Work and change in industrial society: a sociological perspective
Cite this chapter.
- Andrew Cameron
57 Accesses
Despite predictions of the ‘collapse of work’ or the arrival of the ‘leisure society’, it is clear that work continues to be a central institution in modern contemporary societies such as Britain.
This is a preview of subscription content, log in via an institution to check access.

Access this chapter
Subscribe and save.
- Get 10 units per month
- Download Article/Chapter or Ebook
- 1 Unit = 1 Article or 1 Chapter
- Cancel anytime
- Available as PDF
- Read on any device
- Instant download
- Own it forever
- Available as EPUB and PDF
- Compact, lightweight edition
- Dispatched in 3 to 5 business days
- Free shipping worldwide - see info
Tax calculation will be finalised at checkout
Purchases are for personal use only
Institutional subscriptions
Unable to display preview. Download preview PDF.
Similar content being viewed by others
The Sociology of Work: From Industrial Sociology to Work, Employment and the Economy
The Sociology of Work in France
Sociology of work in germany, references and further reading.
Abbott, P. (1990) Health visitors: policing the family, in Abbott, P. and Wallace, C. The Sociology of the Caring Professions , The Falmer Press, London.
Google Scholar
Allen, S. et al. (1986) The Experience of Unemployment , Macmillan, Basingstoke.
Argyris, C. (1964) Integrating the Individual and the Organisation , Wiley, New York.
Atkinson, J. (1988) Recent changes in the internal labour market structure in the UK, in Buitelaar, W. (ed.) Technology and Work: Labour Studies in England, Germany and The Netherlands , Avebury, Aldershot.
Bell, D. (1973) The Coming of Post Industrial Society , Basic Books, New York.
Berggren, C. (1993) The Volvo Experience , Macmillan, Basingstoke.
Blane, D. (1991) Health professionals, in Scambler, G. (ed.) Sociology as Applied to Medicine , Bailliere Tindall, London.
Blauner, R. (1967) Alienation and Freedom , Chicago Press, Chicago.
Braverman, H. (1974) Labour and Monopoly Capitalism: The Degradation of Work in the Twentieth Century , Monthly Review Press, New York.
Burns, T. and Stalker, G.M. (1961) The Management of Innovation , Tavistock, London.
Clegg, S.R. (1990) Modern Organisations: Organisation Studies in the Post Modern World , Sage, London.
Crook, S., Pakulski, J. and Waters, M. (1992) Postmodernization: Change in Advanced Society , Sage, London.
Dahrendorf, R. (1959) Class and Class Conflict in Industrial Society , Routledge, Kegan & Paul, London.
Doyal, L. (1979) The Political Economy of Health , Pluto, London.
Durkheim, E. (1984) The Division of Labour in Society , Macmillan, Basingstoke.
Eldridge, J.E.T. (1971) Sociology and Industrial Life , Nelson, London.
Elston, M. (1991) The politics of professional power: medicine in a changing health service, in Gabe, J., Calnan, M. and Bury, M. The Sociology of the Health Service , Routledge, London.
Evans, S., Ewing, K. and Nolan, P. (1992) Industrial relations and the British Economy in the 1990s; Mrs Thatcher’s Legacy, Journal of Management Studies , 29 , Sept. 1992, 571–89.
Article Google Scholar
Fielding, A. (1988) Professional elites and the state in privatising Britain, in Thakuar, R.N. (ed.) Elites: Paradigm and Change in Transnational Perspective , Indian Political Institute.
Fox, N.J. (1993) Postmodernism Sociology and Health , Open University Press, Milton Keynes.
Freidson, E. (1986) Professional Powers , Chicago University Press, Chicago.
Gabe, J., Calnan, N. and Bury, M. (1991) The Sociology of the Health Service , Routledge, London.
Book Google Scholar
Gabriel, Y. (1988) Working Lives in Catering , Routledge, Kegan & Paul, London.
Gallie, D., Marsh, C. and Vogler, C. (1994) Social Change and the Experience of Unemployment , Oxford University Press, Oxford.
Garrahan, P. (1992) The Nissan Enigma: Flexibility at Work in a Local Economy , Mansell, London.
General Household Survey, as Quoted in Social Trends (1991), HMSO, London.
Gershuny, J. (1983) The New Service Economy , Frances Pinter, London.
Gittens, D. (1982) Fair Sex , Hutchinson, London.
Goffman, E. (1961) Asylums: Essays on the social situation of Mental Patients and other inmates , Doubleday & Co, New York.
Goldthorpe, J., Lockwood, D., Bechhofer, F. and Platt, J. (1968) The Affluent Worker: Industrial Attitudes and Behaviour , Cambridge University Press, Cambridge.
Goldthorpe, J. et al. (1987) Social Mobility and Class Structure in Modern Britain , Oxford University Press, Oxford.
Halmos, P. (1970) The Personal Service Society , Constable, London.
Handy, C. (1984) The Future of Work: A Guide to a Changing Society , Blackwell, Oxford.
Herzberg, F., Mausner, B. and Snyderman, B. (1959) The Motivation to Work , Wiley, New York.
Illich, I. (1975) Medical Nemesis: The Expropriation of Health , Calder & Boyers, London.
Jahoda, M. (1982) Employment and Unemployment: A Social and Psychological Analysis , Cambridge University Press, Cambridge.
Jacobson, B., Smith, A. and Whitehead, M. (1991) The Nation’s Health: A Strategy for the Nineties , King Edward’s Hospital Fund, London.
Knox, S.S., Theorell, T., Svensonn, J.C. and Waller, D. (1985) The relation of social support and working environment to medical variables associated with elevated blood pressure in young males: a structural model. Social Science and Medicine , 21 , 525–31.
Article PubMed CAS Google Scholar
Kumar, K. (1978) Prophecy and Progress , Allen Lane, London.
Likert, R. (1961) New Patterns of Management , McGraw Hill, New York.
McGregor, D. (1960) The Human Side of Enterprise , McGraw Hill, New York.
Maslow, A. (1957) Motivation and Personality , Harper & Row, New York.
Marx, K. (1844) Economic and philosophical manuscripts, in McLellan, D. (1977) (ed.) Karl Marx: Selected Writings , Oxford University Press, Oxford.
Mills, A.J. and Murgatroyd, S.J. (1991) Organisational Rules , Open University Press, Milton Keynes.
Morgan, G. (1986) Images of Organisation , Sage, London.
Morgan, M. (1991) The doctor-patient relationship, in Scambler, G. (ed.) Sociology as Applied to Medicine , Bailliere Tindall, London.
Pilgrim, D. and Rogers, A. (1993) A Sociology of Mental Health and Illness , Open University Press, Milton Keynes.
Portwood, D. (1985) The quiescence of the unemployed: a sociological perspective, Journal of Community Studies .
Reed, M.I. (1992) The Sociology of Organisations: Themes, Perspectives and Prospects , Harvester, London.
Roethlisberger, F. J. and Dickson, W. J. (1939), Management and the Worker , Harvard University Press, Cambridge, MA.
Rose, M. (1975) Industrial Behaviour: Theoretical Developments since Taylor , Allen Lane, Harmondsworth.
Silverman, D. (1970) The Theory of Organisation , Heinemann, London.
Social Trends (1993) (ed.) J. Church, HMSO, London.
Storey, J. (1992) Human resource management in the public sector, in Salaman, G. (ed.) Human Resource Strategies , Sage, London.
Taylor, F.W. (1911) The Principles of Scientific Management , Harper & Row, New York.
Thompson, P. (1989) The Nature of Work , Macmillan, Basingstoke.
Watson, T.J. (1987) Sociology, Work and Industry , Routledge & Kegan Paul, London.
Weber, M. (1968) Economy and Society , Bedminster, New York.
Weber, M. (1991) The Protestant Ethic and the Spirit of Capitalism , Harper Collins Academic, London.
Whitehead, M. (1987) The Health Divide , Health Education Authority, London.
Willmott, P. and Young, M. (1973) The Symmetrical Family , Routledge & Kegan Paul, London.
Download references
You can also search for this author in PubMed Google Scholar
Editor information
Editors and affiliations.
West Midlands Regional Health Authority, UK
Margaret Bamford ( Director of Nursing Services (Acting ) ( Director of Nursing Services (Acting )
Rights and permissions
Reprints and permissions
Copyright information
© 1995 Springer Science+Business Media Dordrecht
About this chapter
Cameron, A. (1995). Work and change in industrial society: a sociological perspective. In: Bamford, M. (eds) Work and Health. Springer, Boston, MA. https://doi.org/10.1007/978-1-4899-4423-8_4
Download citation
DOI : https://doi.org/10.1007/978-1-4899-4423-8_4
Publisher Name : Springer, Boston, MA
Print ISBN : 978-0-412-48430-8
Online ISBN : 978-1-4899-4423-8
eBook Packages : Springer Book Archive
Share this chapter
Anyone you share the following link with will be able to read this content:
Sorry, a shareable link is not currently available for this article.
Provided by the Springer Nature SharedIt content-sharing initiative
- Publish with us
Policies and ethics
- Find a journal
- Track your research
- DOI: 10.1111/J.1467-6486.1979.TB00379.X
- Corpus ID: 145589317
INDUSTRIAL SOCIOLOGY: THEORY, RESEARCH AND TEACHING–SOME PROBLEMS AND PROPOSALS
- Published 1 May 1979
- Journal of Management Studies
3 Citations
Work and the sociological imagination, the teaching of industrial and other sociologies in higher education: the case of hotel and catering management studies, against social science, 8 references, towards a sociology of organisational structure, a critical evaluation of fox's radicalisation of industrial relations theory, approaches to the study of white-collar unionism, economic rationality and the relevance of weberian sociology to industrial relations, the two sociologies, the effort bargain, theoretical requirements of the applied social sciences, type of work and motivation, related papers.
Showing 1 through 3 of 0 Related Papers
Industrial Sociology: Overview
Industrial Sociology is a relatively young branch of sociology. While Durkheim and Max Weber made some analysis of industrial institutions, industrial sociology as a separate branch got its push due to the famous experiments at Hawthorne Works in Chicago, of the Western Electric Company, conducted by George Elton Mayo and his associates between 1924 and 1932. While the field cannot be confined by any one definition, various sociologist have tried to define industrial sociology in the following ways-
According to Miller and Form , “Industrial Sociology is a substantive area of general sociology which might more accurately be termed the sociology of work organisations or sociology of economy”.
According to J H Smith , “Industrial Sociology is concerned with industry (or any form of work organisation) as a social system, including those factors (technical, emotional, political) which affect the structure, the function and the changes in that system”.
However, there is no general agreement among sociologists about the origin, definition and content of the sub-discipline.
CONCEPT OF INDUSTRIAL SOCIOLOGY
Sociology is the study of the collective behaviour of people together in society as a whole. It would explain the reasons for why people behave the way they tend to behave. And when our economies turned into industrial economy urbanization began with the competition. Industrialization is both boon and bane for our society. Industrialization brought a lot of development alongside class divisions among the people. And so has the social problems that have arisen with all the complexities.
Industrial sociology does not merely see them as an economic organization but more like a social or human organization. The concept covers how people communicate with each other, who do they communicate at the times of distress, how the community
Emergence and Evolution
With the rise of industrialisation rose interest in socio-economic aspects of industrial societies. Industrial sociology has blurred contour and as its subject matter has not been delimited, it often crosses over into the subject matter of Economics. Adam Smith addressed the philosophical issues and moral sentiments attached to economic activities. Later he turned his attention to the social (macroscopic) and organisational (microscopic) roles in society of capitalist and labours. He regarded effective mobilisation and utilisation of labour as the true wealth of a nation instead of material things such as silver and gold. Smith’s work on free trade, minimal government interference, the role of differentiated divisions of labour, the rise of ‘factory system’ laid out the basics for modern industrial sociological research: economic organisation, manager’s and worker’s waves, economic exchange and trade, the correlation between technology and division of labour, the distributive effect of industrialisation on national stratification system, etc.
Subject Matter
Burns has classified the subject matter of industrial sociology in the following ways-
Industrial Human/Social Relations
Industries form human interactions and relations across fields. These social relations can be categorised in the following ways-
Scope of Industrial Sociology
Industrial sociology is an applied discipline. The following things come under its scope. This list is not exhaustive.
Importance of Industrial Sociology
Why do we require industrial sociology.
The concept of industrial sociology is wide and is very vital to the organization. The following could be stated a few of the reasons why we would require industrial sociology.
