km (6.2 miles or about 33,000 feet) above sea level. We humans live in the troposphere, and nearly all weather occurs in this lowest layer. Most clouds appear here, mainly because 99% of the water vapor in the atmosphere is found in the troposphere. Air pressure drops, and temperatures get colder, as you climb higher in the troposphere .
- Stratosphere
The next layer up is called the stratosphere . The stratosphere extends from the top of the troposphere to about 50 km (31 miles) above the ground. The infamous ozone layer is found within the stratosphere. Ozone molecules in this layer absorb high-energy ultraviolet (UV) light from the Sun, converting the UV energy into heat. Unlike the troposphere, the stratosphere actually gets warmer the higher you go! That trend of rising temperatures with altitude means that air in the stratosphere lacks the turbulence and updrafts of the troposphere beneath. Commercial passenger jets fly in the lower stratosphere, partly because this less-turbulent layer provides a smoother ride. The jet stream flows near the border between the troposphere and the stratosphere.
Above the stratosphere is the mesosphere . It extends upward to a height of about 85 km (53 miles) above our planet. Most meteors burn up in the mesosphere. Unlike the stratosphere, temperatures once again grow colder as you rise up through the mesosphere. The coldest temperatures in Earth's atmosphere, about -90° C (-130° F), are found near the top of this layer. The air in the mesosphere is far too thin to breathe (the air pressure at the bottom of the layer is well below 1% of the pressure at sea level and continues dropping as you go higher).
- Thermosphere
The layer of very rare air above the mesosphere is called the thermosphere . High-energy X-rays and UV radiation from the Sun are absorbed in the thermosphere, raising its temperature to hundreds or at times thousands of degrees. However, the air in this layer is so thin that it would feel freezing cold to us! In many ways, the thermosphere is more like outer space than a part of the atmosphere. In fact, the approximate boundary between our atmosphere and outer space, known as the Kármán Line, is in the thermosphere, at an altitude of about 100 km. Many satellites actually orbit Earth within the thermosphere! Variations in the amount of energy coming from the Sun exert a powerful influence on both the height of the top of this layer and the temperature within it. Because of this, the top of the thermosphere can be found anywhere between 500 and 1,000 km (311 to 621 miles) above the ground. Temperatures in the upper thermosphere can range from about 500° C (932° F) to 2,000° C (3,632° F) or higher.
Although some experts consider the thermosphere to be the uppermost layer of our atmosphere, others consider the exosphere to be the actual "final frontier" of Earth's gaseous envelope. As you might imagine, the "air" in the exosphere is very, very, very thin, making this layer even more space-like than the thermosphere. In fact, the air in the exosphere is constantly - though very gradually - "leaking" out of Earth's atmosphere into outer space. There is no clear-cut upper boundary where the exosphere finally fades away into space. Different definitions place the top of the exosphere somewhere between 100,000 km (62,000 miles) and 190,000 km (120,000 miles) above the surface of Earth. The latter value is about halfway to the Moon!
The ionosphere is not a distinct layer like the others mentioned above. Instead, the ionosphere is a series of regions in parts of the mesosphere and thermosphere where high-energy radiation from the Sun has knocked electrons loose from their parent atoms and molecules. The electrically charged atoms and molecules that are formed in this way are called ions, giving the ionosphere its name and endowing this region with some special properties. The aurora, or Northern Lights and Southern Lights, occur in the parts of the thermosphere that correspond to layers of the ionosphere.
- Earth's Atmosphere (overview)
Related Links
- Explore a Mural of the Atmosphere
- Change in the Atmosphere with Altitude
- Virtual Ballooning Simulation
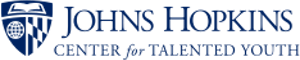
Discover, experience, and achieve with CTY
The Center for Talented Youth, a nonprofit academic center of Johns Hopkins University, delivers academic excellence and transformational experiences to advanced learners in grades 2-12.
Founded in 1979, CTY fosters intellectual growth in exceptional students from all backgrounds and communities. As a world leader in gifted education, advocacy, and research, CTY provides:
- Accredited, advanced online and on-campus courses often not found in school
- Personalized instruction
- An inclusive community of peers who share a passion for learning
Advanced students thrive when they accelerate their learning, challenge themselves, and follow their curiosity in a safe, supportive environment. So, whether you’re looking to get ahead in your studies, progress in a subject you love, or find a community where you can be yourself, there’s a CTY experience to help you meet your goals. Learn more about our mission and join us today.
Join CTY Learn More
Now Enrolling!
- On-Campus Summer Programs at sites across the U.S. After May 31, 2024 , registration is available upon request pending eligibility and seat availability. To request placement, email [email protected] after submitting a program application.
- 150+ Online courses available worldwide.
Take charge of your learning online, or immerse yourself in summer academics. Our programs deliver rigorous and exciting coursework led by attentive, world-class educators.
Online Programs
Find real-time, self-paced, session-based, and individually paced courses that fit your learning style and schedule.
On-Campus Summer Programs
Join a community of young scholars tackling exciting and challenging subjects.
Talent Search and Testing
Identify the extraordinary academic skills of your young scholar through CTY's variety of above-grade level assessments.
Not sure where to start? We can help.
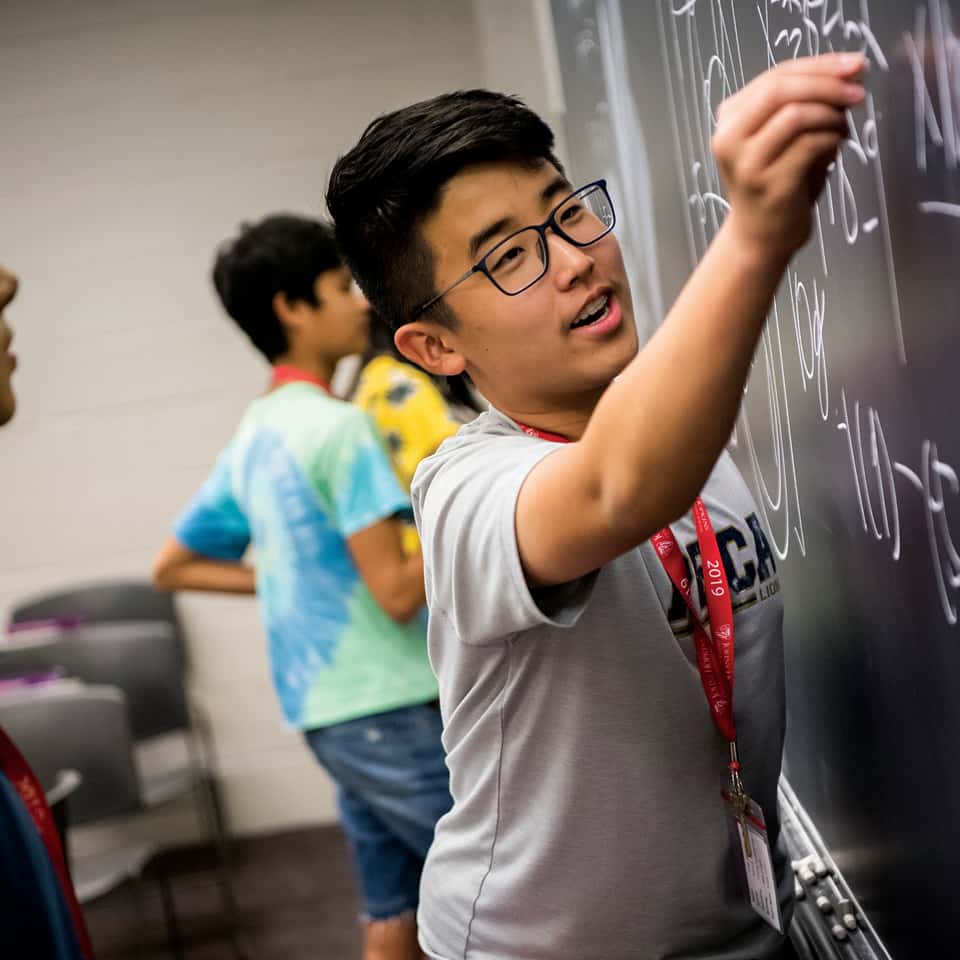
At CTY, I am intellectually stimulated and I can think at higher levels and strive to achieve the best. Everyone seems incredibly different in the beginning, but we are all so similar by the end because we are given a place to be ourselves.
CTY On-Campus Programs Student
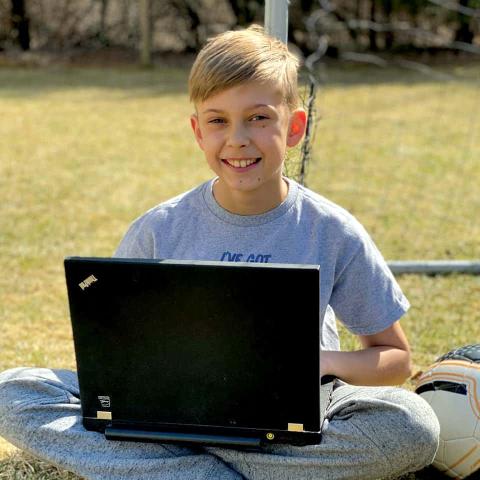
The CTY Online Programs are an excellent addition to my children's regular academic course work. The convenience of taking high quality courses from virtually any location is a tremendous advantage.
CTY Online Programs Parent
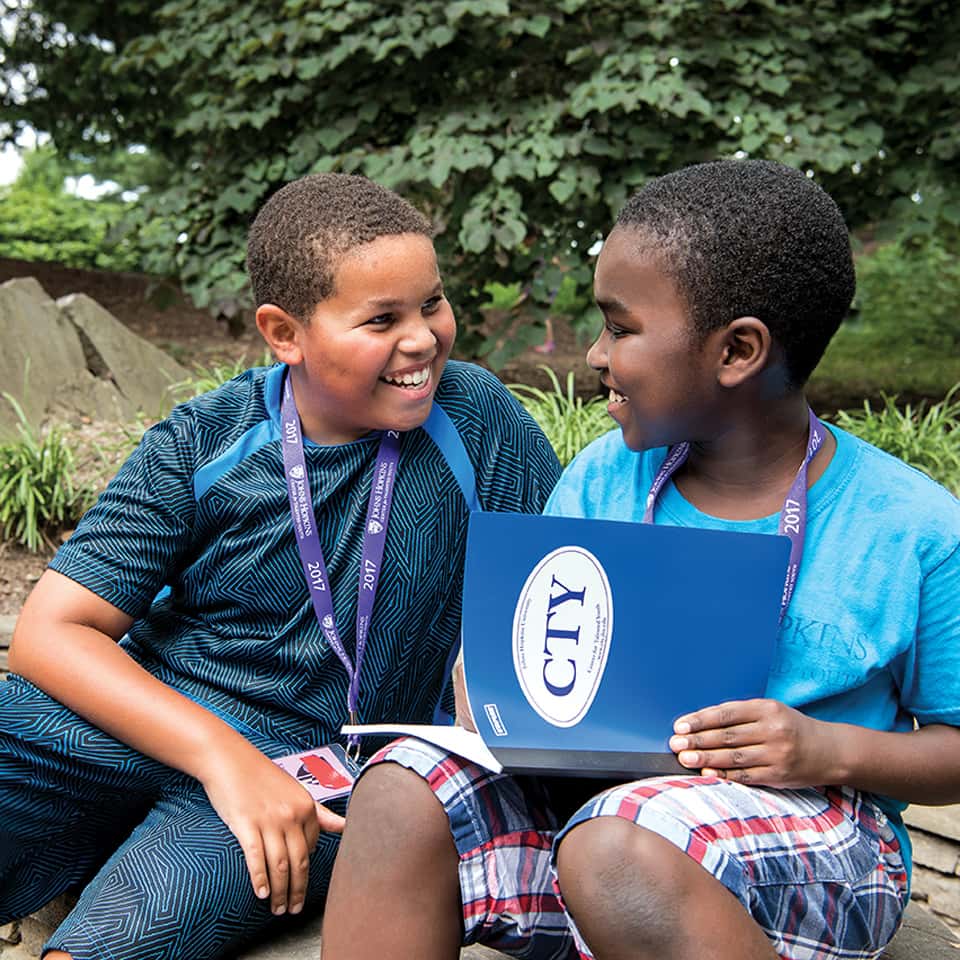
The teacher and teaching assistant were beyond exceptional. The activities, campus community, and unique CTY customs made him feel like a part of a secret society. They foster and embrace individuality while and cultivating academic excellence. This was a life-changing experience for my son.
CTY On-Campus Programs Parent
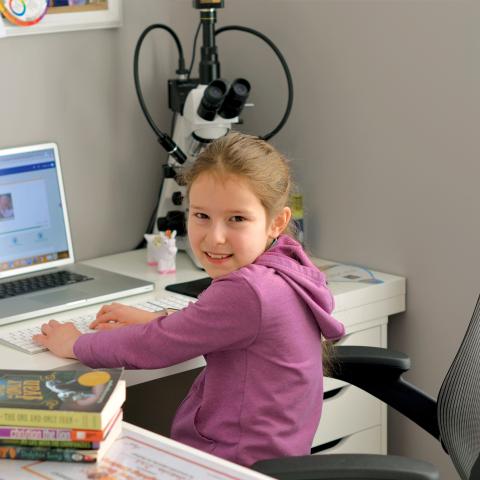
CTY has saved our bright kids from boredom, made it possible for our eldest to become an extremely accomplished musician, and helped our youngest continue to be a joyful learner when most educational experiences haven't been challenging enough.
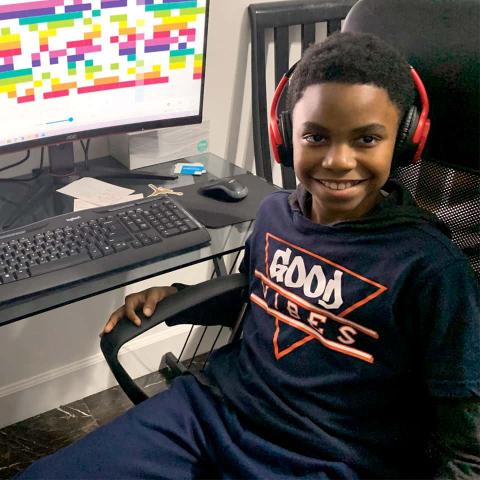
My child became self-reliant in learning, taking tests and following timeliness. It helped him build self-confidence while increasing his true knowledge about the subject.
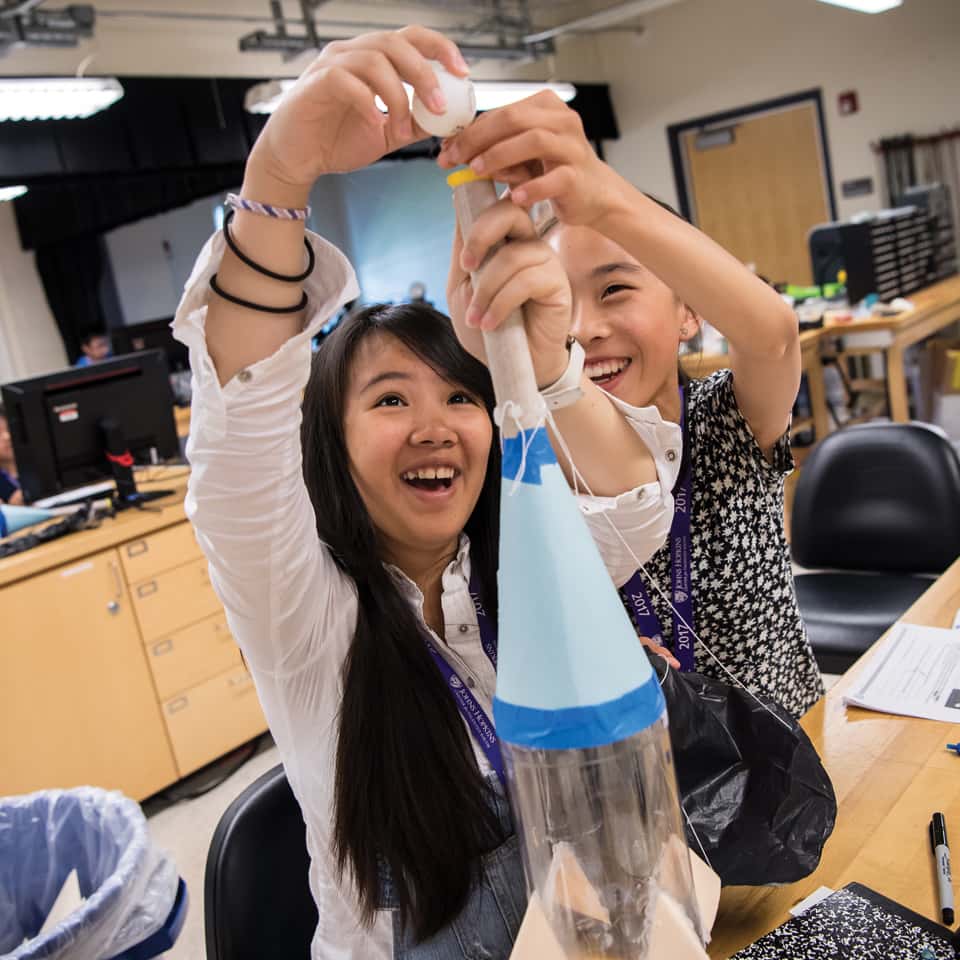
What I love about CTY is that you feel like you're in this bubble of learning and growth surrounded by kids just like you who are just as curious about the world as you are. You make friends and memories that will last you a lifetime.
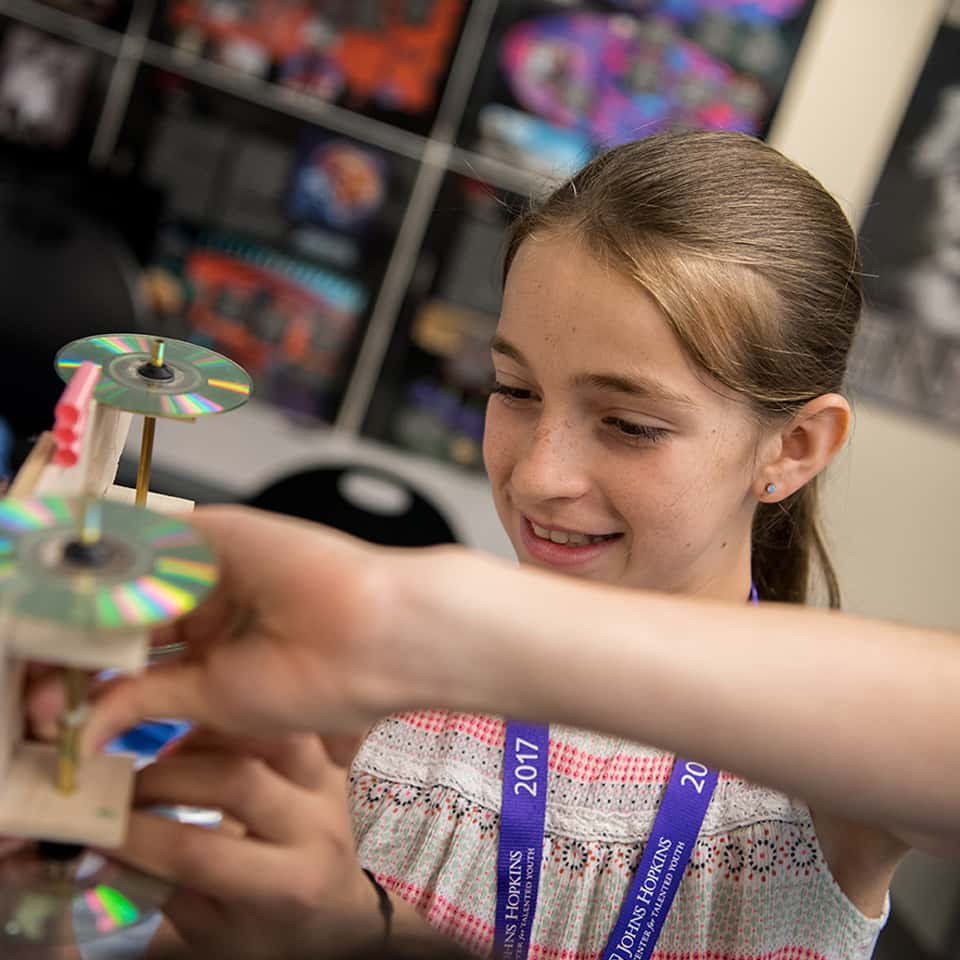
For our daughter, it was the realization that it’s cool and feminine to want to be a scientist. There’s nothing like 25 girls in a college dorm together taking number theory and astrophysics. At CTY, she feels like she’s ‘among her people.’
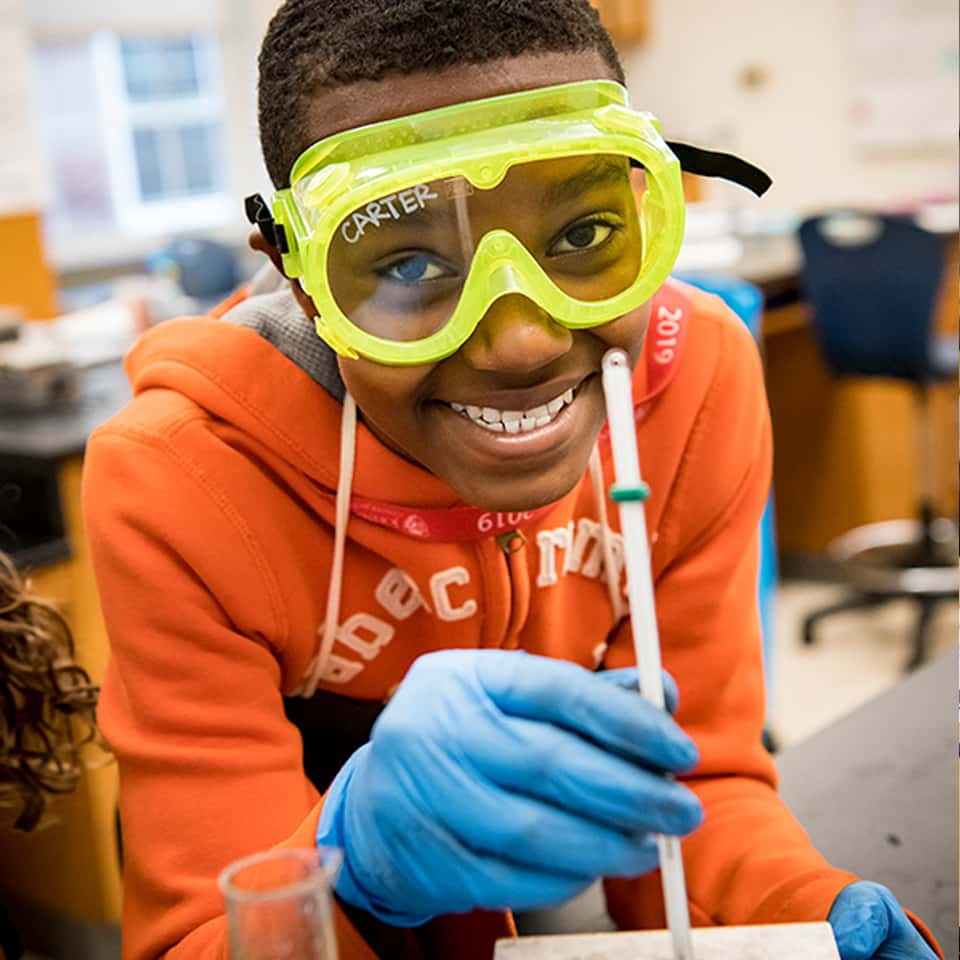
The impact my years at CTY have had on me are indescribable. Somewhere between the vast knowledge I gained in class and the unbreakable bonds I formed with other students, I was able to discover and accept who I am.