With the event of industrialization and vast developing economy, it is very important for us to realize the human potential in order to compete in the vast competition. In order to realize human potential, it is important for us to learn human behaviours in the first place in a given situation. Industrial sociology serves as a platform to learn about the human pattern of behaviour.
https://www.researchgate.net/publication/313973752_
Sociology Group
We believe in sharing knowledge with everyone and making a positive change in society through our work and contributions. If you are interested in joining us, please check our 'About' page for more information
We’re fighting to restore access to 500,000+ books in court this week. Join us!
Internet Archive Audio
- This Just In
- Grateful Dead
- Old Time Radio
- 78 RPMs and Cylinder Recordings
- Audio Books & Poetry
- Computers, Technology and Science
- Music, Arts & Culture
- News & Public Affairs
- Spirituality & Religion
- Radio News Archive
- Flickr Commons
- Occupy Wall Street Flickr
- NASA Images
- Solar System Collection
- Ames Research Center
- All Software
- Old School Emulation
- MS-DOS Games
- Historical Software
- Classic PC Games
- Software Library
- Kodi Archive and Support File
- Vintage Software
- CD-ROM Software
- CD-ROM Software Library
- Software Sites
- Tucows Software Library
- Shareware CD-ROMs
- Software Capsules Compilation
- CD-ROM Images
- ZX Spectrum
- DOOM Level CD

- Smithsonian Libraries
- FEDLINK (US)
- Lincoln Collection
- American Libraries
- Canadian Libraries
- Universal Library
- Project Gutenberg
- Children's Library
- Biodiversity Heritage Library
- Books by Language
- Additional Collections
- Prelinger Archives
- Democracy Now!
- Occupy Wall Street
- TV NSA Clip Library
- Animation & Cartoons
- Arts & Music
- Computers & Technology
- Cultural & Academic Films
- Ephemeral Films
- Sports Videos
- Videogame Videos
- Youth Media
Search the history of over 866 billion web pages on the Internet.
Mobile Apps
- Wayback Machine (iOS)
- Wayback Machine (Android)
Browser Extensions
Archive-it subscription.
- Explore the Collections
- Build Collections
Save Page Now
Capture a web page as it appears now for use as a trusted citation in the future.
Please enter a valid web address
- Donate Donate icon An illustration of a heart shape
Industrial sociology and industrial relations:
Bookreader item preview, share or embed this item, flag this item for.
- Graphic Violence
- Explicit Sexual Content
- Hate Speech
- Misinformation/Disinformation
- Marketing/Phishing/Advertising
- Misleading/Inaccurate/Missing Metadata
![[WorldCat (this item)] [WorldCat (this item)]](https://archive.org/images/worldcat-small.png)
plus-circle Add Review comment Reviews
378 Previews
5 Favorites
DOWNLOAD OPTIONS
No suitable files to display here.
EPUB and PDF access not available for this item.
IN COLLECTIONS
Uploaded by station04.cebu on July 30, 2019
Industrial Sociology Research Paper

View sample sociology research paper on industrial sociology. Browse other research paper examples for more inspiration. If you need a thorough research paper written according to all the academic standards, you can always turn to our experienced writers for help. This is how your paper can get an A! Feel free to contact our custom writing service for professional assistance. We offer high-quality assignments for reasonable rates.
Trying to define and then describe industrial sociology is a challenge because there is no general agreement among sociologists about the definition of industrial sociology or even the content of the subdiscipline (Miller 1984). This disagreement has produced alternative labels for the subdiscipline from “sociology of work” to “work and occupations” to “organizational sociology.” Furthermore, there is no sense of identity among social scientists conducting industrial sociology investigations. While important industrial sociological research is being conducted, it is spread among many different disciplines, including sociology, economics, and business. Here, industrial sociology will be defined as the study of work and work organizations, careers and adjustments by workers, and the relations of workers and work organizations to the community and society (Miller 1984; Stover, Lichty, and Stover 1999).
Academic Writing, Editing, Proofreading, And Problem Solving Services
Get 10% off with 24start discount code, the history.
Investigations of topics that would eventually be labeled industrial sociology began in the early part of the twentieth century. In-depth studies of occupations such as prostitutes, teachers, salespeople, physicians, waitresses, and ministers were conducted in the 1920s at the University of Chicago (Taylor 1968). However, the subdiscipline of industrial sociology is generally considered to have begun with the famous Western Electric research program conducted at the Hawthorne Works in Chicago (Whyte 1968). These studies, conducted during much of the Great Depression, were designed to understand the factors involved in worker productivity (Simpson 1989). When the studies ended, the researchers claimed to have determined that the social environment—the work group of the worker and the way workers were treated by management—had a powerful effect on worker performance (Roethlisberger and Dickson 1939). Although disagreement now exists about whether their results actually support their claims (Carey 1967; Franke and Kaul 1978; Jones 1992), there is little doubt that their conclusions captured the imagination of social scientists interested in worker productivity and culminated in substantial research projects dealing with work, workers, and the workplace.
That research activity eventually became known as industrial sociology and represented, for a time, one of the most vibrant sociology subdisciplines (Miller 1984). (For examples of the research being conducted during this time, see Chinoy 1955; Walker 1950; Walker and Guest 1952; Walker, Guest, and Turner 1956.) Guest provides an example of the importance of this research when he describes the results of one of his projects. In 1948, he and his team launched a two-phase project on a community whose U.S. Steel plant was to be shut down. The first phase was to be a study of the plant and the community before the shutdown and the second was to be a study of the community after the shutdown. After the first phase was completed, the results were published in the book Steeltown. A year later, he contacted the head of public relations for U.S. Steel and asked why the mill had not yet closed. The director was surprised that Guest had not heard what had happened. Apparently, the head of engineering for U.S. Steel had read the report, realized the importance of the skill in the mill’s workforce, and convinced top management to upgrade the mill to keep it in operation. The director concluded by saying, “You won’t have a ghost town to study, but I’m sure that if you went back for a visit the Chamber of Commerce would parade you down Main Street as heroes. Everyone knows the story” (Guest 1987:8).
The Splintering
During the 1960s, industrial sociology began to splinter. As sociologists recognized the potential value of the information available from a study of the workplace, they carved out specialty areas of study. Some began to study industrial organizations instead of the workers within those organizations; others focused on nonindustrial organizations (e.g., government, education, and welfare organizations); still others focused on the characteristics of the labor force (e.g., the unequal distribution of wages among various occupations). At the same time, others chose to leave sociology and to affiliate with business schools. Miller (1984) argues that industrial sociology research began to spread outside of sociology when business schools abandoned their “trade school” image in the late 1950s and created new sociology-based courses with labels such as Business and Society, Personnel and Organizational Behavior, Management and Labor Relations, and Dynamics of the Labor Force. Through the appointment of sociologists to academic positions in business schools, sociological expertise was transferred to other disciplines (Miller 1984). This splintering is at least partially responsible for the current status of industrial sociology as a very important but underappreciated subdiscipline within sociology.
Milestone Investigations
Social scientists have investigated and described numerous exceptionally important industrial sociology topics. Among the more important are those pertaining to changes in society due to industrialization and to changes in the design and operation of industrial organizations.
Societal Changes
Convergence versus Divergence
Perhaps the most important of the topics that industrial sociologists have investigated pertain to the consequences of the industrial process. What happens to a society as it industrializes? Two opposing theories have been described. The divergence theory of industrialization suggests that although the industrialization process changes the production system of a society, the culture of a society is so strong and durable that the industrialization process has minimal, if any, effect on it. In contrast, the convergence theory of industrialization argues that the industrialization process is so strong it substantially transforms any society that is industrializing. Substantial research supports the convergence theory (Form 1976; Form and Rae 1988; Inkeles 1960; Inkeles and Rossi 1961). For a time, it appeared that Japanese workers might be exceptional and provide support for the divergence theory. However, formal investigations support the conclusion that Japan is not an exceptional case (Cole 1971; Marsh 1984; Marsh and Mannari 1976; Naoi and Schooler 1985).
Deindustrialization of America and the Development of a Service Economy
The concept deindustrialization means the loss of industrial capacity and, implicitly, the loss of goodsproducing jobs. The phrase deindustrialization of America refers to both the loss of industrial capacity and to the economic and social consequences of that loss for the United States. The development of the service economy is the counterpart to that trend. Service-producing jobs have arisen to take the place of goods-producing jobs.
The change started in the 1960s when the United States lost the virtual monopoly it had maintained on many markets since World War II; in fact, the United States was forced out of several markets (Bluestone and Harrison 1982; Harrison and Bluestone 1988). Corporations responded in several ways. As Harrison and Bluestone (1988) state, “They abandoned core businesses, invested offshore, shifted capital into overtly speculative ventures, subcontracted work to low-wage contractors here and abroad, demanded wage concessions from their employees, and substituted part-time and other forms of contingent labor for full-time workers” (p. xxvii). Bluestone and Harrison (1982) suggest that somewhere between 32 and 38 million jobs were lost during the 1970s alone as the direct result of private disinvestment in American businesses (p. 9).
The consequences of this deindustrialization are substantial. First, the ability of a country to continue to develop economically depends on having a strong and growing manufacturing base. Many industries (e.g., trucking and railroads) are highly dependent on goods production because they move parts to the assembly plants and then move the finished products to the distributors.
Second, the nature and character of the jobs available to workers change. The United States has developed a service economy. The combination of the loss of a substantial number of goods-producing jobs with the creation of a huge service sector has produced a substantial shift in the nature of the jobs available in the U.S. economy. Good jobs with good pay, good fringe benefits, job security, and guaranteed civil rights are being destroyed or moved overseas and are being replaced by bad jobs with poor pay, few fringe benefits, no job security, and little protection of civil rights.
In 1970, the proportion of workers in the goodsproducing sector of the economy was about 44 percent; by 2003, it had dropped to about 27 percent. While the number of jobs in the goods-producing sector increased slightly from about 35 million in 1970 to 37 million in 2003, the number of service-producing jobs increased from about 44 million to slightly more than 100 million ( Statistical Abstract of the United States 1997 , table 649; 2004–2005 , table 601).
The change in the characteristics of the available jobs is also clearly evident. Thurow (1987) demonstrates that in the 1963 through 1973 time frame, almost half of newly created jobs were well-paying jobs, whereas only about 20 percent were poor-paying jobs. Yet only six years later, the proportions had reversed; over 40 percent were poor-paying jobs, whereas only 10 percent were well-paying jobs (Thurow 1987). Average hourly and average weekly earnings peaked in the early 1970s; both have declined substantially since then (U.S. Bureau of Labor Statistics 2005a, table B-16; Economic Report of the President 1994:320).
The Decline of U.S. Private Sector Unionization
The 1930s and early 1940s were periods of solid growth for organized labor in the United States. The three decades following World War II were years of relative stability for unions. However, since the mid-1970s, private sector unionization has experienced a precipitous decline (Clawson and Clawson 1999). Whereas at its peak in the 1950s, almost one in three eligible workers were union members, today the number is slightly more than 12 percent and still declining (U.S. Bureau of Labor Statistics 2005b). Nationwide, total membership is down from the historic high of about 21 million in 1979 to about 16 million today (Chang and Sorrentino 1991:48; U.S. Bureau of Labor Statistics 2005b, table 1).
Two contradictory trends complicate the discussion of the decline of membership in the American labor union movement. One trend applies to private sector union membership and the second pertains to public sector union membership (essentially, governmental employment). While the unionization rate for private workers has dropped from almost 40 percent in 1960 to about 8 percent in 2005, the unionization rate for public employees shows the exact opposite trend; it has risen from about 10 percent in 1960 to close to 36 percent in 2005 (Stover, Lichty, and Stover 1999:238; U.S. Bureau of Labor Statistics 2005b:1).
The reasons given for the decline include the abandonment by management of the tacit agreement it had with unions to maintain the standard of living of current union members in exchange for the abandonment of (or at the least a diminution of) aggressive union-organizing activities, the actual attempts by management to hamper union activities among the workers in an organization, the shift by many companies of their operations to nonunion geographic areas such as the South and West, the failure of unions to successfully organize work in nonunion areas such as the South and West, the failure of unions to successfully organize traditionally nonunion work such as women-dominated occupations and service work (Kimeldorf and Stephan-Norris 1992), the choice of increasing numbers of workers to eschew unions (Farber and Krueger 1993), and changes in the legal climate making it more difficult to be protected from retaliation for union activities (Freeman and Medoff 1984; Geoghegan 1991; Weiler 1993). Structural changes in the economy— (a) the development of a service economy, (b) the shift within the manufacturing part of the economy from “traditional” to “high-tech,” and (c) the increasing importance of the export component part of the economy—have also been noted as reasons for the decline in private sector unionization (Troy 1990).