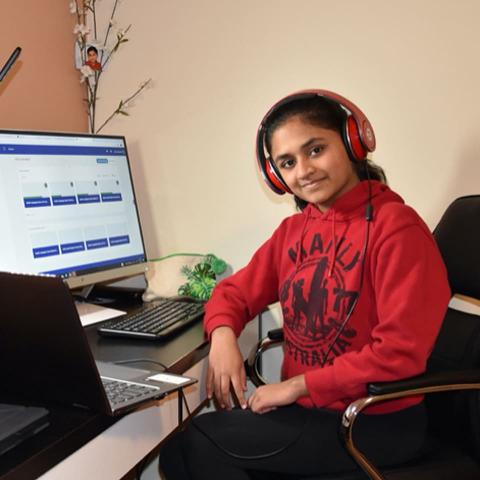
The intellectual stimulation provided by the instructor, thought provoking assignments, and comprehensive feedback have collectively ensured that my child has enjoyed every bit of her time with the CTY program.
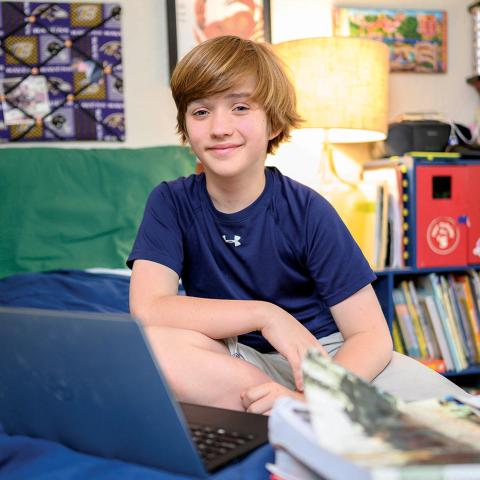
Online programs offer tremendous academic value that can be accessed from anywhere, anytime. This is exactly what we have been looking for. My children love the learning environment including both the classes themselves, and also the social programs.
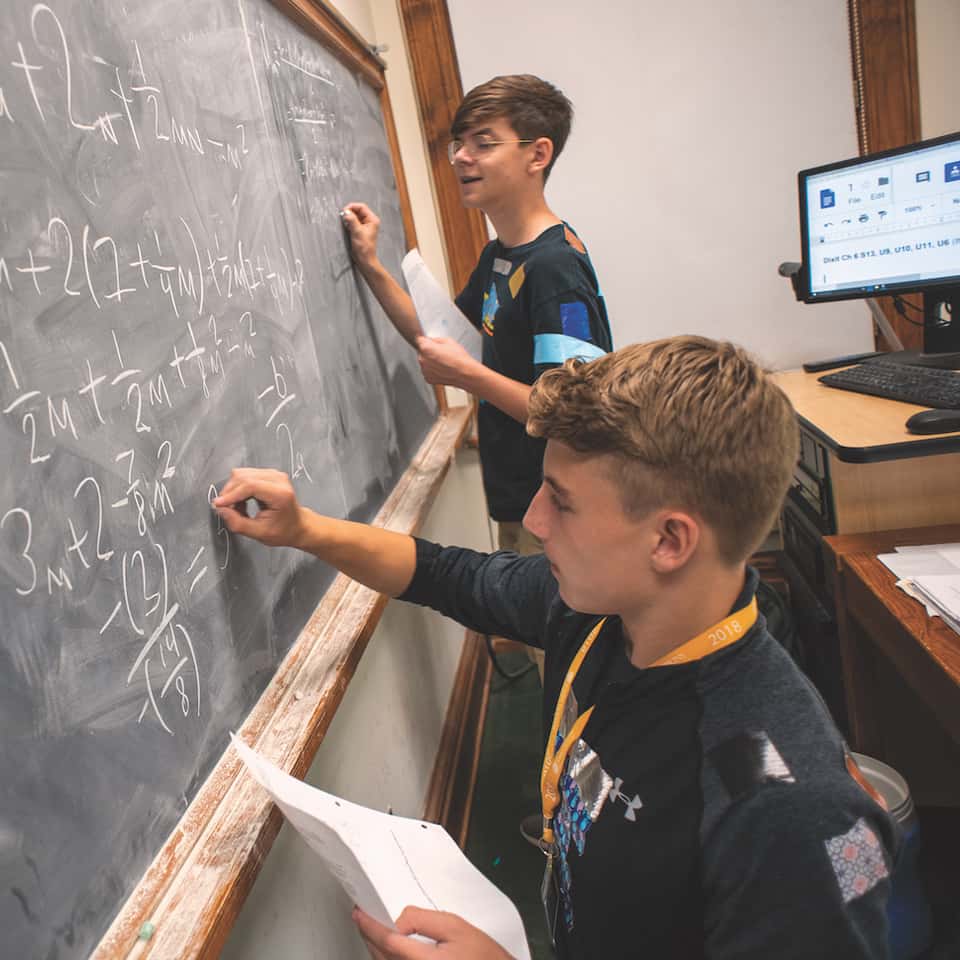
His teacher was amazed after he went to CTY last year. He got straight A’s. He’s going to be in a high honors class, and CTY has helped him prepare for that. He used to be like, ‘Mom I don’t want to do that.’ Now he’s like, ‘Mom, I think I’m ready.'
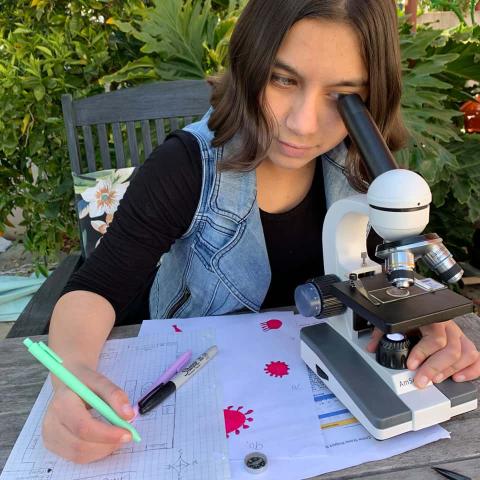
Maggie has now taken more than 15 CTY courses. She has grown exponentially, thinks well independently, uses and schedules her time wisely, and has gained boundless confidence as a result of her CTY courses.
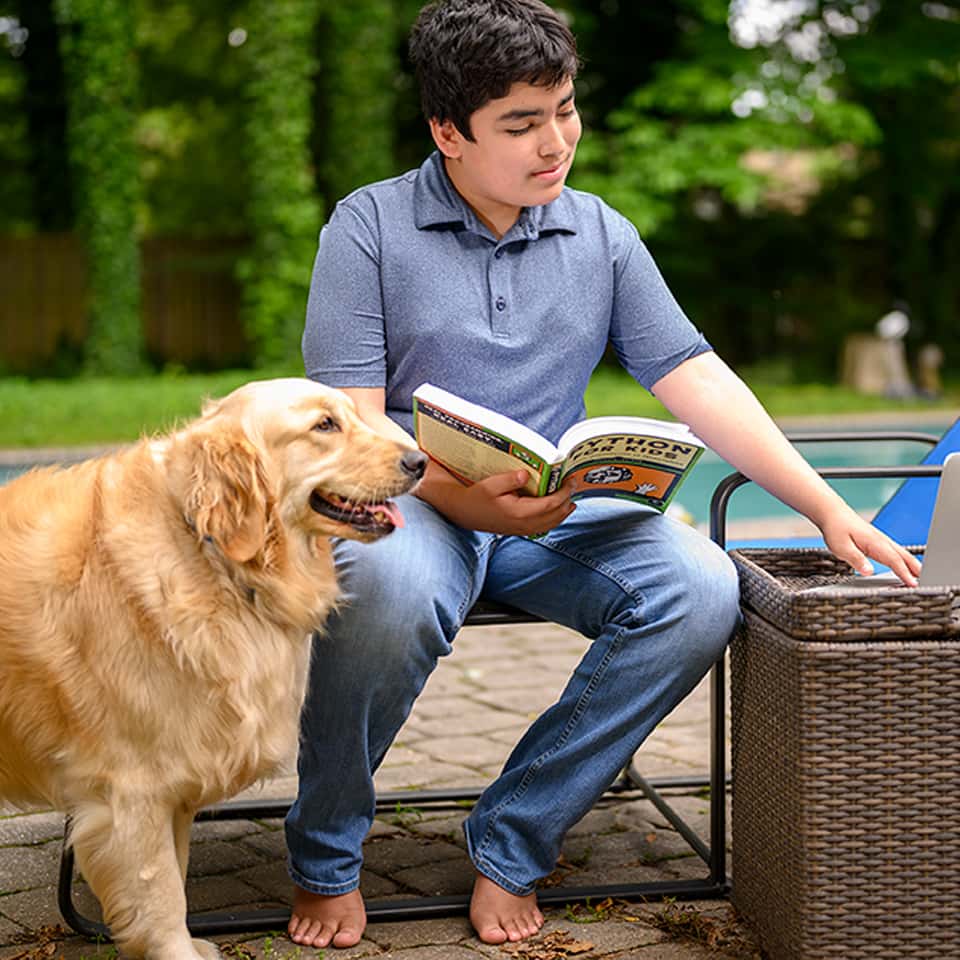
CTY provides me the opportunity to take advanced classes that are not offered at my school. I like the individually paced option because it gives me the flexibility to balance my regular schoolwork with other activities.
CTY Online Programs Student
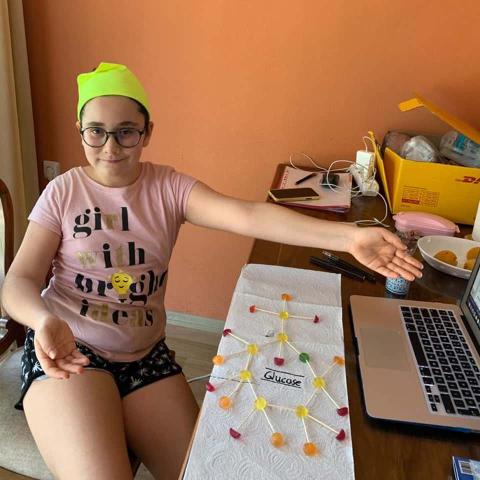
The level of academic rigor exceeds anything my daughter has experienced in school to date. In fact, this year she's homeschooling with CTY as her main resource.