Trends in Unionization Outside the United States
But what about unions in other industrialized countries? The discussion is complicated because countries vary in their approach to organized labor. For some countries, generalizations are difficult because little is known about their policy toward organized labor. For example, the status of unions in the former Eastern Bloc nations—the former United Soviet Socialist Republics (USSR) and its East European allies—is hard to describe because those unions have been free of political control for such a short period of time that it is unclear how they will be treated. For other countries, commenting on the status of unions is a useless endeavor because organized labor has little or no legal standing. Unions in some developing countries (such as the Philippines) are either outlawed or have had their activities severely curtailed by the laws of the country (McGinnis 1979). Unionization in Japan deserves special consideration because of its variety. Many unions are company unions and are controlled to a great extent by company management (Berggren 1992; Ginsbourger 1981). Others are either industrywide unions or members of nationwide coalitions that are sometimes able to achieve worker demands (Kerbo 2006). Finally, German unions must be distinguished from unions in other industrialized countries because of their special relationship to management. Germany’s labor-management relationship is qualitatively different from that of other industrial countries because of its Mitbestimmung labor-management system— a legally mandated formal arrangement between workers and management requiring cooperation between workers and management. (For details, see the section below titled “Germany’s Mitbestimmung Labor Policy” and also Kerbo 2006:538–543.)
Accepting these caveats, several overall trends in unionization rates among various countries since World War II can be described (Chang and Sorrentino 1991; Kassalow 1984; Stover, Lichty, and Stover 1999:255). First, the proportion of the labor force unionized in most of these countries has remained remarkably stable over the last two to three decades. There have been fluctuations—some minor declines and some minor increases—but overall there is a great deal of stability. Second, France and the Netherlands, like the United States, have experienced substantial declines in unionization rates since World War II. Third, two countries—Sweden and Denmark— have experienced substantial increases. In both the countries, virtually the entire labor force is unionized.
Four differences have been noted concerning differences in the approach taken by Western European nations to organized labor and that taken by the United States (Kassalow 1984; Thurow 1992). First, there seems to be a much greater acceptance of unionization as a societal institution in European countries. While unions in the United States are the subject of considerable ambivalence, if not outright hostility, unionization in Europe is accepted as a matter of course. Second, while the United States is experiencing growth in industries that were previously heavily unionized, much of that growth is not covered by union contracts. That type of growth of nonunion employment in an industry covered by union contracts typically would not occur in Europe; the workers in a new mill or new mine would be covered automatically under the terms of a previously existing, industrywide contract.
Third, management responses to the adverse economic conditions of the 1970s and 1980s were radically different. Members of many unions in the United States had to accept severe declines in their quality of life either through pay cuts or fringe-benefit givebacks. Other unions faced attacks on their existence as companies developed tactics to convince workers to decertify their unions. Such attacks tend not to be the case in Europe. Although some union members in Europe have had to accept concessions, these concessions generally do not threaten the standard of living of the workers, and they do not represent an assault on the existence of the unions. Fourth, while workers in both high-tech and service industries—both high-growth areas in mature industrial societies—will be covered by existing contracts in Europe, they will not be covered by such contracts in the United States.
Organizational Changes
Challenges to Frederick Taylor’s Scientific Management
With his success in popularizing his scientific management theory in the late nineteenth and early twentieth centuries, Frederick Taylor (1911) saw many of his ideas about how to run organizations eventually dominate management practices (Braverman 1974; Hill 1981; Kanter 1977). As Hill (1981) notes, “Taylorism . . . established the basic philosophy of work organization which has dominated the administration of work through to the present day” (p. 27). However, there have been numerous industrial sociology investigations into the consequences of his management philosophy for workers, and calling into question the validity of his insistence that the best way to manage an industrial organization is to have managers conceptualize and plan work and to have workers carefully controlled and carefully instructed on exactly how to do the work. Berggren (1992) argues that the consequences of Taylorism—such as alienation, massive job dissatisfaction, worker absenteeism and turnover, deskilling, and worker powerlessness—were so negative there was a virtual revolt against it during the 1970s in the Western industrial world (p. 232). (For discussions about concerns with the limitations and negative consequences of Taylorism, see Blauner 1964; Braverman 1974; Chinoy 1955; Gersuny 1981; Goldman and Van Houten 1981; Gottfried 1998; Harvey 1975; Roy 1952, 1954, 1958; Walker and Guest 1952; Weil 1962.)
Sociologists have described three especially striking international challenges to the basic principles of Taylorism: (1) Germany’s Mitbestimmung labor policy, (2) Spain’s Mondragon industrial complex, and (3) sweden’s automotive assembly system.
Germany’s Mitbestimmung Labor Policy
Industrial and political leaders of West Germany planning to rebuild the economy of the country after the devastation of World War II decided not only to rebuild the physical plant of industry but also to restructure labormanagement relations as well. They embarked on a policy of Mitbestimmung (roughly translated, Mitbestimmung means codetermination) to ensure that the interests of workers would be given serious consideration in industrial organizational planning (Frege 2003; Furstenberg 1977; Kerbo 2006; Putman 1977). Workers have extensive rights and representation in all but the smallest companies through workers’ corporate board representatives and worker councils elected by employees of the company. Kerbo (2006) notes,
Workers must be given extensive information about all matters affecting them and the whole company; works councils must be consulted on any changes in policies affecting work time arrangements, overtime, work breaks, vacation times, plant wage policy systems, the introduction of new technologies and any other alterations in the work environment, as well as the hiring, transfer, reclassification, or firing of workers. (P. 540)
Furthermore, under German law, workers are assumed to have rights, legal protection, and authority equal to that of stockholders. The supervisory board of large German corporations (roughly equivalent to an American board of directors) must include representation for workers equal to that of stockholders; the supervisory board must be made up of 10 employee representatives and 10 stockholder representatives (see Diamant 1977; Rowley 1977 for critiques of Mitbestimmung ).
Spain’s Mondragon Industrial Complex
After World War II, a Catholic priest began a radical experiment in industrial development in the Spanish town of Mondragon. The radical nature of the experiment stems from the way the work organizations are owned and managed. The workers own and control the organizations. Only workers own the organization in which they are employed, and all workers own a share of the organization in which they work. Workers, acting through worker councils in each of the product or service organizations, establish the policies of the company and hire management to carry out the policies; managers are thus the subordinates of the workers. Managers do not make policy, and they have little say in the policies that are created. They must carry out policy; managers who fail to carry out worker directives can be fired (Johnson and Whyte 1977; Whyte and Whyte 1991).
The Mondragon experiment has recorded substantial organizational success. Of the 103 worker cooperatives (and supporting organizations) created between 1956 and 1986, only 3 failed (Whyte and Whyte 1991:3). The number of cooperatives now exceeds 160 industrial and service organizations, and the complex as a whole is recognized as one of the most successful industrial complexes in Europe (see Mondragon 2003, 2005). There has also been success in terms of creating jobs. Employment grew from 23 workers in 1956, to 25,322 in 1992, and to 68,200 in 2003 (Mondragon 2003, 2005; Whyte and Whyte 1991:3).
For a different perspective on the success of the Mondragon experiment—one that focuses much more on the political aspects and implications of the Mondragon experiment—see Kasmir (1996). Writing the results of her ethnographic study from a working-class perspective, Kasmir argues that workers in cooperatives face the same strains as do those not in cooperatives—shift work, assembly line work, routinization of tasks, and demands for everincreasing productivity. Furthermore, she insists that the cooperatives have political implications. For example, they divide the working class—those in cooperatives from those not in cooperatives—in terms of trying to achieve working-class goals.
Sweden’s Automotive Assembly System
In the 1960s, Swedish auto companies faced a labor crisis consisting of very high rates of turnover (which approached 100 percent per year), high rates of both shortterm and long-term absenteeism, and the inability to recruit new workers. Searching for an answer to their labor crisis, the Swedish automobile industry leaders discovered the results of studies by sociologists working in industry— especially those studying workers on the assembly line. Based on the results of the studies, those leaders began to completely revamp their production processes (Berggen 1992; Freyssenet 1998).
Volvo was a leader in the changes as it experimented with a series of different assembly systems. All the systems with which they experimented had two distinct features. First, they represented efforts to eliminate the traditional assembly system by having teams assemble major components—for example, an engine or a transmission. Second, they replaced the traditional shop floor hierarchy with work groups responsible for shop floor assembly decisions. The role of the foremen was changed to that of coordinating and planning the activities of the work groups and of providing the logistical and informational support for the activities of the groups. Volvo’s Kalmar plant—the first plant designed with the new assumptions—opened in 1974. At that time, it was the world’s first auto assembly plant without mechanically driven assembly lines. Speaking of the importance of the Kalmar plant, Berggren (1992) suggests that it was important in several ways; it demonstrated that there were feasible alternatives to the traditional rigid assembly line, that a small factory could produce efficiently because it was more productive than a Volvo plant five times as large, and that a small plant could produce high-quality products because in one of the years of its operation its cars had the highest standards in the history of Volvo (p. 129).
In 1993, Volvo closed Uddevalla—a three-year-old plant designed with their new automotive production principles. Some argued that the failure of the plant cast doubt on the potential success of Volvo’s principles, whereas others argued the closure could be explained by other factors (for the debate, see Adler and Cole 1993; Berggren 1994).
A Critique of the Japanese Lean Automotive Production Model
In 1982, Japanese automobile transplants first appeared in the United States with the opening of the Honda plant in Marysville, Ohio (Graham 1995:6). The success of the Japanese automobile industry relative to that of the U.S. automobile industry spurred industrial sociology research into the nature of organizational and management practices of the Japanese. That research agrees that Japanese management practices are as authoritarian as they are under scientific management (Berggren 1992; Graham 1993, 1995). In fact, management—especially in the guise of the foremen—seems to have even greater authority and decision-making power than ever. There are strict and precise management controls concerning (a) the distribution of power—workers have virtually no decision-making authority at all, (b) the way a worker works, (c) the way a worker dresses (he or she will wear company uniforms), and (d) the way the worker thinks (under the “Kaizan” system of continuous improvement, a worker who does not constantly think of new ways of improving productivity is assumed to have the “wrong” attitude and will be sanctioned or even fired) (Berggren 1992).
The Quality Revolution
The Quality Revolution refers to the increasing emphasis by consumers for quality goods and services; it is a label for a revolution of rising expectations in terms of quality. Numerous investigations, including those by industrial sociologists, documented how this revolution affected the operations, success, and sometimes failure of U.S. organizations (Dobyns and Crawford-Mason 1991; Kanter 1989; Main 1994; Thurow 1992; Womack, Jones, and Roos 1990). Japanese companies provided the stimulus for this revolution when, after World War II, they emphasized quality in production. Womack, Jones, and Roos (1990), based on their multiyear study of the automobile industry, stated,
Today, Toyota assembly plants have practically no rework areas and perform almost no rework. . . . American buyers report that Toyota’s vehicles have among the lowest number of defects of any in the world, comparable to the very best of the German luxury car producers, who devote many hours of assembly-plant effort to rectification. (Pp. 57–58)
American companies were forced to change their operations, adapt to the new production standards, or go out of business.
Workplace Democracy
As U.S. industrial organizations struggled with the challenges of the Quality Revolution and with the negative consequences of Taylor’s Scientific Management, many analysts concluded that the power and authority that were once restricted to management should be redistributed throughout the organization (Blumberg 1968; Fantasia, Clawson, and Graham 1988; Grenier 1988; Guest 1957, 1987; Hodson 1996; Hodson et al. 1993; Kanter 1995; Knights and Collinson 1985; Kornbluh, 1984; Parker 1985; Parker and Slaughter 1988; Peters 1987; Ramsay 1977; Safizadeh 1991; Sorge 1976; Thomas 1985; Turner 1991). Efforts to redistribute this power have various labels— Workplace Democracy, Worker Participation, Participative Management. These efforts range from moderate “finetuning” of the traditional worker-management relationships to radical revisions of them. This range can be categorized into four major groupings: (1) humanization of work, (2) labor-management quality-of-work-life (QWL) committees, (3) worker-owned companies, and (4) workerowned/worker-managed companies (Zwerdling 1978a).
Humanization of work experiments are explicit attempts to improve productivity by improving the workers’ QWL. Their underlying assumption is that by improving the QWL, the worker will feel better about work, and if the worker feels better about work, he or she will be a more productive worker.
Labor-management QWL committees experiments represent a more radical step in that they involve significant changes in the power relationships between labor and management because the worker has meaningful power over his or her working conditions. The basic assumption of these experiments is that improving the QWL is a worthy goal in and of itself and that one of the best ways to improve the worker’s QWL is to give him or her real power.
Worker-owned company experiments are those in which workers actually own but do not manage the company. The workers own all or part of the company; that ownership may involve a coequal share of the company or may involve unequal ownership. Worker-owned company experiments are particularly important for labormanagement relations for two reasons. First, they change the workers’ attitudes toward the company because the company belongs to them, and they know their economic future is tied to that of the company. Second, with ownership, workers can have a meaningful say in both the policy and the production decisions that affect their lives. In other words, such experiments have the potential of bringing democracy to the workplace.
Worker-owned/worker-managed companies are obviously the most radical of the workplace democracy experiments and are, therefore, the most infrequently tried. Zwerdling (1978a) suggests that a “true” workerowned/worker-managed company has the following characteristics. First, it is owned and operated by the people who work in it: Only the workers have control. Second, there is no stock, since stock implies that control is turned over to someone else. If capital is needed, the company uses debt financing. Third, all profits, in excess of operating expenses and investments in productivity enhancement, are divided equally among all workers. Fourth, it is run democratically. All workers regardless of skill and experience make decisions on how the business is run. Each worker has one and only one vote. Fifth, although workers can loan money to the company, their loan will be treated like any other loan and will not entitle them to any special privileges because those special privileges would conflict with the democratic principles on which the organization is based. The Mondragon system discussed earlier is an example of such an organization (for American examples, see Pencavel 2001; Perry 1978; Zwerdling 1978b).
Needed Investigations
There are several industrial sociological topics that deserve thorough investigations. Among them are a sociologybased explanation for the British Industrial Revolution, a sociological understanding of the great depressions, and an exploration of the impacts of globalization.
A Sociology-Based Explanation for the British Industrial Revolution
Given the profound consequences of industrialization for the organization not only of work but also of society itself, it is surprising that relatively little sociological effort has been invested in explaining why the first Industrial Revolution—the one that occurred in Great Britain approximately in 1750 to 1850—occurred (for two such sociological explanations, see Brown 1966; Campbell 1987). To date, the best explanation is an ecological one (Charlton 1986; Wilkinson 1973). In an important study dealing explicitly with ecological analysis and cultural evolution, Wilkinson (1973) argues that the underlying explanation for the Industrial Revolution can be found in ecological factors. He states,
The ecological roots of the English industrial revolution are not difficult to find. The initial stimulus to change came directly from resource shortages and other ecological effects of an economic system expanding to meet the needs of a population growing within a limited area. (P. 112)
He illustrates the process by describing how the timber shortage caused by the cutting down of the forests of England resulted in the shift to coal as the country’s principal energy source. That shift, in turn, led to the invention of Newcomen’s atmospheric engine (which was eventually modified by James Watt into a steam engine) because of the need to pump water out of the flooded coal mines. In effect, he argues that Great Britain’s Industrial Revolution was a series of necessary adaptations resulting from the degradation of an environment whose carrying capacity had been exceeded.
Although there is convincing evidence that environmental changes played an important role in the British Industrial Revolution, single-factor explanations for such an historical event should be considered suspect. The driving force in Wilkinson’s theory is population increase. Yet for the century preceding the Industrial Revolution, the population of England was relatively stable (Deane 1965:11; Wilkinson 1973:71). Why, starting at about 1750, did the population of England dramatically increase? It is reasonable to assume that social factors played a part.
A Sociological Understanding of the Great Depressions
Industrial societies endure depressions—severe economic downturns characterized by drastic declines in production and extremely high levels of unemployment. There have been three economic depressions so far. While there is widespread acknowledgement of the Great Depression of the 1930s, there is little acknowledgement of the two prior depressions endured by industrial societies. The United States was not industrialized enough to be severely affected by the first depression—the one that devastated England from roughly 1820 to the mid-1840s (Gordon 1978). However, the industrial boom that the United States experienced after the American Civil War resulted in an industrial nation susceptible to economic fluctuations, and it was hard-hit by the second depression. For almost 20 years, starting in 1873, the economies of the United States and other industrialized nations endured what economic analysts at that time called the “Great Depression” (Gordon 1978; for a good summary of that depression, see Parshall 1992). In the United States, that depression resulted in such a massive concentration of business power that an alarmed federal government was forced to intervene as “trust busters” during the first two decades of the twentieth century. The Great Depression of the 1930s changed the United States in even more fundamental ways than had the second depression. In response to the collapse of the American economy, the National Industrial Recovery Act was passed to give the federal government extraordinary powers to intervene in the economy. The federal government was also forced to provide massive support to the U.S. economy (Watkins 1993). That support continues today: the housing industry is supported by legislation that allows homeowners to claim as tax deductions interest paid on home mortgages, the agricultural industry is supported by a multi-billion-dollar farm subsidy program, and numerous businesses are protected from international competition by high import tariff and import quotas. Given the enormous social organization consequences of depressions, it is curious that industrial sociologists have not devoted more time and effort to describe the depressions and their consequences and understand why they occur.
Understanding the Implications of Globalization
Markets were once primarily restricted to small geographic areas because of the limitations of transportation systems. As transportation systems developed, markets became regional and then national. Today, neither consumer nor labor markets are national. Consumers have access to products and services from a world market. And those customers are increasingly taking advantage of that world market. They demand quality products and services, and they use that world market in their search for those products and services. Consumers now have access to a world economy . Furthermore, labor markets are becoming international because companies can now “source” their production worldwide (Friedman 2005). That is, they can shift their jobs to whatever location they decide is best for their company, irrespective of the effects of these shifts for the workers, communities, and countries they leave.
The potential consequences of changes in both these markets for the status of industrial organizations and their workers are profound. As Kanter (1995) notes, “Globalization is surely one of the most powerful and pervasive influences on nations, businesses, workplaces, communities, and lives at the end of the twentieth century” (p. 11). There is currently great concern and divergence of opinion about globalization. There are those who focus on the potential of globalization for all societies, not just industrial ones (Friedman 2005). There are others who describe the tremendous costs of globalization. The opposition to the North American Free Trade Agreement and the often violent protests whenever the World Trade Organization meets illustrate their concern (see also Gern 1995; Kamala 1998; Lewis 2002; Michalowski and Kramer 1987 for other expressions of concern). Investigations into globalization have documented benefits (Firebaugh and Goesling 2004) and costs (Horn 1993; Sass 2000; Storm and Rao 2004). Further investigations by industrial sociologists into the implications of globalization seem warranted (for examples of such investigations, see Ciccantell and Bunker 2004; Howes 1993; Johnson 1991, 2002; Kanter 1991, 1995; Kanter and Corn 1994; Kappel 1995; Perrucci 1994; Reich 1991; Ross and Trachte 1990; Sallaz 2004; Wolf 2005).
The Future of Industrial Societies
The social sciences have documented numerous instances of societal collapse (Catton 1993; Diamond 2005; Tainter 1988). Investigators are intrigued by the survival potential of industrial societies. What are the possibilities?
Every production system negatively affects the environment in some way. The degree and permanence of that environmental degradation, however, varies tremendously. In some cases, it is very limited and short term, whereas in others it is extensive and long term. As societies become larger and more complex, their environmental degradation becomes more pervasive and more permanent.
The degradation is a threat to both individuals and society. In the 1960s, the USSR dumped huge quantities of highly radioactive waste into Lake Karachay. The lake is now so radioactive that anyone standing on its shore for an hour or two will receive a lethal dose of radiation (Lenssen 1992:53). In the early 1980s, the town of Times Beach, Missouri, suffered severe, widespread chemical contamination. Rather than attempt the very costly procedure of rectifying the environmental damage, the U.S. Environmental Protection Agency bought out the residents and declared the town off-limits for humans (Boraiko 1985).
The threat of environmental degradation also extends to the actual survival of society itself. There are numerous instances of societies that have overexploited their resources and degraded their environment to such an extent that the society collapsed. Three of the most well-known examples are Easter Island, the Classic Maya, and the Anasazi of the U.S. Desert Southwest (Catton 1993; Diamond 2005, chap. 4; Pennsylvania State University and WQED 1993; Thorne 1989). (For other examples of societal collapse, see Chedd 1980; Diamond 2005; Tainter 1988.)
What, then, can be said about the future of industrial society? After all, industrial societies are among the largest and most complex of all societies and create some of the most pervasive and permanent environmental degradation. Predictions of the future of industrial society differ greatly. This range of alternatives can be collapsed into three major categories: pessimistic, moderate, and optimistic.
Pessimists point out that industrial societies are complex, resource-consuming, and environmentally degrading societies. The dismal history of other such societies suggests that industrial societies have a limited life span. Societal complexity, high rates of resource consumption, and extensive environmental degradation all seem incompatible with societal longevity. Few, if any , complex societies have survived for even a thousand years. Some analysts (Daily, Ehrlich, and Ehrlich 1994; Pimentel et al. 1994) claim that the world community has already exceeded the world’s carrying capacity and the resultant environment degradation will inevitably lead to the collapse of society. An early investigation into the consequences of human population growth was conducted by researchers at the Massachusetts Institute of Technology (Meadows et al. 1974). They conducted a series of computer simulations focusing on “the five basic factors that determine, and, therefore, ultimately limit, growth on this planet—population, agricultural production, natural resources, industrial production, and pollution” (Meadows et al. 1974:xi). In the course of their investigation, they systematically varied the value of each of the crucial factors. Despite changing the assumptions, the end result was almost always the same. The system continued to grow beyond what could be sustained and collapsed within a hundred years. Their computer models indicated that there was only one possible set of conditions that would stabilize the system and that was to simultaneously control population and industrial output. In other words, industrial societies had to be radically redesigned. Two decades later, the team updated their original study (Meadows et al. 1992). They discovered that their original time frame was wrong. Their analyses suggested that without substantial change—not just minor “fine-tuning”—industrial society would collapse in as little as 20 years. Pessimists, then, argue that industrial societies as they currently operate cannot survive, and that without substantial change, industrial society as we know it will collapse. In fact, some argue that the negative impacts of such societies are so severe that they should not survive (Lewis 2002).
Proponents of moderate scenarios share with the pessimists the common theme that industrial society is sustainable indefinitely only if changes are made in its basic operating assumptions (Dobkowski and Wallimann 2002). Whether they argue for developing a “steady-state economy” (Daly 1973; Postel and Flavin 1991), or for the importance of building a “sustainable society” (Brown 1981), or for the value that “small is beautiful” (Schumacher 1973), they insist that industrial societies can survive long term only if the premises on which they are based are substantially changed. And the core change centers on the concept of sustainability. In a sustainable society, renewable resources are used at a rate that ensures the indefinite survival of the resource, while the use of nonrenewable resources is de-emphasized or even abandoned. In sum, proponents of moderate scenarios are optimistic about the future of industrial societies. They argue that moderate, not radical, changes in the operation of industrial societies will allow industrial societies to survive.
Optimists insist that despite all the problems facing industrial societies, the future is not bleak but is instead filled with possibilities. They suggest that not only are industrial societies not threatened by the problems that have been documented but also that the problems may ultimately disappear as pressing human concerns (Budiansky 1994; Simon 1981). The optimists focus their attention on what they believe to be a misplaced emphasis on the problems of overpopulation and resource limits. They argue that even if the pessimists are right about resource limits, technological innovations will overcome any problems created by the limits. They note, for example, that as copper has become more expensive, fiber-optic cable has been used in its place (Simon 1981). According to the optimists, then, the future of industrial society is bright. The creativity and innovation of people in industrial societies and the productivity of industrial production systems will yield increased wealth and a better quality of life for all. Population growth will cease to be a problem, and resources will become more abundant and less expensive (Simon 1981).
There is little reason to believe that the subdiscipline of industrial sociology will ever attain its former prominence. However, given the importance of work in industrial societies, there is little doubt that there will continue to be theoretically and practically important investigations into industrial sociology topics.
Bibliography:
- Adler, Paul and Robert Cole. 1993. “Designed for Learning: A Tale of Two Auto Plants.” Sloan Management Review 34(Spring):85–94.
- Berggren, Christian. 1992. Alternatives to Lean Production: Work Organization in the Swedish Auto Industry. Ithaca, NY: ILR Press.
- Berggren, Christian. 1994. “NUMMI versus Uddevalla.” Sloan Management Review 35(Winter):37–45.
- Blauner, Robert. 1964. Alienation and Freedom:The Factory Worker and His Industry. Chicago, IL: University of Chicago Press.