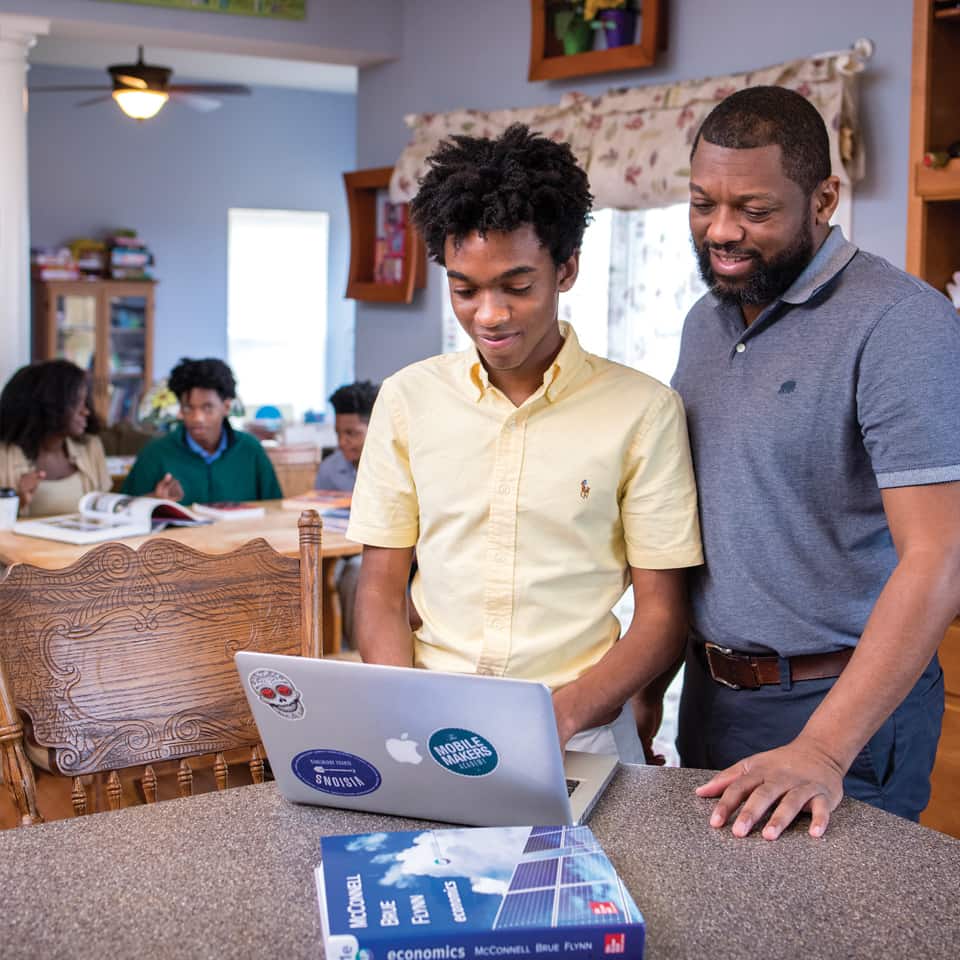
The instructors are wonderful. They give you feedback on how you can improve, and highlight what you did well. The live sessions are also a place where you can share your work, and sometimes there is a student chat lounge where you can just chat about whatever you want.
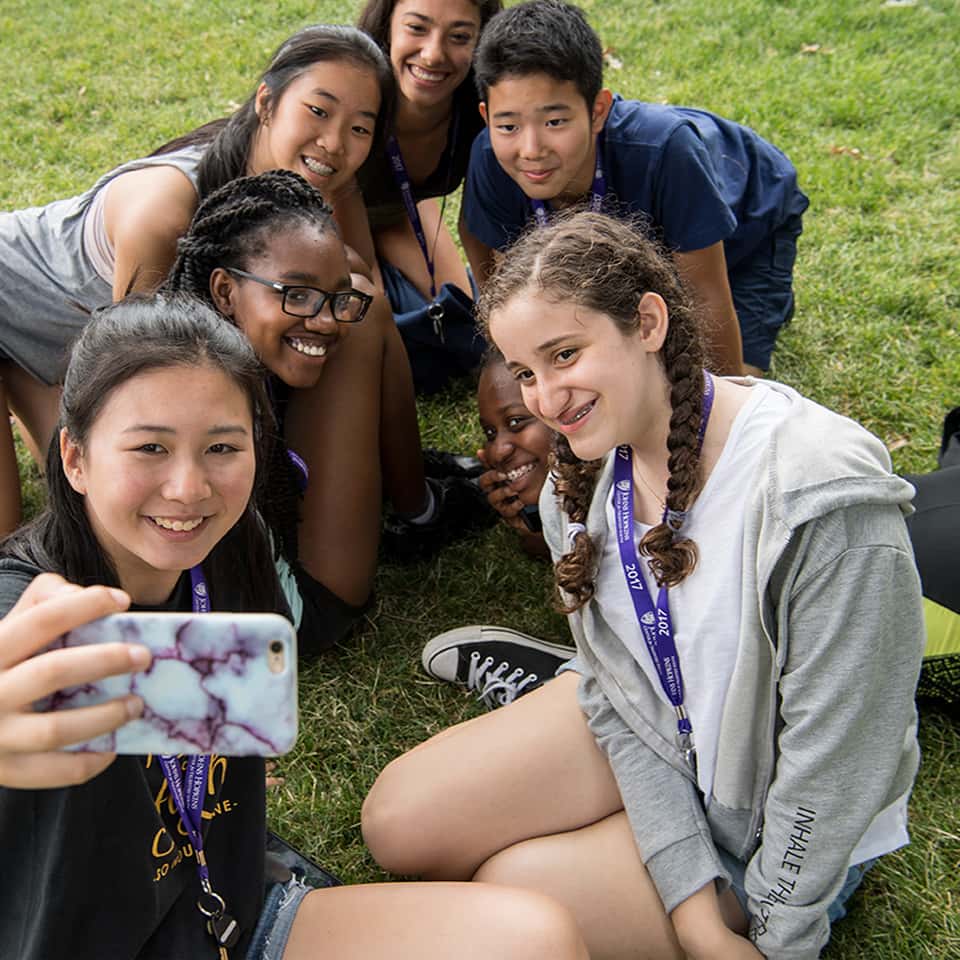
I learned not only about writing, which my instructors made more interesting than I could have ever expected, but also about the value of friendship, kindness, and teamwork. This camp truly changed who I was for the better.
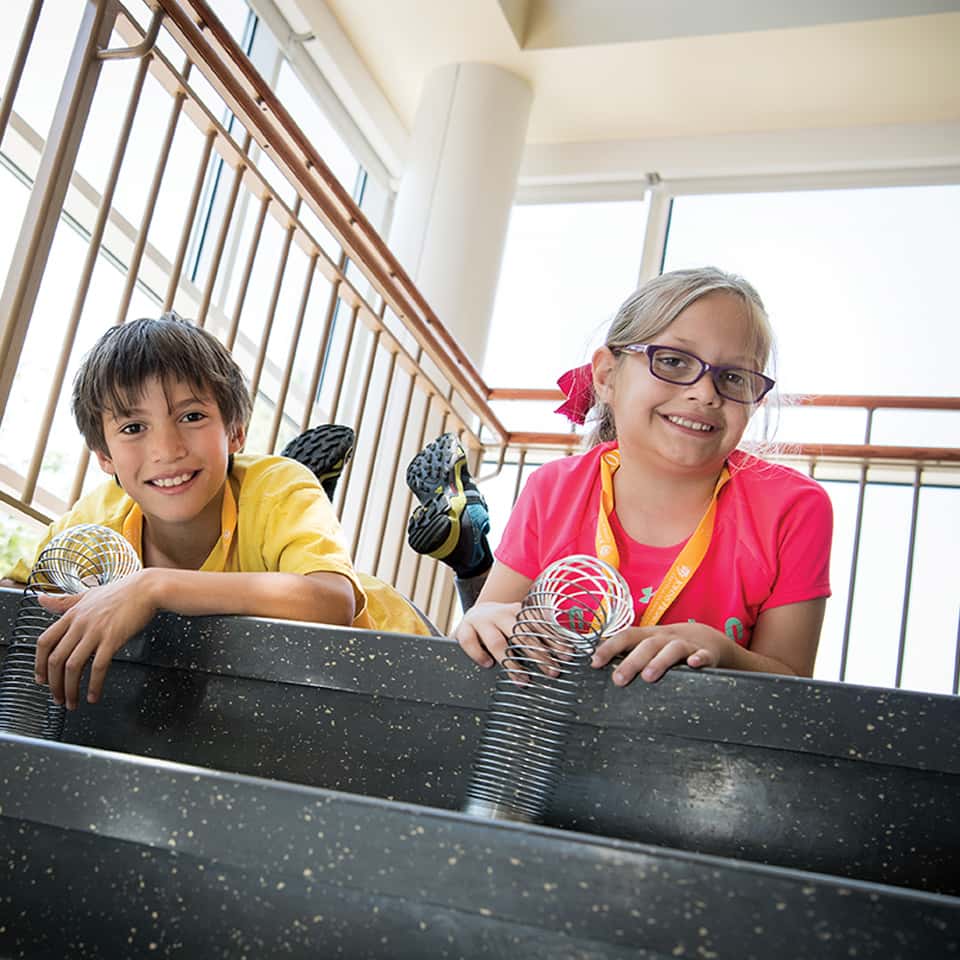
CTY has given Samuel much-needed encouragement and academic inspiration, and opened his eyes to many new opportunities. Each day was like a whole new world.
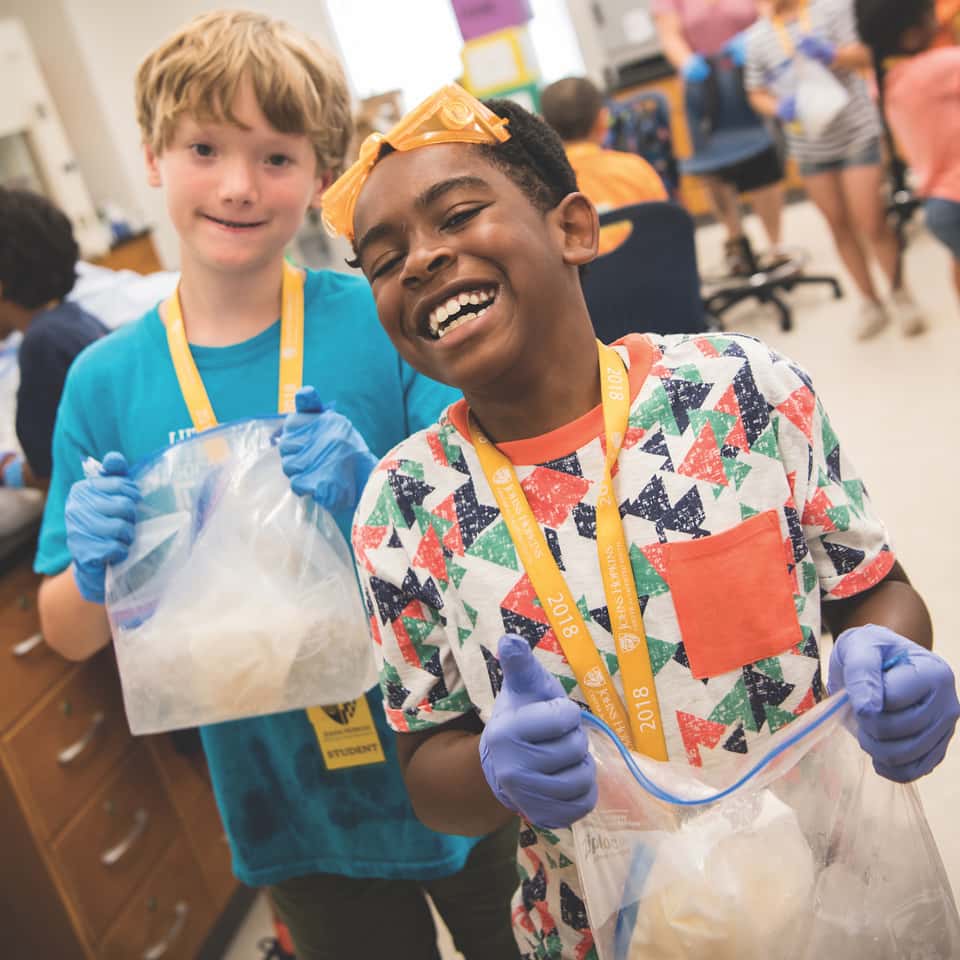
Our son loves to learn because of programs like CTY. Enriching curricula and friendly teachers and staff create an open, welcoming atmosphere to explore and grow. Thank you!