- Bluestone, Barry and Bennett Harrison. 1982. The Deindustrialization of America: Plant Closings, Community Abandonment, and the Dismantling of Basic Industry. New York: Basic Books.
- Blumberg, Paul. 1968. Industrial Democracy: The Sociology of Participation. New York: Schocken Books.
- Boraiko, Allen A. 1985. “Storing Up Trouble: Hazardous Waste.” National Geographic, March, pp. 318–51.
- Braverman, Harry. 1974. Labor and Monopoly Capital: The Degradation of Work in the Twentieth Century. New York: Monthly Review Press.
- Brown, Lester R. 1981. Building a Sustainable Society. New York: W. W. Norton.
- Brown, Murray. 1966. “Toward an Endogenous Explanation of Industrialization.” Social Research 33(2):295–313.
- Budiansky, Stephen. 1994. “10 Billion for Dinner, Please.” S. News & World Report, September 12, pp. 57–60.
- Campbell, Colin. 1987. The Romantic Ethic and the Spirit of Modern Consumption. Oxford, England: Basil Blackwell.
- Carey, Alex. 1967. “The Hawthorne Studies: A Radical Criticism.” American Sociological Review 32:403–16.
- Catton, William R., Jr. 1993. “Carrying Capacity and the Death of a Culture:A Tale of Two Autopsies.” Sociological Inquiry 63(2):202–23.
- Chang, Clara and Constance Sorrentino. 1991. “Union Membership Statistics in 12 Countries.” Monthly Labor Review 114(12):46–53.
- Charlton, Michael. 1986. “The Revolution of Necessity.” Episode 4 of the video series Out of the Fiery Furnace, produced by Robert Raymond . Melbourne, Australia: Magnum Opus Films.
- Chedd, Graham. 1980. The Chaco Legacy, produced by Michael Ambrosino. Boston, MA: Odyssey Series.
- Chinoy, Ely. 1955. Automobile Workers and the American Dream. Boston, MA: Beacon Press.
- Ciccantell, Paul S. and Stephen G. Bunker. 2004. “The Economic Ascent of China and the Potential for Restructuring the Capitalist World-System.” Journal of World-Systems Research 10(3):565–89.
- Clawson, Dan and Mary A. Clawson. 1999. “What Has Happened to the US Labor Movement? Union Decline and Renewal.” Annual Review of Sociology 25:95–119.
- Cole, Robert E. 1971. Japanese Blue-Collar: The Changing Tradition. Berkeley: University of California Press.
- Daily, Gretchen, Anne H. Ehrlich, and Paul R. Ehrlich. 1994. “Optimum Human Population Size.” Population and Environment: A Journal of Interdisciplinary Studies 15(6):469–75.
- Daly, Herman E. 1973. “The Steady-State Economy: Toward a Political Economy of Biophysical Equilibrium and Moral Growth.” Pp. 149–74 in Toward a Steady-State Economy , edited by H. E. Daly. San Francisco, CA: W. H. Freeman.
- Deane, Phyllis. 1965. The First Industrial Revolution. Cambridge, England: Cambridge University Press.
- Diamant,A. 1977. “Democratizing the Workplace: The Myth and Reality of Mitbestimmung in the Federal Republic of Germany.” Pp. 25–48 in Worker Self-Management in Industry: The West European Experience, edited by G. D. Garson. New York: Praeger.
- Diamond, Jared. 2005. Collapse: How Societies Choose to Fail or Succeed. New York: Penguin Group.
- Dobkowski, Michael N. and Isidor Wallimann. 2002. “Introduction: On the Edge of Scarcity.” Pp. xxi–xxxi in On the Edge of Scarcity: Environment, Resources, Population, Sustainability, and Conflict, edited by M. N. Dobkowski and I. Wallimann. Syracuse, NY: Syracuse University Press.
- Dobyns, Lloyd and Clare Crawford-Mason. 1991. Quality or Else: The Revolution in World Business. Boston, MA: Houghton Mifflin.
- Economic Report of the President, 1994. February. Washington, DC: Council of Economic Advisors.
- Fantasia, Rick, Dan Clawson, and Gregory Graham. 1988. “A Critical View of Worker Participation in American Industry.” Work and Occupations 15:468–88.
- Farber, Henry S. and Alan B. Krueger. 1993. “Union Membership in the United States: The Decline Continues.” Pp. 105–34 in Employee Representation: Alternatives and Future Directions, edited by Bruce E. Kaufman and Morris M. Kleiner. Madison, WI: Industrial Relations Research Association.
- Firebaugh, Glenn and Brian Goesling. 2004. “Accounting for the Recent Decline in Global Inequality.” American Journal of Sociology 110(2):283–312.
- Form, William. 1976. Blue-Collar Stratification: Auto Workers in Four Countries. Princeton, NJ: Princeton University Press.
- Form, William and Kyu Han Rae. 1988. “Convergence Theory and the Korean Connection.” Social Forces 66(3):618–44.
- Franke, Richard H. and James D. Kaul. 1978. “The Hawthorne Experiments: First Statistical Interpretation.” American Sociological Review 43(5):623–43.
- Freeman, Richard B. and James L. Medoff. 1984. What Do Unions Do? New York: Basic Books.
- Frege, Carola. 2003. “Transforming German Workplace Relations: Quo Vadis Cooperation.” Economic and Industrial Democracy 24(3):317–47.
- Freyssenet, Michel. 1998. “Reflective Production: An Alternative to Mass Production and Lean Production?” Economic and Industrial Democracy 19(1):91–117.
- Friedman, Thomas L. 2005. The World Is Flat: A Brief History of the Twenty-first Century. New York: Farrar, Straus & Giroux.
- Furstenberg, Friedrich. 1977. “West German Experience with Industrial Democracy.” Annuals of the American Academy of Political and Social Science 431(May):44–53.
- Geoghegan, Thomas. 1991. Which Side Are You On? Trying to Be for Labor When It Is Flat on Its Back. New York: Plume.
- Gern, Jean-Peirre. 1995. “Economic Globalization and Growing Anomie.” International Journal of Sociology and Sociology Policy 15:8–10, 65–76.
- Gersuny, Carl. 1981. Work Hazards and Industrial Conflict. Hanover, NH: University Press of New England for the University of Rhode Island.
- Ginsbourger, Francis. 1981. “Japan’s Dark Side,” World Press Review (July):32–33.
- Goldman, Paul and Donald R. Van Houten. 1981. “Bureaucracy and Domination: Managerial Strategy in Turn-of-theCentury American Industry.” Pp. 89–216 in Complex Organizations: Critical Perspectives, edited by M. ZeyFerrell and M. Aiken. Glenview, IL: Scott Foresman.
- Gordon, David M. 1978. “Up and Down the Long Roller Coaster.” Pp. 22–35 in S. Capitalism in Crisis, prepared by the Crisis Reader Editorial Collective . New York: Union for Radical Political Economics.
- Gottfried, Heidi. 1998. “Whither Taylorism?” Work, Employment, and Society 12(3):545–49.
- Graham, Laurie. 1993. “Inside a Japanese Transplant: A Critical Perspective.” Work and Occupations 20(2):147–73.
- Graham, Laurie. 1995. On the Line at Subaru-Isuzu: The Japanese Model and the American Worker. Ithaca, NY: ILR Press.
- Grenier, Guillermo. 1988. Inhumane Relations: Quality Circles and Anti-Unionism in American Industry. Philadelphia, PA: Temple University Press.
- Guest, Robert H. 1957. “Job Enlargement: A Revolution in Job Design.” Personnel Administration 20:9–16.
- Guest, Robert H. 1987. “Industrial Sociology: The Competitive Edge.” Footnotes, January, pp. 5, 8.
- Harrington, Michael. 1977. The Vast Majority: A Journey to the World’s Poor. New York: Simon & Schuster.
- Harris, Marvin. 1977. Cannibals and Kings: The Origins of Cultures. New York: Random House.
- Harrison, Bennett and Barry Bluestone. 1988. The Great U-Turn: Corporate Restructuring and the Polarizing of America. New York: Basic Books.
- Harvey, Edward B. 1975. Industrial Society: Structures, Roles, and Relations. Homewood, IL: The Dorsey Press.
- Hill, Stephen. 1981. Competition and Control at Work: The New Industrial Sociology. Cambridge: MIT Press.
- Hodson, Randy. 1996. “Dignity in the Workplace under Participative Management: Alienation and Freedom Revisited.” American Sociological Review 61(5):719–38.
- Hodson, Randy, Sandy Wessh, Sabine Rieble, Cheryl Sorenson Jamison, and Sean Creighton. 1993. “Is Worker Solidarity Undermined by Autonomy and Participation? Patterns from the Ethnographic Literature.” American Sociological Review 58(June):398–416.
- Horn, Patricia. 1993. “Paying Top Lose Our Jobs: The U.S.’s Job Export Strategy.” Dollars and Sense, March, pp. 10–11, 20–21.
- Howes, Candice. 1993. Japanese Auto Transplants and the U.S. Automobile Industry. Washington, DC: Economic Policy Institute.
- Inkeles, Alex. 1960. “Industrial Man: The Relation of Status to Experience, Perception, and Value.” American Journal of Sociology 66(1):1–31.
- Inkeles, Alex and Peter H. Rossi. 1961. “National Comparisons of Occupational Prestige.” Pp. 506–16 in Sociology, edited by S. M. Lipset and N. J. Smelser. Englewood Cliffs, NJ: Prentice Hall.
- Johnson, Ana Gutierrez and William F. Whyte. 1977. “The Mondragon System of Worker Production Cooperatives.” Industrial and Labor Relations Review 31(1):18–30.
- Johnson, Christopher H. 2002. “Introduction: De-Industrialization and Globalization.” International Review of Social History, 47(Suppl. 10, December):3–33.
- Johnson, William B. 1991. “Global Work Force 2000: The New World Labor Market.” Harvard Business Review 69(March–April):115–27.
- Jones, Stephen R. G. 1992. “Was There a Hawthorne Effect?” American Journal of Sociology 98(3):451–68.
- Kamala, Bonala K. 1998. Globalization: The Perils for Vulnerable Groups. International Sociological Association.
- Kanter, Rosabeth M. 1977. Men and Women of the Corporation. New York: Basic Books.
- Kanter, Rosabeth M. 1983. The Change Masters: Innovation & Entrepreneurship in the American Corporation. New York: Simon & Schuster.
- Kanter, Rosabeth M. 1989. When Giants Learn to Dance. New York: Simon & Schuster.
- Kanter, Rosabeth M. 1991. “Transcending Business Boundaries: 12,000 World Managers View Change.” Harvard Business Review 69(May–June):151–64.
- Kanter, Rosabeth M. 1995. World Class: Thriving Locally in the Global Economy. New York: Touchstone.
- Kanter, Rosabeth M. and Richard I. Corn. 1994. “Do Cultural Differences Make a Business Difference? Contextual Factors Affecting Cross-Cultural Relationship Success.” Journal of Management Development 13(Winter):5–23.
- Kappel, Robert. 1995. “Core and Periphery in the Global Order: Globalization, Tripolarity and Marginalization” (in German). Peripherie 15(December):59–60, 79–117.
- Kasmir, Sharryn. 1996. The Myth of Mondragon: Cooperatives, Politics, and Working-Class Life in a Basque Town. Albany: State University of New York Press.
- Kassalow, Everett M. 1984. “The Future of American Unionism: A Comparative Perspective.” Annals of the American Association of Political and Social Scientists 73(May): 52–63.
- Kerbo, Harold R. 2006. Social Stratification and Inequality: Class Conflict in Historical, Comparative, and Global Perspective. 6th ed. New York: McGraw-Hill.
- Kimeldorf, Howard and Judith Stephan-Norris. 1992. “Historical Studies of Labor Movement in the United States.” Annual Review of Sociology 18:495–517.
- Knights, David and David Collinson. 1985. “Redesigning Work on the Shop Floor: A Question of Control and Consent.” Pp. 197–226 in Job Redesign: Critical Perspectives on the Labor Process, edited by D. Knights, H. Willmott, and D. Collinson. Aldershot, England: Gower.
- Kornbluh, Hy. 1984. “Work Place Democracy and Quality of Work Life: Problems and Prospects.” Annals of American Academy of Political and Social Science 473(May):88–95.
- Lenssen, Nicholas. 1992. “Confronting Nuclear Waste.” Pp. 46–65 in State of the World, 1992, edited by Lester R. Brown. New York: Norton.
- Lewis, Chris H. 2002. “Global Industrial Collapse: The Necessary Collapse.” Pp. 16–29 in On the Edge of Scarcity: Environment, Resources, Population, Sustainability, and Conflict, edited by M. N. Dobkowski and I. Wallimann. Syracuse, NY: Syracuse University Press.
- Main, Jeremy. 1994. Quality Wars: The Triumphs and Defeats of American Business. New York: Free Press.
- Marsh, Robert M. 1984. “Whither the Comparative Study of Modernization and Development?” Pp. 81–106 in Work, Organizations, and Society, edited by M. B. Brinkerhoff. Westport, CT: Greenwood Press.
- Marsh, Robert M. and Hiroshi Mannari. 1976. Modernization and the Japanese Factory. Princeton, NJ: Princeton University Press.
- McGinnis, James B. 1979. Bread and Justice: Toward a New International Economic Order. New York: Paulist Press.
- Meadows, Donella H., Dennis L. Meadows, Jorgen Randers, and William W. Behrens III. 1974. The Limits to Growth. 2d ed. New York: Potomac Associates Books.
- Meadows, Donella H., Dennis L. Meadows, and Jorgen Randers. 1992. Beyond the Limits. Post Hills, VT: Chelsea Green.
- Michalowski, Raymond J. and Ronald C. Kramer. 1987. “The Space between Laws: The Problem of Corporate Crime in a Transnational Context.” Social Problems 34(1):34–53.
- Miller, Delbert C. 1984. “Whatever Will Happen to Industrial Sociology.” Sociological Quarterly 25(Spring):251–56.
- Mondragon Corporacion Cooperativa. https://www.mondragon-corporation.com/en/
- Naoi, Atsushi and Carmi Schooler. 1985. “Occupational Conditions and Psychological Functioning in Japan.” American Journal of Sociology 90(4):729–52.
- Parker, Mike. 1985. Inside the Circle: A Union Guide to QWL. Boston, MA: South End.
- Parker, Mike and Jane Slaughter. 1988. Choosing Sides: Unions and the Team Concept. Boston, MA: South End.
- Parshall, Gerald. 1992. “The Great Panic of ’93.” S. News & World Report, November 2, pp. 70, 72.
- Pencavel, John. 2001. Worker Participation: Lessons from the Worker Co-Ops of the Pacific Northwest. New York: Russell Sage Foundation.
- Pennsylvania State University and WQED. 1993. “The Collapse.” Program #8 of Out of the Past. Burlington, VT: Annenberg/CPB Collection.
- Perrucci, Robert. 1994. Japanese Auto Transplants in the Heartland. Hawthorne, NY: Aldine de Gruyter.
- Perry, Stewart E. 1978. San Francisco Scavengers: Dirty Work and the Pride of Ownership. Berkeley: University of California Press.
- Peters, Tom. 1987. Thriving on Chaos: Handbook for a Management Revolution. New York: HarperPerennial.
- Pimentel, David, Rebecca Harman, Matthew Pacenza, Jason Pecarsky, and Marcia Pimentel. 1994. “Natural Resources and an Optimum Human Population.” Population and Environment: A Journal of Interdisciplinary Studies 15(5):347–69.
- Postel, Sandra and Christopher Flavin. 1991. “Reshaping the Global Economy.” Pp. 170–88 in State of the World: 1991, edited by L. R. Brown. New York: W. W. Norton.
- Putman, John J. 1977. “West Germany: Continuing Miracle.” National Geographic, August, pp. 151–81.
- Ramsay, H. 1977. “Cycles of Control: Worker Participation in Sociological and Historical Perspective.” Sociology 11:481–506.
- Reich, Robert. 1991. The Work of Nations: Preparing Ourselves for 21st Century Capitalism. New York: Knopf.
- Roethlisberger, Fritz J. and William J. Dickson. 1939. Management and the Worker: An Account of a Research Program Conducted by the Western Electric Company, Hawthorne Works, Chicago. Cambridge, MA: Harvard University Press.
- Ross, Robert J. S. and Kent C. Trachte. 1990. Global Capitalism: The New Leviathan. Albany: State University of New York.
- Rothstein, Frances and Michael L. Blim, eds. 1992. Anthropology and the Global Factory: Studies of the New Industrialization in the Late Twentieth Century. New York: Bergin & Garvey.
- Rowley, R. Kent. 1977. “A Skeptical View of the West German Model.” Pp. 219–21 in Industrial Democracy Today, edited by G. Sanderson and F. Stapenhurst. Toronto, Ontario, Canada: McGraw-Hill.
- Roy, Donald. 1952. “Quota Restriction and Goldbricking in a Machine Shop.” American Journal of Sociology 57:427–42.
- Roy, Donald. 1954. “Efficiency and the Fix: Informal Intergroup Relations in a Piece-Work Machine Shop.” American Journal of Sociology 60:255–66.
- Roy, Donald. 1958. “Banana Time: Job Satisfaction and Informal Interaction.” Human Organization 18:158–68.
- Safizadeh, M. Hossein. 1991. “The Case of Workgroups in Manufacturing Operations.” California Management Review 33(4):61–82.
- Sallaz, Jeffrey J. 2004. “Manufacturing Concessions:Attritionary Outsourcing at General Motor’s Lordstown, USA Assembly Plant.” Work, Employment and Society 18(4):687–708.
- Sass, Robert. 2000. “Agricultural “Killing Fields”: The Poisoning of Costa Rica Banana Workers.” International Journal of Health Services 30(3):491–514.
- Schumacher, E. F. 1973. Small Is Beautiful: Economics as if People Mattered. New York: Harper & Row.
- Simon, Julian L. 1981. The Ultimate Resource. Princeton, NJ: Princeton University Press.
- Simpson, Ida H. 1989. “The Sociology of Work: Where Have the Workers Gone?” Social Forces 67(3):563–81.
- Sorge, A. 1976. “The Evolution of Industrial Democracy in the Countries of the European Community.” British Journal of Industrial Relations 14:274–94.
- Statistical Abstract of the United States, 1997: The National Data Book. Washington, DC: U.S. Bureau of the Census.
- Statistical Abstract of the United States, 2004–2005: The National Data Book. Washington, DC: U.S. Bureau of the Census.
- Stover, Ronald G., Melodie L. Lichty, and Penny W. Stover. 1999. Industrial Societies: An Evolutionary Perspective. Englewood Cliffs, NJ: Prentice Hall.
- Storm, Servaas and J. Mohan Rao. 2004. “Market-Led Globalization and World Democracy: Can the Twain Ever Meet?” Development and Change 35(3):567–81.
- Tainter, Joseph A. 1988. The Collapse of Complex Societies. New York: Cambridge University Press.
- Taylor, Frederick W. 1911. Scientific Management. New York: Harper & Row.
- Taylor, Lee. 1968. Occupational Sociology. New York: Oxford University Press.
- Thomas, Robert J. 1985. “Quality and Quantity: Worker Participation in the U.S. and Japanese Automobile Industries.” Pp. 162–88 in Technological Change and Worker Movements, edited by M. Dubofsky. Beverly Hills, CA: Sage.
- Thorne, Alan. 1989. “The Feathered Serpent” episode. Man on the Rim: Peopling the Pacific. Falls Church, VA: Landmark Films.
- Thurow, Lester C. 1987. “A Surge in Inequality.” Scientific American 256(5):30–37.
- Thurow, Lester C. 1992. Head to Head: The Coming Economic Battle Among Japan, Europe, and America. New York: William Morrow.
- Troy, Leo. 1990. “Is the U.S. Unique in the Decline of Private Sector Unionism?” Journal of Labor Research 11(2): 111–43.
- Turner, Lowell. 1991. Democracy at Work: Changing World Markets and the Future of Labor Unions. Ithaca, NY: Cornell University Press.
- S. Bureau of Labor Statistics. 2005a. Labor Force Statistics from the Current Population Survey. Retrieved December 7, 2005 (ftp.bls.gov/pub/suppl/empsit.ceseeb16.txt).
- S. Bureau of Labor Statistics. 2005b. Union Members Summary. USDL 05–112. Retrieved December 6, 2005 (www.bls.gov/news.release/unioin2.nro).
- Walker, Charles R. 1950 . Steeltown: An Industrial Case History of the Conflict Between Progress and Security. New York: Harper.
- Walker, Charles R. and Robert H. Guest. 1952. The Man on the Assembly Line. Cambridge, MA: Harvard University Press.
- Walker, Charles R., Robert H. Guest, and Arthur N. Turner. 1956. The Foreman on the Assembly Line. Cambridge, MA: Harvard University Press.
- Watkins, T. H. 1993. The Great Depression: America in the 1930s. New York: Little, Brown.
- Weil, Simone. 1962. “Factory Work.” Pp. 452–57 in Man, Work, and Society: A Reader in the Sociology of Occupations, edited by S. Nosow and W. Form. New York: Basic Books.
- Weiler, Paul C. 1993. “Governing the Workplace: Employee Representation in the Eyes of the Law.” Pp. 81–104 in Employee Representation: Alternatives and Future Directions, edited by Bruce E. Kaufman and Morris M. Kleiner. Madison, WI: Industrial Relations Research Association.
- Wilkinson, Richard G. 1973. Poverty and Progress: An Ecological Perspective on Economic Development. New York: Praeger.
- Whyte, William F. 1968. “Elton Mayo.” Pp. 82–83 in The International Encyclopedia of the Social Sciences. New York: Macmillan.
- Whyte, William F. and Kathleen K. Whyte. 1991. Making Mondragon: The Growth and Dynamics of the Worker Cooperative Complex. Ithaca, NY: ILR Press.
- Wolf, Jamie. 2005. Globalization in the Steel Industry: The Market and Commodity Chain Transitions from Industrial to Post-Industrial Capitalism. Charlotte, NC: Southern Sociological Society.
- Womack, James P., Daniel T. Jones, and Daniel Roos. 1990. The Machine That Changed the World. New York: Rawson Associates.
- Zwerdling, Daniel. 1978a. “Intro.” Pp. 1–8 in Democracy at Work: A Guide to Workplace Ownership, Participation, & Self-Management Experiments in the United States & Europe, edited by D. Zwerdling . Washington, DC: Association for Self-Management.
- Zwerdling, Daniel. 1978b. “Cooperative Central.” Pp. 101–11 in Democracy at Work: A Guide to Workplace Ownership, Participation, & Self-Management Experiments in the United States & Europe, edited by D. Zwerdling . Washington, DC: Association for Self-Management.
Industrial sociology and industrial relations
An assessment of the contribution which industrial sociology can make towards understanding and resolving some of the problems now being considered by the royal commission., by fox, alan..
- 24 Want to read
- 1 Currently reading
- 1 Have read

Preview Book
My Reading Lists:
Use this Work
Create a new list
My book notes.
My private notes about this edition:
Check nearby libraries
Buy this book
This edition doesn't have a description yet. Can you add one ?
Previews available in: English
Showing 1 featured edition. View all 1 editions?
1 | Listen |
Add another edition?
Book Details
Edition notes, classifications, the physical object, source records, community reviews (0).
- Created April 1, 2008
- 9 revisions

Wikipedia citation
Copy and paste this code into your Wikipedia page. Need help?
Edited by | import existing book | |
Edited by | import existing book | |
Edited by | import existing book | |
Edited by | Added new cover | |
Created by an anonymous user | Imported from |
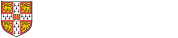
Study at Cambridge
About the university, research at cambridge.
- Undergraduate courses
- Events and open days
- Fees and finance
- Postgraduate courses
- How to apply
- Postgraduate events
- Fees and funding
- International students
- Continuing education
- Executive and professional education
- Courses in education
- How the University and Colleges work
- Term dates and calendars
- Visiting the University
- Annual reports
- Equality and diversity
- A global university
- Public engagement
- Give to Cambridge
- For Cambridge students
- For our researchers
- Business and enterprise
- Colleges & departments
- Email & phone search
- Museums & collections
- Department of Sociology
- Our History
- Job Vacancies
- Alumni overview
- Help support the Department of Sociology
- Alumni Benefits
- Alumni Events
- Academic Staff
- Affiliated Staff
- Postdoctoral and Research Staff
- Postgraduate Students
- Emeritus Academics
- Administrative Staff overview
- Paulina Baltsoukou
- Lara Gisborne
- Lucy O'Connor
- Lisa Watson
- Abigail Youngman
- Yvonne Frankfurth
- Yvonne Martin-Portugues
- Lucian Stephenson
- Undergraduates overview
- Part I overview
- Supervisions
- One-Year Part II
- Undergraduate Teaching FAQs
- Postgraduates overview
- Library Services
- Resources & Training
- Support & Wellbeing
- Cambridge University Sociology Society (SocSoc)
- Generative AI and your learning
- Why study Sociology?