Kimberly A.
Latest at cty, cty program for bright baltimore city schools students turns 10.
The Johns Hopkins Center for Talented Youth recently celebrated a happy milestone: The CTY Baltimore Emerging Scholars Program turned 10. Baltimore City Public School elementary students in the free, school-based enrichment program celebrated with their families on Johns Hopkins University's Homewood campus May 19 with a certificate ceremony, guest speakers, a photo booth, and cupcakes. Founded in 2014, Emerging Scholars brings innovative, hands-on coursework to 400 city schools students with high academic potential annually. Read the article on the JHU Hub .
Our dedication to supporting young scholars goes far beyond the classroom. Since CTY first began in 1979, we’ve been at the forefront of research in gifted education—equipping educators to better identify, serve, and challenge their most advanced students.
Support for Educators
Interested in working with us? We provide a variety of resources and assistance for educators and institutions alike: identifying exceptional students, integrating CTY coursework, offering professional development, and much more.
Thank you for visiting nature.com. You are using a browser version with limited support for CSS. To obtain the best experience, we recommend you use a more up to date browser (or turn off compatibility mode in Internet Explorer). In the meantime, to ensure continued support, we are displaying the site without styles and JavaScript.
- View all journals
- Explore content
- About the journal
- Publish with us
- Sign up for alerts
- NATURE INDEX
- 05 June 2024
Chinese science still has room to grow
Strong potential.
Chemistry and physical sciences are clear areas of focus for China, accounting for 85% of the country’s total Share in the Nature Index in 2023*. But output in other subjects is growing fast. China’s adjusted Share in biological sciences increased by 15.8% from 2022 to 2023* — the highest percentage among the four natural-sciences subjects shown below.
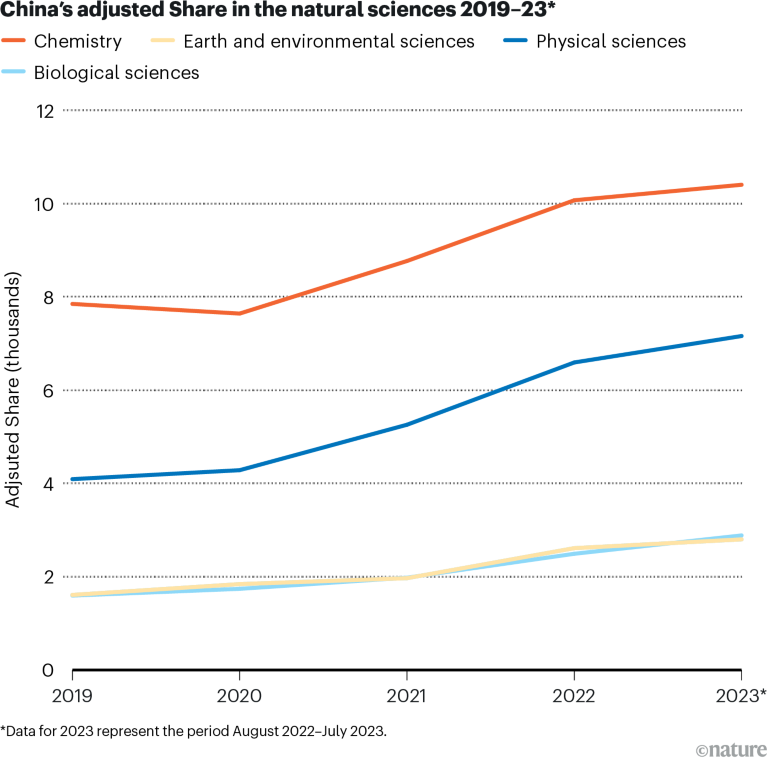
Source: Nature Index. Analysis by Bo Wu. Infographic by Simon Baker, Bec Crew and Tanner Maxwell
Topic trends
The top fields of research (FORs) in each of the five subjects tracked by Nature Index are shown. The most dominant FORs across the respective areas are biochemistry and cell biology, at 36% of biological-sciences output, and materials engineering, which represents 34.7% of physical-sciences output. FORs can relate to more than one subject: biochemistry and cell biology is also among the top five FORs for health sciences, for instance.
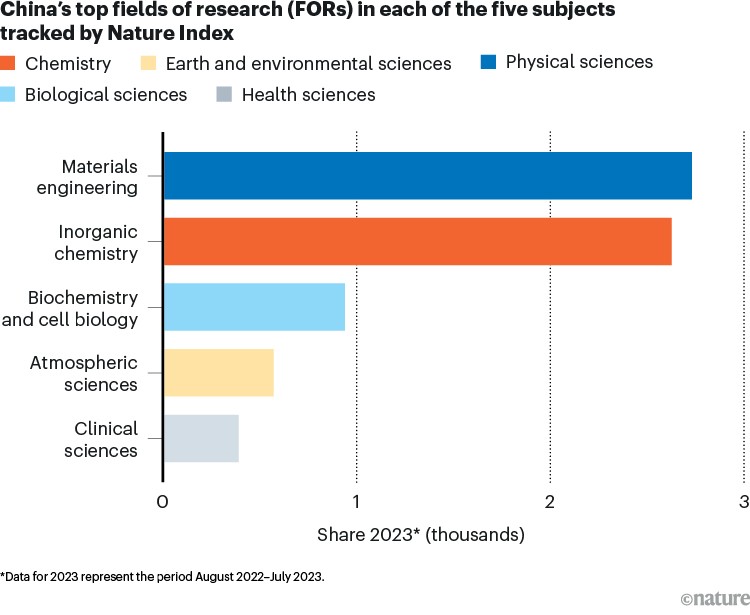
Looking outwards
China’s areas of relative weakness have the highest percentage of internationally collaborative papers. For most subject areas, China’s international-article percentage was lower than every other leading country in the Nature Index in 2023*. In biological sciences, however, it is 54.1%, a higher proportion than the United States (52.7%).
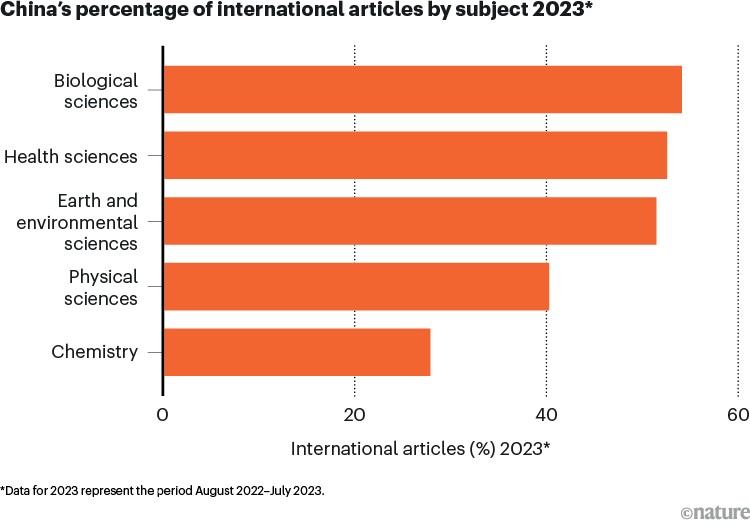
Strength in numbers
China might be more outward-looking in its approach to biological sciences research, but it still dominates its top three international partnerships in the subject. A different dynamic can be seen in its collaboration with Harvard University in Cambridge, Massachusetts, which has more than double the collaboration score (6.39) of the Chinese Academy of Sciences in Beijing (3.02), in the fourth-ranked international partnership in the subject (not shown).
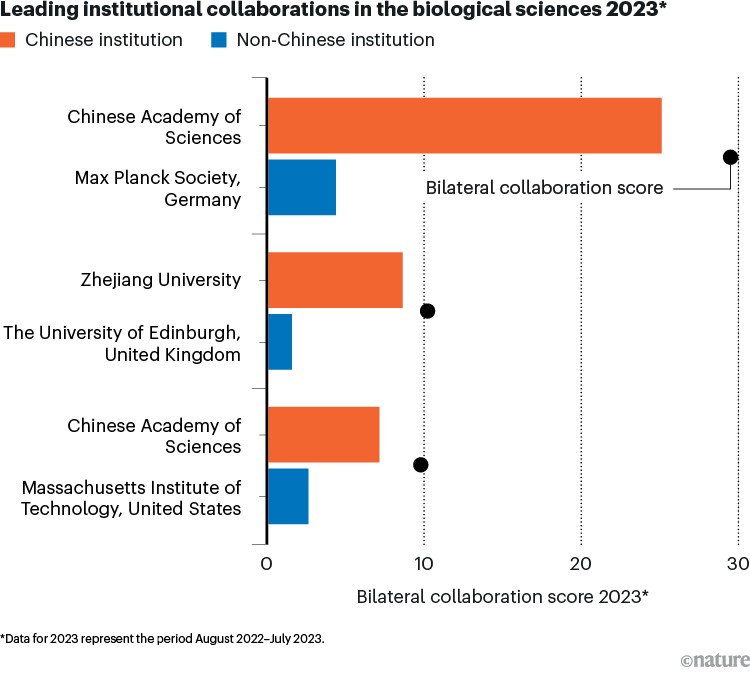
Concentrated expertise
It’s perhaps no surprise that China’s largest research institute, the Chinese Academy of Sciences, forms five of the country’s ten leading international partnerships in biological sciences. What is striking is the strength of the University of Hong Kong — a much smaller institution — which forms the top three international health-sciences collaborations. Among China’s top international collaborations in health sciences and biology, the University of Sydney is the only institution from outside Europe and the United States.
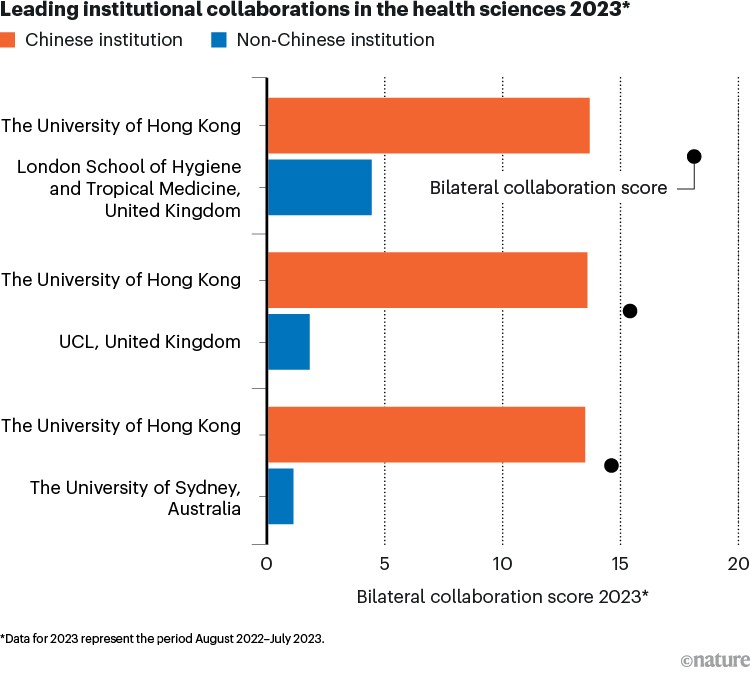
Nature 630 , S17 (2024)
doi: https://doi.org/10.1038/d41586-024-01600-9
This article is part of Nature Index 2024 China , an editorially independent supplement. Advertisers have no influence over the content. For more information about Nature Index, see the homepage .