- Undergraduate Study overview
- Course Structure
- Applying to Sociology
- Fees and Funding
- Guidance for Teachers
- Preparing for Interview
- Student Testimonials
- Support and Services
- Postgraduate Study overview
- Choosing a Supervisor
- Applications overview
- Postgraduate FAQs
Visiting Scholars
- Outreach & Open Days
- Y10-13 Photography Competition
- Events overview
- News overview
- Decolonise Sociology ↗
CUQM SOC5 prize 2024 winner announced
- Current Students
- Prospective Students

Submitted by A.B. Youngman on Thu, 04/07/2024 - 16:49
Congratulations to Charlotte Blixen-Finecke who has won the Cambridge Undergraduate Quantitative Methods SOC5 prize for the highest mark in the 2024 SOC5 (statistics and methods) exam paper.
Charlotte, pictured above, said, ‘Having recently finished my degree in Politics and Sociology (HSPS), I still look back at SOC5 as one of the most useful papers I have taken. Like many in HSPS, I was at first intimidated by the idea of statistical methods, but it really did feel like it was made for students with no previous knowledge on the topic and was always relevant to the study of sociology.
‘SOC5 has enabled me to conduct my own quantitative analyses in my dissertation and has more generally allowed me to better understand articles in both sociology and politics whenever they have included quantitative analyses. While sociology can sometimes feel like a subject with many more questions than answers, SOC5 is refreshing in its slightly more concrete methodology. When quantitative and qualitative methods are both considered, I have felt that sociology becomes slightly more approachable. I highly recommend anyone with the option to take the paper!’
The Department of Sociology University of Cambridge Free School Lane Cambridge CB2 3RQ
Tel: 01223 (3)34520
Contact: [email protected]
Privacy notice & cookie policies.
Website Updates
Profile Update Form
Research Project Form
Event Promotion Form
Tweet Request Form
Useful Information
Annual Reports
Equipment for Loan
IT Services Guide
twitterrrr.png

facebook_logo_square.png
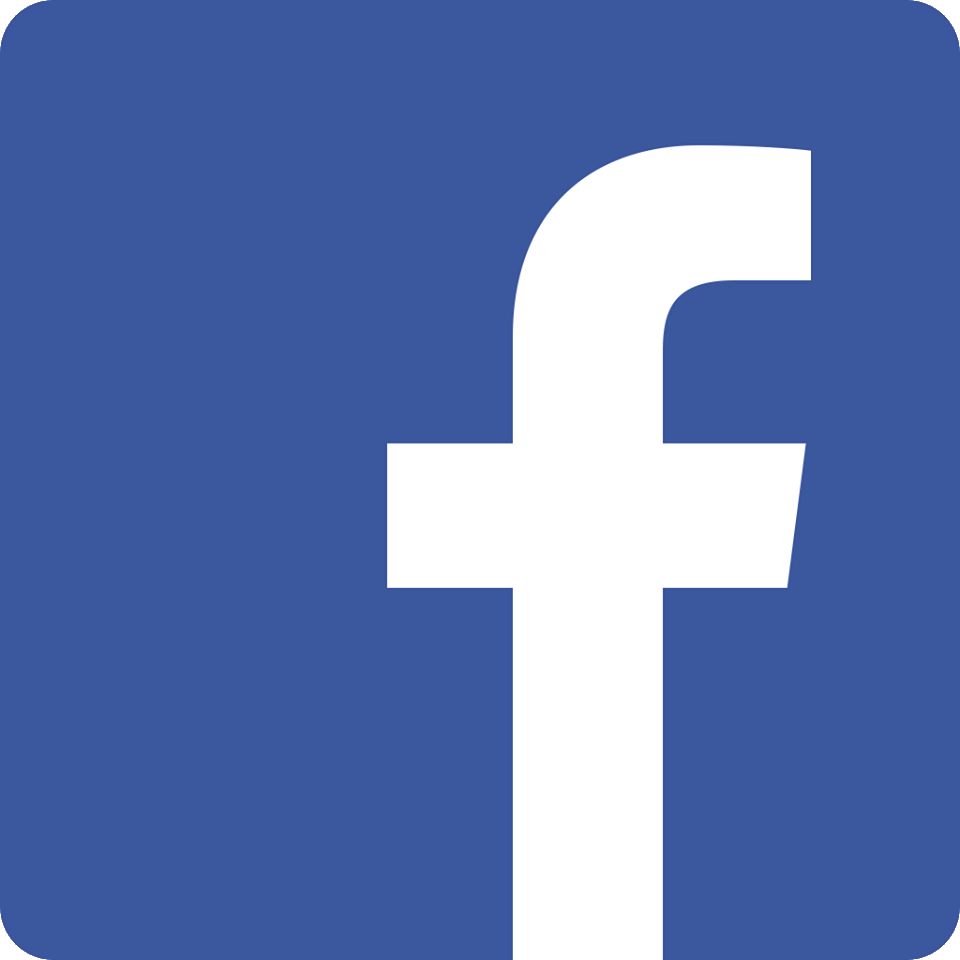
117156_media_512x512.png
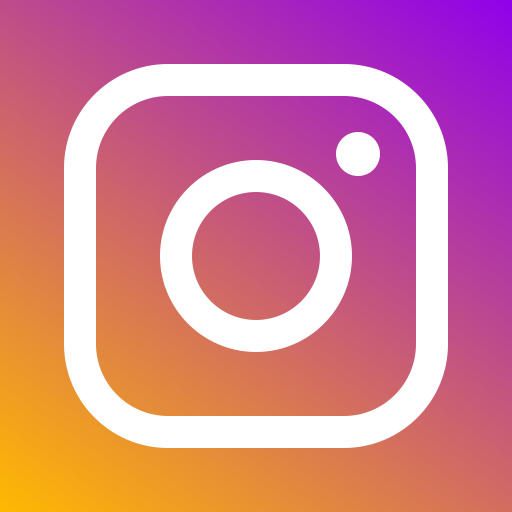
768px-youtube_play_button_square_2013-2017.svg_.png
© 2024 University of Cambridge
- Contact the University
- Accessibility
- Freedom of information
- Privacy policy and cookies
- Statement on Modern Slavery
- Terms and conditions
- University A-Z
- Undergraduate
- Postgraduate
- Research news
- About research at Cambridge
- Spotlight on...
- MyU : For Students, Faculty, and Staff

Four new CSE department heads begin in 2024-25

They bring a wealth of academic, research, and leadership abilities
MINNEAPOLIS / ST. PAUL (07/01/2024)—University of Minnesota College of Science and Engineering Dean Andrew Alleyne has named four new department heads in the college. All bring a wealth of academic, research, and leadership abilities to their departments.
Department of Chemical Engineering and Materials Science
Professor Kevin Dorfman has been appointed as the new d epartment h ead for the Department of Chemical Engineering and Materials Science (CEMS). Dorfman started his five-year term on July 1, 2024.
Dorfman joined the University of Minnesota faculty in January of 2006 and was quickly promoted up the ranks, receiving tenure in 2011, promotion to professor in 2015, and named a Distinguished McKnight Professor in 2020. He previously served as the director of undergraduate studies in chemical engineering from 2018-2022, where he headed a large-scale revision of the chemical engineering curriculum and saw the department through its most recent ABET accreditation.
His research focuses on polymer physics and microfluidics, with applications in self-assembly and biotechnology. He is particularly well known for his integrated experimental and computational work on DNA confinement in nanochannels and its application towards genome mapping. Dorfman’s research has been recognized by numerous national awards including the AIChE Colburn Award, Packard Fellowship in Science and Engineering, NSF CAREER Award, and DARPA Young Faculty Award.
Dorfman received a bachelor’s degree in chemical engineering from Penn State and a master’s and Ph.D. in chemical engineering from MIT.
Department of Industrial and Systems Engineering
Professor Archis Ghate has been appointed as the new Department Head for the Department of Industrial and Systems Engineering after a national search. Ghate will begin his five-year term on July 8, 2024.
Ghate is an expert in operations research and most recently served as the Fluor Endowed Chair in the Department of Industrial Engineering at Clemson University. Previously, he was a professor of industrial and systems engineering at the University of Washington. He has won several research and teaching awards, including an NSF CAREER Award.
Ghate’s research in optimization spans areas as varied as health care, transportation and logistics, manufacturing, economics, and business analytics. He also served as a principal research scientist at Amazon working on supply chain optimization technologies.
Ghate received bachelor’s and master’s degrees, both in chemical engineering, from the Indian Institute of Technology. He also received a master’s degree in management science and engineering from Stanford University and a Ph.D. in industrial and operations engineering from the University of Michigan.
Department of Mechanical Engineering
Professor Chris Hogan has been appointed as the new department head for the Department of Mechanical Engineering. Hogan started his five-year term on July 1, 2024.
Hogan, who currently holds the Carl and Janet Kuhrmeyer Chair, joined the University of Minnesota in 2009, and since then has taught fluid mechanics and heat transfer to nearly 1,000 undergraduates, advised 25+ Ph.D. students and postdoctoral associates, and served as the department’s director of graduate studies from 2015-2020. He most recently served as associate department head.
He is a leading expert in particle science with applications including supersonic-to-hypersonic particle impacts with surfaces, condensation and coagulation, agricultural sprays, and virus aerosol sampling and control technologies. He has authored and co-authored more than 160 papers on these topics. He currently serves as the editor-in-chief of the Journal of Aerosol Science . Hogan received the University of Minnesota College of Science and Engineering’s George W. Taylor Award for Distinguished Research in 2023.
Hogan holds a bachelor’s degree Cornell University and a Ph.D. from Washington University in Saint Louis.
School of Physics and Astronomy
Professor James Kakalios has been appointed as the new department head for the School of Physics and Astronomy. Kakalios started his five-year term on July 1, 2024.
Since joining the School of Physics and Astronomy in 1988, Kakalios has built a research program in experimental condensed matter physics, with particular emphasis on complex and disordered systems. His research ranges from the nano to the neuro with experimental investigations of the electronic and optical properties of nanostructured semiconductors and fluctuation phenomena in neurological systems.
During his time at the University of Minnesota, Kakalios has served as both director of undergraduate studies and director of graduate studies. He has received numerous awards and professorships including the University’s Taylor Distinguished Professorship, Andrew Gemant Award from the American Institute of Physics, and the Award for Public Engagement with Science from the American Association for the Advancement of Science (AAAS). He is a fellow of both the American Physical Society and AAAS.
In addition to numerous research publications, Kakalios is the author of three popular science books— The Physics of Superheroes , The Amazing Story of Quantum Mechanics , and The Physics of Everyday Things .
Kaklios received a bachelor’s degree from City College of New York and master’s and Ph.D. degrees from the University of Chicago.
Rhonda Zurn, College of Science and Engineering, [email protected]
University Public Relations, [email protected]
Read more stories:
Find more news and feature stories on the CSE news page .
Related news releases
- Faculty and grad student earn collegiate awards in 2024
- U of M Professor Vladimir Sverak elected to the Academy of Arts and Sciences
- Five CSE faculty members named Distinguished McKnight University Professors
- CSE Professor Hubert Lim named director of Bakken Medical Devices Center
- Assistant Professor Zhen Liu receives prestigious Sloan Research Fellowship for early-career researchers
- Future undergraduate students
- Future transfer students
- Future graduate students
- Future international students
- Diversity and Inclusion Opportunities
- Learn abroad
- Living Learning Communities
- Mentor programs
- Programs for women
- Student groups
- Visit, Apply & Next Steps
- Information for current students
- Departments and majors overview
- Departments
- Undergraduate majors
- Graduate programs
- Integrated Degree Programs
- Additional degree-granting programs
- Online learning
- Academic Advising overview
- Academic Advising FAQ
- Academic Advising Blog
- Appointments and drop-ins
- Academic support
- Commencement
- Four-year plans
- Honors advising
- Policies, procedures, and forms
- Career Services overview
- Resumes and cover letters
- Jobs and internships
- Interviews and job offers
- CSE Career Fair
- Major and career exploration
- Graduate school
- Collegiate Life overview
- Scholarships
- Diversity & Inclusivity Alliance
- Anderson Student Innovation Labs
- Information for alumni
- Get engaged with CSE
- Upcoming events
- CSE Alumni Society Board
- Alumni volunteer interest form
- Golden Medallion Society Reunion
- 50-Year Reunion
- Alumni honors and awards
- Outstanding Achievement
- Alumni Service
- Distinguished Leadership
- Honorary Doctorate Degrees
- Nobel Laureates
- Alumni resources
- Alumni career resources
- Alumni news outlets
- CSE branded clothing
- International alumni resources
- Inventing Tomorrow magazine
- Update your info
- CSE giving overview
- Why give to CSE?