Related Articles
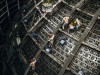
Partner content: How innovative research is improving dairy products
Partner content: Fighting micronutrient deficiencies in China
Partner content: From ancient remedies to skincare innovation
Partner content: China’s largest university courts global scholars
- Research data
- Institutions
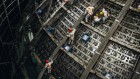
China seeks global impact and recognition
Nature Index 05 JUN 24
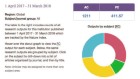
A guide to the Nature Index
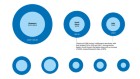
Chinese research collaborations shift to the Belt and Road
Unlocking carbene reactivity by metallaphotoredox α-elimination
Article 06 JUN 24
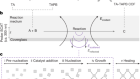
Early stages of covalent organic framework formation imaged in operando
Article 05 JUN 24
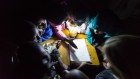
More than a billion people live in ‘energy poverty’
Research Highlight 30 MAY 24
Faculty Positions in School of Engineering, Westlake University
The School of Engineering (SOE) at Westlake University is seeking to fill multiple tenured or tenure-track faculty positions in all ranks.
Hangzhou, Zhejiang, China
Westlake University
High-Level Talents at the First Affiliated Hospital of Nanchang University
For clinical medicine and basic medicine; basic research of emerging inter-disciplines and medical big data.
Nanchang, Jiangxi, China
The First Affiliated Hospital of Nanchang University
Professor/Associate Professor/Assistant Professor/Senior Lecturer/Lecturer
The School of Science and Engineering (SSE) at The Chinese University of Hong Kong, Shenzhen (CUHK-Shenzhen) sincerely invites applications for mul...
Shenzhen, China
The Chinese University of Hong Kong, Shenzhen (CUHK Shenzhen)
Faculty Positions& Postdoctoral Research Fellow, School of Optical and Electronic Information, HUST
Job Opportunities: Leading talents, young talents, overseas outstanding young scholars, postdoctoral researchers.
Wuhan, Hubei, China
School of Optical and Electronic Information, Huazhong University of Science and Technology
Sign up for the Nature Briefing newsletter — what matters in science, free to your inbox daily.
Quick links
- Explore articles by subject
- Guide to authors
- Editorial policies
Advertisement
Teaching With and About Nature of Science, and Science Teacher Knowledge Domains
- Published: 04 August 2012
- Volume 22 , pages 2087–2107, ( 2013 )
Cite this article
- Fouad Abd-El-Khalick 1
4811 Accesses
135 Citations
1 Altmetric
Explore all metrics
The ubiquitous goals of helping precollege students develop informed conceptions of nature of science (NOS) and experience inquiry learning environments that progressively approximate authentic scientific practice have been long-standing and central aims of science education reforms around the globe. However, the realization of these goals continues to elude the science education community partly because of a persistent, albeit not empirically supported, coupling of the two goals in the form of ‘teaching about NOS with inquiry’. In this context, the present paper aims, first, to introduce the notions of, and articulate the distinction between, teaching with and about NOS, which will allow for the meaningful coupling of the two desired goals. Second, the paper aims to explicate science teachers’ knowledge domains requisite for effective teaching with and about NOS. The paper argues that research and development efforts dedicated to helping science teachers develop deep, robust, and integrated NOS understandings would have the dual benefits of not only enabling teachers to convey to students images of science and scientific practice that are commensurate with historical, philosophical, sociological, and psychological scholarship (teaching about NOS), but also to structure robust inquiry learning environments that approximate authentic scientific practice, and implement effective pedagogical approaches that share a lot of the characteristics of best science teaching practices (teaching with NOS).
This is a preview of subscription content, log in via an institution to check access.
Access this article
Price includes VAT (Russian Federation)
Instant access to the full article PDF.
Rent this article via DeepDyve
Institutional subscriptions
Similar content being viewed by others
Social Learning Theory—Albert Bandura
Discovery learning—jerome bruner, social constructivism—jerome bruner.
Abd-El-Khalick, F. (2003). Socioscientific issues in pre-college science classrooms: The primacy of learners’ epistemological orientations and views of nature of science. In D. L. Zeidler (Ed.), The role of moral reasoning in socioscientific issues and discourse in science education (pp. 41–61). Dordrecht, The Netherlands: Kluwer.
Chapter Google Scholar
Abd-El-Khalick, F. (2005). Developing deeper understandings of nature of science: The impact of a philosophy of science course on preservice science teachers’ views and instructional planning. International Journal of Science Education, 27 (1), 15–42.
Article Google Scholar
Abd-El-Khalick, F. (2008). Modeling science classrooms after scientific laboratories. In R. A. Duschl & R. E. Grandy (Eds.), Teaching scientific inquiry: Recommendations for research and application (pp. 80–85). Rotterdam, The Netherlands: Sense.
Google Scholar
Abd-El-Khalick, F. (2012). Nature of science in science education: Toward a coherent framework for synergistic research and development. In B. J. Fraser, K. Tobin, & C. McRobbie (Eds.), Second international handbook of science education (Vol. 2, pp. 1041–1060). The Netherlands: Springer.
Abd-El-Khalick, F., & Akerson, V. L. (2004). Learning about nature of science as conceptual change: Factors that mediate the development of preservice elementary teachers’ views of nature of science. Science Education, 88 (5), 785–810.
Abd-El-Khalick, F., & Akerson, V. L. (2009). The influence of metacognitive training on preservice elementary teachers’ conceptions of nature of science. International Journal of Science Education, 31 (16), 2161–2184.
Abd-El-Khalick, F., Bell, R. L., & Lederman, N. G. (1998). The nature of science and instructional practice: Making the unnatural natural. Science Education, 82 , 417–437.
Abd-El-Khalick, F., BouJaoude, S., Duschl, R. A., Hofstein, A., Lederman, N. G., Mamlok, R., et al. (2004). Inquiry in science education: International perspectives. Science Education, 88 (3), 397–419.
Abd-El-Khalick, F., & Lederman, N. G. (2000a). Improving science teachers’ conceptions of nature of science: A critical review of the literature. International Journal of Science Education, 22 (7), 665–701.
Abd-El-Khalick, F., & Lederman, N. G. (2000b). The influence of history of science courses on students’ views of nature of science. Journal of Research in Science Teaching, 37 (10), 1057–1095.
Abd-El-Khalick, F., Waters, M., & Le, A. (2008). Representations of nature of science in high school chemistry textbooks over the past four decades. Journal of Research in Science Teaching, 45 (7), 835–855.
Aguirre, J. M., Haggerty, S. M., & Linder, C. J. (1990). Student-teachers’ conceptions of science, teaching and learning: A case study in preservice science education. International Journal of Science Education, 12 , 381–390.
Akerson, V. L., Buzzelli, C. A., & Donnelly, L. A. (2010). On the nature of teaching nature of science: Preservice early childhood teachers’ instruction in preschool and elementary settings. Journal of Research in Science Teaching, 47 (2), 213–233.
Akindehin, F. (1988). Effect of an instructional package on preservice science teachers’ understanding of the nature of science and acquisition of science-related attitudes. Science Education, 72 (1), 73–82.
Allchin, D. (2011). Teaching whole science. American Biology Teacher, 73 , 53–55.
American Association for the Advancement of Science. (1990). Science for all Americans . New York: Oxford University Press.
Anderson, R. (2007). Inquiry as an organizing theme for science curricula. In S. Abell & N. Lederman (Eds.), Handbook of research on science education (pp. 807–830). Mahwah, NJ: Lawrence Erlbaum Associates.
Barlow, N. (Ed.). (1993/1958). The autobiography of Charles Darwin: 1809 – 1882 . New York: W. W. Norton.
Barufaldi, J. P., Bethel, L. J., & Lamb, W. G. (1977). The effect of a science methods course on the philosophical view of science among elementary education majors. Journal of Research in Science Teaching, 14 (4), 289–294.
Bell, R. L., Blair, L. M., Crawford, B. A., & Lederman, N. G. (2003). Just do it? Impact of a science apprenticeship program on high school students’ understandings of the nature of science and scientific inquiry. Journal of Research in Science Teaching, 40 (5), 487–509.
BouJaoude, S., Salloum, S., & Abd-El-Khalick, F. (2004). Relationships between selective cognitive variables and students’ ability to solve chemistry problems. International Journal of Science Education, 26 (1), 63–84.
Brewer, W. F., & Chinn, C. A. (1994). The theory-ladenness of data: An experimental demonstration. In A. Ram & K. Eiselt (Eds.), Proceedings of the sixteenth annual conference of the cognitive science society (pp. 61–65). Hillsdale, NJ: Lawrence Erlbaum.
Brickhouse, N. W. (1989). The teaching of the philosophy of science in secondary classrooms: Case studies of teachers’ personal theories. International Journal of Science Education, 11 , 401–415.
Burbules, N., & Linn, M. C. (1991). Science education and philosophy of science: Congruence or contradiction? International Journal of Science Education, 13 (3), 227–242.
Chinn, C. A., & Brewer, W. F. (1993). The role of anomalous data in knowledge acquisition: A theoretical framework and implications for science instruction. Review of Educational Research, 63 , 1–49.
Chinn, C. A., & Malhorta, B. A. (2002). Children’s responses to anomalous scientific data: How is conceptual change impeded? Journal of Educational Psychology, 94 (2), 327–343.
Chinn, C. A., & Malhotra, B. A. (2002). Epistemologically authentic inquiry in schools: A theoretical framework for evaluating inquiry tasks. Science Education, 86 , 175–218.
Conant, J. B. (1957). Harvard case histories in experimental science (Vol. 1). Cambridge, MA: Harvard University Press.
Council of Ministers of Education, Canada (CMEC) Pan-Canadian Science Project. (1997). Common framework of science learning outcomes K to 12 [On-line]. Available: http://www.cmec.ca/science/framework/Pages/english/CMEC%20 Eng.html.
Crawford, B. A. (2007). Learning to teach science as inquiry in the rough and tumble of practice. Journal of Research in Science Teaching, 44 (4), 613–642.
Curriculum Council. (1998). Curriculum framework for Kindergarten to Year 12 education in Western Australia . Osborne Park, WA: Author.
Dogan, N., & Abd-El-Khalick, F. (2008). Turkish grade 10 students’ and science teachers’ conceptions of nature of science: A national study. Journal of Research in Science Teaching, 45 (10), 1083–1112.
Donnelly, L. A., & Sadler, T. (2009). High school science teachers’ views of standards and accountability. Science Education, 93 (6), 1050–1075.
Duhem, P. (1954). The aim and structure of physical theory (with a new introduction by Jules Vuillemin) . Princeton, NJ: Princeton University Press.
Duit, R., Treagust, D., & Widodo, A. (2008). Teaching science for conceptual change: Theory and practice. In S. Vosiniadou (Ed.), International handbook of research on conceptual change (pp. 629–646). Mahwah, NJ: Erlbaum.
Duncan, R. G., Freidenreich, H. B., Chinn, C. A., & Bausch, A. (2011). Promoting middle school students’ understandings of molecular genetics. Research in Science Education, 41 (2), 147–167.
Gillies, D. (1998). Philosophy of science in the twentieth century: Four central themes . Cambridge, MA: Blackwell.
Hewson, P. W., Beeth, M. E., & Thorley, N. R. (1998). Teaching for conceptual change. In B. J. Fraser & K. G. Tobin (Eds.), International handbook of science education (pp. 199–218). Dordrecht, The Netherlands: Kluwer.
Hillis, S. R. (1975). The development of an instrument to determine student views of the tentativeness of science. In E. J. Montague (Ed.), Research and curriculum development in science education: Science teacher behavior and student affective and cognitive learning (Vol. 3, pp. 32–38). Austin, TX: University of Texas Press.
Holton, G., Rutherford, J., & Watson, F. G. (1971). About the Project Physics Course: An introduction to the teacher resource book . New York: Holt, Rinehart, and Winston.
Holton, G., Watson, F. G., & Rutherford, F. J. (1967). Harvard project physics: A progress report. The Physics Teacher, 5 (5).
Howe, E. M. (2007). Addressing nature-of-science core tenets with the history of science: An example with sickle-cell anemia & malaria. American Biology Teacher, 69 (8), 467–472.
Howe, E. M., & Rudge, D. W. (2005). Recapitulating the history of sickle-cell anemia research: Improving students’ NOS views explicitly and reflectively. Science & Education, 14 (3–5), 423–441.
Judson, E. (2010). Science education as a contributor to adequate yearly progress and accountability. Science Education, 94 (5), 888–902.
Kang, S., Scharmann, L. C., & Noh, T. (2005). Examining students’ views on the nature of science: Results from Korean 6th, 8th, and 10th graders. Science Education, 89 (2), 314–334.
Kelly, G. J., & Duschl, R. A. (2002, April). Toward a research agenda for epistemological studies in science education. In Paper presented at the annual meeting of the National Association for Research in Science Teaching , New Orleans, LA.
Khishfe, R., & Abd-El-Khalick, F. (2002). Influence of explicit reflective versus implicit inquiry-oriented instruction on sixth graders’ views of nature of science. Journal of Research in Science Teaching, 39 (7), 551–578.
Kim, S. Y., & Irving, K. E. (2010). History of science as an instructional context: Student learning in genetics and nature of science. Science & Education, 19 (2), 187–215.
Klopfer, L. E. (1964a). History of science cases: The cells of life (teacher’s guide) . Chicago, IL: Science Research Associates.
Klopfer, L. E. (1964b). History of science cases: The cells of life . Chicago, IL: Science Research Associates.
Klopfer, L. E. (1966). History of science cases: Frogs and batteries (teacher’s guide) . Chicago, IL: Science Research Associates.
Klopfer, L. E. (1969). The teaching of science and the history of science. Journal of Research in Science Teaching, 6 (1), 87–95.
Klopfer, L. E., & Cooley, W. W. (1963). The history of science cases for high schools in the development of student understanding of science and scientists: A report on the HOSC instruction project. Journal of Research in Science Teaching, 1 (1), 33–47.
Kuhn, T. S. (1996). The structure of scientific revolutions (3rd ed.). Chicago: The University of Chicago Press. (First published 1962).
Book Google Scholar
Lederman, N. G. (1999). Teachers’ understanding of the nature of science and classroom practice: Factors that facilitate or impede the relationship. Journal of Research in Science Teaching, 36 , 916–929.
Lederman, N. G., & Abd-El-Khalick, F. (1998). Avoiding de-natured science: Activities that promote understandings of the nature of science. In W. McComas (Ed.), The nature of science in science education: Rationales and strategies (pp. 83–126). Dordrecht, The Netherlands: Kluwer.
Lederman, N. G., Schwartz, R., Abd-El-Khalick, F., & Bell, R. L. (2001). Preservice teachers’ understanding and teaching of nature of science: An intervention study. Canadian Journal of Science, Mathematics and Technology Education, 1 (2), 135–160.
Lee, O., Buxton, C., Lewis, S., & LeRoy, K. (2006). Science inquiry and student diversity: Enhanced abilities and continuing difficulties after an instructional intervention. Journal of Research in Science Teaching, 43 (7), 607–636.
Lin, H., & Chen, C. C. (2002). Promoting preservice chemistry teacher’ understandings about the nature of science through history. Journal of Research in Science Teaching, 39 (9), 773–792.
Longino, H. (1990). Science as social knowledge: Values and objectivity in scientific inquiry . Princeton, NJ: Princeton University Press.
Lunetta, V. N., Hofstein, A., & Clough, M. P. (2007). Learning and teaching school science laboratory: An analysis of research, theory, and practice. In S. K. Abell & N. G. Lederman (Eds.), Handbook of research on science education (pp. 393–441). Mahwah, NJ: Lawrence Erlbaum.
Marchlewicz, S. C., & Wink, D. L. (2011). Using the activity model of inquiry to enhance general chemistry students’ understanding of nature of science. Journal of Chemical Education, 88 (8), 1041–1047.
Mathews, M. R. (1994). Science teaching: The role of history and philosophy of science . New York: Routledge.
McDonald, C. V. (2010). The influence of explicit nature of science and argumentation instruction on preservice primary teachers’ views of nature of science. Journal of Research in Science Teaching, 47 (9), 1137–1164.
Millar, R., & Osborne, J. (Eds.). (1998). Beyond 2000: Science education for the future . London: King’s College.
Ministry of Education. (1990). Programa de articulación. Caracas. Programa de Articulación. Venezuela: Author.
Ministry of Education. (1999). Curriculum outline for “nature science and living technology.” Taipei: Ministry of Education (In Taiwanese).
Ministry of National Education. (2000). Journal of Announcements of Ministry of National Education. Ankara, Turkey: Author [In Turkish].
Monk, M., & Osborne, J. (1997). Placing the history and philosophy of science on the curriculum: A model for the development of pedagogy. Science Education, 81 (4), 405–424.
Morrison, J. A., Raab, F., & Ingram, D. (2009). Factors influencing elementary and secondary teachers’ views on the nature of science. Journal of Research in Science Teaching, 46 (4), 384–403.
National Research Council. (1996). National science education standards . Washington, DC: National Academic Press.
National Science Teachers Association. (1982). Science-Technology-Society: Science education for the 1980 s . Washington, DC: Author.
Newton-Smith, W. H. (2000). Underdetermination of theory by data. In W. H. Newton-Smith (Ed.), A companion to the philosophy of science (pp. 532–536). Malden, MA: Blackwell.
Olitsky, S. (2005). Social and cultural capital in science teaching: Relating practice and reflection. In K. Tobin, R. Elmesky, & G. Seiler (Eds.), Improving urban science education: New roles for teachers, students and researchers (pp. 279–297). New York: Rowman & Littlefield.
Peters, E., & Kitsantas, A. (2010). The effect of nature of science metacognitive prompts on science students’ content and nature of science knowledge, metacognition, and self-regulatory efficacy. School Science and Mathematics, 110 (8), 382–396.
Popper, K. R. (1963). Conjectures and refutations: The growth of scientific knowledge . London, UK: Routledge & Kagan Paul.
Quine, W. V. (1951). Two dogmas of empiricism. Philosophical Review, 60 , 20–43.
Riley, J. P., I. I. (1979). The influence of hands-on science process training on preservice teachers’ acquisition of process skills and attitude toward science and science teaching. Journal of Research in Science Teaching, 16 , 373–384.
Robinson, J. T. (1965). Science teaching and the nature of science. Journal of Research in Science Teaching, 3 , 37–50.
Rubba, P. A., & Anderson, H. (1978). Development of an instrument to assess secondary school students’ understanding of the nature of scientific knowledge. Science Education, 62 (4), 449–458.
Russell, C. B., & Weaver, G. C. (2011). A comparative study of traditional, inquiry-based, and research-based laboratory curricula: Impacts on understanding of the nature of science. Chemistry Education Research and Practice, 12 (1), 57–67.
Rutherford, F. J. (1964). The role of inquiry in science teaching. Journal of Research in Science Teaching, 2 , 80–84.
Salloum, S., & Abd-El-Khalick, F. (2010). A study of practical-moral knowledge in science teaching: Case studies in physical science classrooms. Journal of Research in Science Teaching, 47 (8), 929–951.
Sandoval, W. A., & Morrison, K. (2003). High school students’ ideas about theories and theory change after a biological inquiry unit. Journal of Research in Science Teaching, 40 (4), 369–392.
Schmuckler, J. S. (2004). Using the history of science to strengthen an understanding of the nature of science. Abstracts of papers of the American Chemical Society, 228 (1), 312.
Schwartz, R. S., & Lederman, N. G. (2002). “It’s the nature of the beast”: The influence of knowledge and intentions on learning and teaching nature of science. Journal of Research in Science Teaching, 39 (3), 205–236.
Scott, P. H., Asoko, H. M., & Driver, R. H. (1992). Teaching for conceptual change: A review of strategies. In R. Duit, F. Goldberg, & H. Niedderer (Eds.), Research in physics learning: Theoretical issues and empirical studies (pp. 310–329). Kiel, Germany: Institute for Science Education at the University of Kiel.
Scott, P., Asoko, H., & Leach, J. (2007). Student conceptions and conceptual learning in science. In S. Abell & N. Lederman (Eds.), Handbook of research on science education (pp. 31–56). Mahwah, NJ: Lawrence Erlbaum Associates.
Shanahan, M.-C., & Nieswandt, M. (2011). Science student role: Evidence of social structural norms specific to school science. Journal of Research in Science Teaching, 48 (4), 367–395.
Shulman, L. S. (1986). Those who understand: Knowledge growth in teaching. Educational Researcher, 15 , 4–14.
Shulman, L. S. (1987). Knowledge and teaching: Foundations of the new reform. Harvard Educational Review, 57 , 1–22.
So, W. M. W., & Ching, N. Y. F. (2011). Creating a collaborative science learning environment for science inquiry at the primary level. Asia-Pacific Education Researcher, 20 (3), 559–569.
Sweitzer, G. L., & Anderson, R. D. (1983). A meta-analysis of research on science teacher education practices associated with inquiry strategy. Journal of Research in Science Teaching, 20 (5), 453–466.
Treagust, D. (2007). General instructional methods and strategies. In S. K. Abell & N. G. Lederman (Eds.), Handbook of research on science education (pp. 373–392). Mahwah, NJ: Lawrence Erlbaum Associates.
van Garderen, D., Hanuscin, D., Lee, E., & Kohn, P. (2012). A collaborative professional development model to meet the needs of diverse learners in K-6 science. Psychology in the Schools, 49 (5), 429–443.
Vesilind, E. M., & Jones, M. G. (1998). Gardens or graveyards: Science education reform and school culture. Journal of Research in Science Teaching, 35 (7), 757–775.
Wahbeh, N. (2009). The effect of a content - embedded explicit - reflective approach on inservice teachers’ views and practices related to nature of science. Unpublished doctoral dissertation, University of Illinois at Urbana-Champaign, Champaign, IL.
Wahbeh, N., & Abd-El-Khalick, F. (2011, September). The primacy of content - embedded teacher understandings of nature of science in impacting instruction. In Paper presented at the biannual conference of the European Science Education Research Association, Lyon, France.
Welch, W. W. (1973). Review of the research and evaluation program of the Harvard project physics. Journal of Research in Science Teaching, 10 (4), 365–378.
Yacoubian, H. A., & BouJaoude, S. (2010). The effect of reflective discussions following inquiry-based laboratory activities on students’ views of nature of science. Journal of Research in Science Teaching, 47 (10), 1229–1252.
Download references
Author information
Authors and affiliations.
Department of Curriculum and Instruction, College of Education, University of Illinois at Urbana-Champaign, 1310 South Sixth Street, Champaign, IL, 61820, USA
Fouad Abd-El-Khalick
You can also search for this author in PubMed Google Scholar
Corresponding author
Correspondence to Fouad Abd-El-Khalick .
Rights and permissions
Reprints and permissions
About this article
Abd-El-Khalick, F. Teaching With and About Nature of Science, and Science Teacher Knowledge Domains. Sci & Educ 22 , 2087–2107 (2013). https://doi.org/10.1007/s11191-012-9520-2
Download citation
Published : 04 August 2012
Issue Date : September 2013
DOI : https://doi.org/10.1007/s11191-012-9520-2
Share this article
Anyone you share the following link with will be able to read this content:
Sorry, a shareable link is not currently available for this article.
Provided by the Springer Nature SharedIt content-sharing initiative
- Science Teacher
- Knowledge Domain
- Pedagogical Content Knowledge
- Science Content
- Science Content Knowledge
- Find a journal
- Publish with us
- Track your research
CS50: Introduction to Computer Science
An introduction to the intellectual enterprises of computer science and the art of programming.
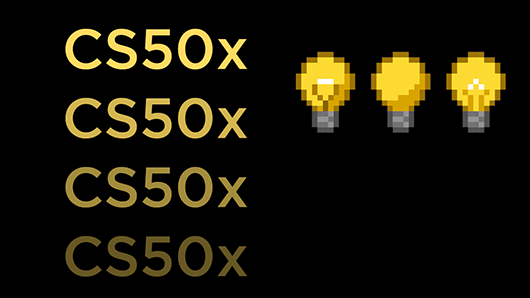
Associated Schools
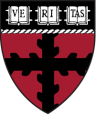
Harvard School of Engineering and Applied Sciences
What you'll learn.
A broad and robust understanding of computer science and programming
How to think algorithmically and solve programming problems efficiently
Concepts like abstraction, algorithms, data structures, encapsulation, resource management, security, software engineering, and web development
Familiarity with a number of languages, including C, Python, SQL, and JavaScript plus CSS and HTML
How to engage with a vibrant community of like-minded learners from all levels of experience
How to develop and present a final programming project to your peers
Course description
This is CS50x , Harvard University's introduction to the intellectual enterprises of computer science and the art of programming for majors and non-majors alike, with or without prior programming experience. An entry-level course taught by David J. Malan, CS50x teaches students how to think algorithmically and solve problems efficiently. Topics include abstraction, algorithms, data structures, encapsulation, resource management, security, software engineering, and web development. Languages include C, Python, SQL, and JavaScript plus CSS and HTML. Problem sets inspired by real-world domains of biology, cryptography, finance, forensics, and gaming. The on-campus version of CS50x , CS50, is Harvard's largest course.
Students who earn a satisfactory score on 9 problem sets (i.e., programming assignments) and a final project are eligible for a certificate. This is a self-paced course–you may take CS50x on your own schedule.
Instructors
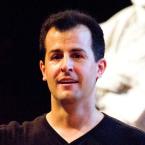
David J. Malan
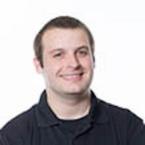
You may also like
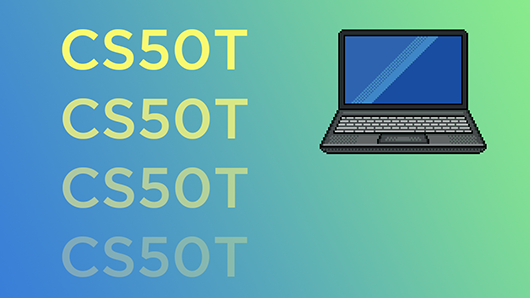
CS50's Understanding Technology
This is CS50’s introduction to technology for students who don’t (yet!) consider themselves computer persons.
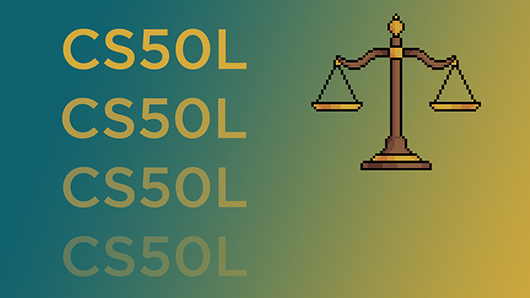
CS50 for Lawyers
This course is a variant of Harvard University's introduction to computer science, CS50, designed especially for lawyers (and law students).
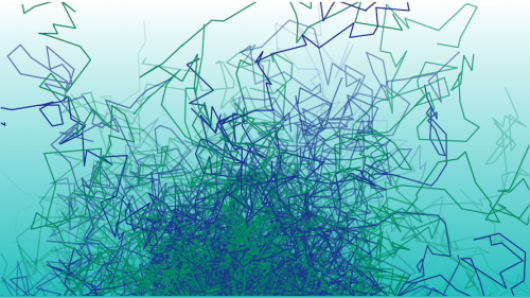
Using Python for Research
Take your introductory knowledge of Python programming to the next level and learn how to use Python 3 for your research.
Join our list to learn more

IMAGES
VIDEO
COMMENTS
The purpose of this chapter is to update new advances in the research on the teaching and learning of nature of science since the previous Handbook of Research on Science Education (Abell & Lederman, 2007). As this is a new volume, the previous handbook will remain in print, and so a complete recapitulation of what was published previously is not necessary. However, some review of research ...
Our goal in this article is to provide research-based strategies for embedding Nature of Science (NOS) into science instruction at the elementary level. We thus intend to aid researchers, professional developers, and teachers in noting that not only is it important and possible to teach NOS at the elementary levels, but also that elementary students can learn ideas about NOS. The manuscript ...
This review traces the history of research on the teaching and learning of nature of scientific knowledge (NOSK), and its implications for curriculum and instruction. Initially, the complex rubric of NOSK is clearly conceptualized, while recognizing that there is no singularly accepted definition. As part of this conceptualization NOSK is distinguished from the body of scientific knowledge and ...
The Teaching and Learning about the Nature of Science special virtual issue illustrates the value of work appearing in Science & Education, and it seeks to illuminate a way forward that informs research and practice so that in the not-too-distant future, such a special issue will make clear the significant positive impact teaching and learning ...
The development of adequate student conceptions of the nature of science has been a perennial objective of science instruction regardless of the currently advocated pedagogical or curricular emphases. Consequently, it has been an area of prolific research characterized by several parallel, but distinct, lines of investigation.
This book offers a comprehensive introduction to Nature of Science (NOS), one of the most important aspects of science teaching and learning, and includes tested strategies for teaching aspects of the NOS in a variety of instructional settings. In line with the recommendations in the field to include NOS in all plans for science instruction ...
ABSTRACT. Volumes have been written arguing why NOS is an important educational objective. Simply put, understanding NOS is often defended as being a critical component of scientifi c literacy (Lederman & Lederman, 2011; NGSS Lead States, 2013; NSTA, 1982). For more elaborated rationales, the reader is referred to Driver, Leach, Millar, and ...
Teaching and Learning of Nature of Scientific Knowledge and Scientific Inquiry: Building Capacity through Systematic Research-Based Professional Development Norman G. Lederman Mathematics and Science Education, Illinois Institute of Technology, Chicago, Illinois, USA Correspondence [email protected]
The second section in this book, which contains three chapters, focuses on Action Research Studies on Online Nature of Science Teaching and Learning The first chapter explored the results of ...
At first glance, teaching about the nature of science can appear esoteric and far removed from students' daily experiences. Decades of research on teaching and learning about the nature of science points to some specific approaches that can make instruction about the nature of science both more effective and engaging. Be Explicit
Nature of science (NOS) is a critical component of scientific literacy that enhances students' understandings of science concepts and enables them to make informed decisions about scientifically-based personal and societal issues. NOS is derived not only from the eight science practices delineated in the Framework for K-12 Science Education ...
Fig. 1 NOS research and teaching contexts views before and after instruction during an informal sci-ence enrichment Saturday Science program entitled, "What is Science? Exploring the True Nature of Science." The course included six sessions that each lasted 2.5 h. Although each session addressed a different science con-
Book Handbook of Research on Science Education. Edition 1st Edition. First Published 2023. Imprint Routledge. Pages 49. eBook ISBN 9780367855758. Previous Chapter Next Chapter. Research on Teaching, Learning, and Assessment of Nature of Science - 1.
Research on Teaching, Learning, and Assessment of Nature of Science. January 2023. DOI: 10.4324/9780367855758-32. In book: Handbook of Research on Science Education (pp.850-898) Authors: Fouad Abd ...
The purpose of this chapter is to update new advances in the research on the teaching and learning of nature of science since the previous Handbook of Research on Science Education (Abell & Lederman, 2007). As this is a new volume, the previous handbook will remain in print, and so a complete recapitulation of what was published previously is ...
(2002). Views of the nature of science questionnaire: toward valid and meaningful assessment of learner's conceptions of the nature of science. Journal of Research in Science Teaching, 39(6), 497-521. Article Google Scholar Lederman, NG, & Lederman, JS (2014). Research on teaching and learning of nature of science.
Learning about the Nature of Science (NOS) has been defended by numerous researchers and educators as one of the main goals of science education, particularly since the last two decades of the twentieth century (for reviews, see, e.g., McComas et al., 1998; Lederman, 2007; Hodson, 2014; Matthews, 2015).NOS can be understood as a pedagogical construct that seeks to foster the understanding of ...
teaching and learning. What should schooling, teaching and, most especially, learning look like in this rapidly changing world? At the same time, empirical research on how people learn, how the mind and brain develop, how interests form, and how people differ in all these has expanded tremendously. This science of learning
Testing ideas with evidence from the natural world is at the core of science. Scientific testing involves figuring out what we would expect to observe if an idea were correct and comparing that expectation to what we actually observe. Scientific arguments are built from an idea and the evidence relevant to that idea.
All of the above studies indicate the researcher's research on social media aids in teaching and learning. All of these studies indicate the positive impact of social media on teaching and learning.
The limitless learning potential of all students and ways to communicate this information to students; The nature of our data-filled world and the meaning of data science; The importance of data literacy for all students; Data analysis tools and different ways to analyze and visualize data; The cross-curricular nature of data literacy and learning
Science education in the Philippines is anchored on the United Nations (UN) goals on sustainability education, as provided in K to 12 Science Curriculum, which envisions learners to be environmentally literate, critical problem solvers, and responsible stewards of nature. However, K to 12 implementations in the Philippines faced several challenges that resulted in student's poor awareness ...
This material is based upon work supported by the NSF National Center for Atmospheric Research, a major facility sponsored by the U.S. National Science Foundation and managed by the University Corporation for Atmospheric Research.
The Nuclear Research Foundation School Certificate Integrated, Volume 1. Book ... Academic Libraries and Public Engagement with Science and Technology. Book • 2019. Academic Libraries and Toxic Leadership. Book ... A Conversation on New Approaches to Teaching and Learning in the post-COVID World. Book • 2022. ACC Current Journal Review. Journal
As a world leader in gifted education, advocacy, and research, CTY provides: ... An inclusive community of peers who share a passion for learning; Advanced students thrive when they accelerate their learning, challenge themselves, and follow their curiosity in a safe, supportive environment. So, whether you're looking to get ahead in your ...
Chemistry and physical sciences are clear areas of focus for China, accounting for 85% of the country's total Share in the Nature Index in 2023*. But output in other subjects is growing fast ...
The ubiquitous goals of helping precollege students develop informed conceptions of nature of science (NOS) and experience inquiry learning environments that progressively approximate authentic scientific practice have been long-standing and central aims of science education reforms around the globe. However, the realization of these goals continues to elude the science education community ...
A broad and robust understanding of computer science and programming How to think algorithmically and solve programming problems efficiently Concepts like abstraction, algorithms, data structures, encapsulation, resource management, security, software engineering, and web development
Commonly, the answer to this question has three parts. First, science is a body of knowledge. This refers to the traditional subjects or body of concepts, laws, and theories, for instance, in biology, chemistry, physics etc. The second part refers to how the knowledge is devel-oped. That is scientific inquiry (or what is referred to as ...