- College priorities
- Give online now
- External relations
- Giving priorities
- CSE Dean's Club
- Donor stories
- Impact of giving
- Ways to give to CSE
- Matching gifts
- CSE directories
- Invest in your company and the future
- Recruit our students
- Connect with researchers
- K-12 initiatives
- Diversity initiatives
- Research news
- Give to CSE
- CSE priorities
- Corporate relations
- Information for faculty and staff
- Administrative offices overview
- Office of the Dean
- Academic affairs
- Finance and Operations
- Communications
- Human resources
- Undergraduate programs and student services
- CSE Committees
- CSE policies overview
- Academic policies
- Faculty hiring and tenure policies
- Finance policies and information
- Graduate education policies
- Human resources policies
- Research policies
- Research overview
- Research centers and facilities
- Research proposal submission process
- Research safety
- Award-winning CSE faculty
- National academies
- University awards
- Honorary professorships
- Collegiate awards
- Other CSE honors and awards
- Staff awards
- Performance Management Process
- Work. With Flexibility in CSE
- K-12 outreach overview
- Summer camps
- Outreach events
- Enrichment programs
- Field trips and tours
- CSE K-12 Virtual Classroom Resources
- Educator development
- Sponsor an event
Thank you for visiting nature.com. You are using a browser version with limited support for CSS. To obtain the best experience, we recommend you use a more up to date browser (or turn off compatibility mode in Internet Explorer). In the meantime, to ensure continued support, we are displaying the site without styles and JavaScript.
- View all journals
- Explore content
- About the journal
- Publish with us
- Sign up for alerts
- Open access
- Published: 26 June 2024
Storing CO 2 while strengthening concrete by carbonating its cement in suspension
- Xiaoxu Fu 1 ,
- Alexandre Guerini 2 ,
- Davide Zampini 3 &
- Alessandro F. Rotta Loria ORCID: orcid.org/0000-0001-6584-7526 1
Communications Materials volume 5 , Article number: 109 ( 2024 ) Cite this article
2849 Accesses
166 Altmetric
Metrics details
- Civil engineering
- Environmental impact
- Geochemistry
Cement is a key constituent of concrete and offers a large sequestration potential of carbon dioxide (CO 2 ). However, current concrete carbonation approaches are hindered by low CO 2 capture efficiency and high energy consumption, often resulting in weakened concrete. Here, we conceptually develop and experimentally explore a carbonation approach that resorts to injecting CO 2 into a cement suspension subsequently used to manufacture concrete, turning the carbonation reaction into an aqueous ionic reaction with a very fast kinetics compared to traditional diffusion-controlled approaches. This approach achieves a CO 2 sequestration efficiency of up to 45% and maintains an uncompromised concrete strength. The study shows that the CO 2 injection rate influences the polymorph selectivity of mineralized calcium carbonate (CaCO 3 ) depending on the local environmental conditions and impacts the strength of concrete. The technological simplicity of the proposed approach enables a reduced carbon footprint and promising prospects for industrial implementation.
Similar content being viewed by others

Carbon Capture and Utilization by mineralization of cement pastes derived from recycled concrete
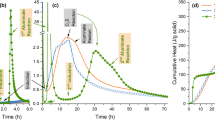
Unlocking the potential of ordinary Portland cement with hydration control additive enabling low-carbon building materials
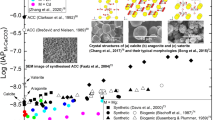
Maximising the benefits of calcium carbonate in sustainable cements: opportunities and challenges associated with alkaline waste carbonation
Introduction.
According to the Intergovernmental Panel on Climate Change, global greenhouse gas emissions continue to rise and have reached a net anthropogenic value of 59 ± 6.6 Gt CO 2 -eq in 2019 1 . The energy sector accounts for over 60% of such emissions, leaving nearly 40% of global emissions to other industry sectors 2 , 3 . The cement and concrete industries play a significant role in this context, with ordinary Portland cement production alone being the largest emitter and the source of ~8% of the global anthropogenic CO 2 emissions 4 . Meanwhile, ordinary Portland cement has a high potential for CO 2 sequestration owing to its inherent alkalinity, promising to turn the cement and concrete industries into carbon sinks via the conversion of CO 2 into solid crystals mainly consisting of calcium carbonate (CaCO 3 ). Stable carbonate crystals have a longer lifetime than structures made of cement and concrete, thus representing an optimal means to store CO 2 in such structures 5 , 6 .
Over the past decades, various efforts have focused on the reduction of the CO 2 emissions deriving from the cement and concrete industries by working on the whole life cycle of these materials, from the extraction of the involved raw materials to their processing 7 , 8 , manufacturing 9 , 10 , use 11 , 12 , and end of life 13 , 14 , 15 , 16 . In this context, two main categories of approaches have been developed to store CO 2 in the cementitious materials that constitute concrete during the manufacturing of such a mixture: hardened and fresh concrete carbonation approaches. Hardened concrete carbonation approaches were introduced in the 1970s 17 , 18 and gained limited attention until recently but remain confined to laboratory studies due to technological limitations. Fresh concrete carbonation approaches were introduced more recently 19 , 20 , 21 but already benefit from commercial pilots due to technological advantages 19 , 22 .
Hardened carbonation approaches involve concrete preparation and mixing, concrete casting, concrete exposure to CO 2 gas, and concrete hydration 23 , 24 , 25 . The pressure, temperature, duration, and general environmental conditions at which CO 2 is placed in contact with hardened concrete distinguish the different types of hardened concrete carbonation approaches. Besides these differences, all hardened concrete carbonation approaches involve chemical reactions that engage the active cementitious constituents of hardened concrete with the conversion of CO 2 gas into solid CaCO 3 crystal forms. Nevertheless, as these approaches are driven by diffusion-controlled kinetics, they are limited by the minimal extent of CO 2 diffusion in hardened concrete, which enables carbonation reactions from the surface of hardened concrete to a depth of a few millimeters 26 . Pressurized vessels, usually between 1 and 5 atm 27 , can improve the carbonation degree and penetration depth 24 , 28 , 29 . However, the resulting cost-effectiveness diminishes as the applied pressure increases 30 , with CO 2 uptake rates that remain within 5–20% 24 at the expense of significant energy and infrastructure needs. Furthermore, the nature of these approaches limits their applicability to precast concrete, as they require CO 2 -rich or high-pressure environments 31 .
Fresh concrete carbonation approaches involve concrete preparation and mixing with the simultaneous injection of CO 2 gas, concrete casting, and concrete hydration 20 , 32 , 33 . With these approaches, chemical reactions engage fresh concrete with the conversion of CO 2 gas into solid CaCO 3 crystal forms. Therefore, two main advantages characterize fresh compared to hardened carbonation approaches: (1) the possibility to carbonate a greater volume of concrete via CO 2 injections during mixing; (2) the possibility to incorporate relatively easily CO 2 injection nozzles in industrial processes.
Considering the advantages of fresh compared to hardened concrete carbonation approaches, increasing experimental investigations have been performed in recent years to maximize their performance 19 , 20 , 21 , 32 , 33 , 34 , 35 , 36 . However, the understanding of the reactions, kinetics, and mechanisms involved in fresh concrete carbonation approaches remains elusive, leading to limited CO 2 capture efficiencies 20 . This evidence, together with the fact that energy consumption induced by CO 2 pumping, purification, transport, and high-pressure vessels can involve greater emissions of CO 2 gas compared to those that are sequestered 5 , involves that the carbon capture potential of fresh concrete carbonation approaches remains largely untapped.
Motivated by the opportunity to improve the fundamental understanding and efficacy of fresh concrete carbonation approaches, this work presents an experimental laboratory study in support of an approach to carbonate fresh concrete that harnesses a key methodological difference compared to the state-of-the-art. The difference between the proposed approach and state-of-the-art approaches is that CO 2 gas is injected into a diluted cement suspension instead of fresh concrete, which completely changes the carbonation reaction kinetics. Specifically, the proposed approach resorts to a multi-stage concrete preparation method whereby CO 2 gas is first injected into a cement suspension, the resulting material is then used to achieve a cement paste of desired properties, and concrete is finally obtained by adding and mixing aggregates. The results of laboratory experiments (see the “Methods” section) indicate that injecting CO 2 gas into a cement suspension, rather than the conventional method of injecting CO 2 into fresh concrete, significantly accelerates constituent reactions and CaCO 3 precipitation kinetics. Therefore, under specific treatment conditions that are precisely identified via the developed experiments, not only the properties of the carbonated concrete can remain unchanged, but the carbon footprint associated with the production of such material can be reduced compared to current standards. This discovery supports more efficient concrete carbonation approaches and advances the understanding of mineralization processes, which are widespread in materials science and engineering.
Figure 1 presents scanning electron microscopy (SEM) images to illustrate the impacts of CO 2 gas injections with different volumes (2000, 4000, and 8000 standard cubic centimeters, i.e., scc; corresponding to 3.9, 7.9, and 15.7 g of CO 2 ) on the morphology of cement particles constituting the concrete mix design selected for this work (see the “Methods” section for details). The results illustrate the features of cement particles deriving from (a) a cement suspension that did not undergo any CO 2 injection (i.e., an uncarbonated cement suspension); (b–d) a cement suspension that underwent a CO 2 injection (i.e., a carbonated cement suspension); (e) a cement suspension that did not undergo any CO 2 injection and was subsequently mixed with additional cement (i.e., an uncarbonated cement paste); and (f–h) a cement suspension that underwent the injection of CO 2 and was subsequently mixed with additional cement (i.e., a carbonated cement paste).
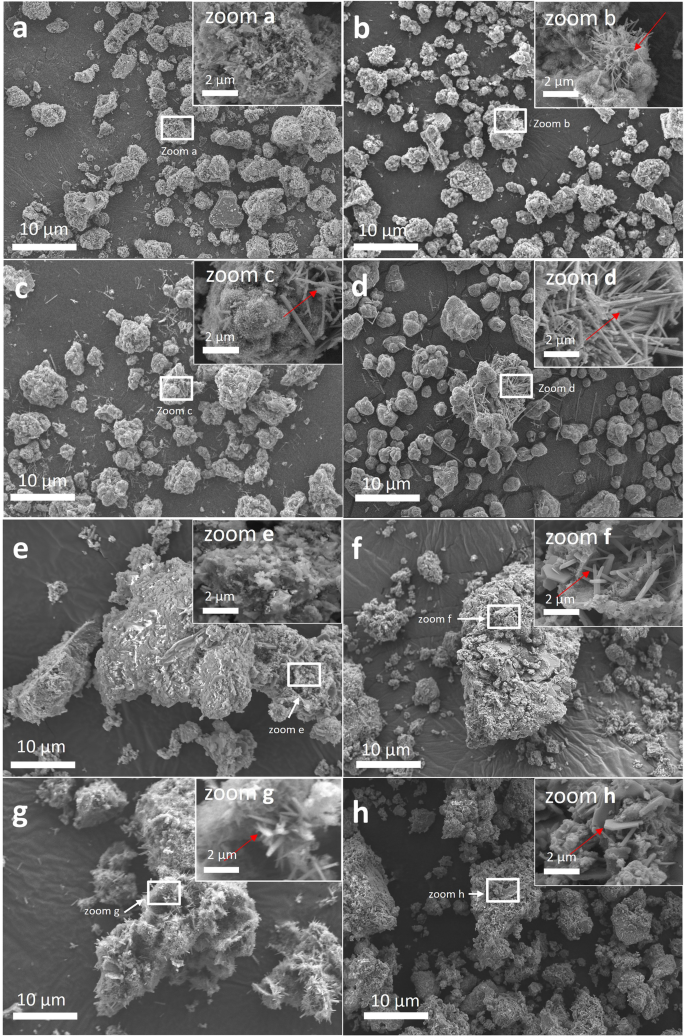
The cement suspension is made of 50 g of cement and 100 mL of deionized water (DI-H 2 O), involving a water-to-cement ratio of w/c = 2. The concrete is prepared from the suspension by first adding and mixing 50 g of virgin cement, achieving a cement paste with w/c = 1, and then adding another 100 g virgin cement (w/c = 0.5) and mixing of aggregates. See the “Methods” section for details. a Uncarbonated suspension. b Carbonated suspension with 2000 scc of CO 2 . c Carbonated suspension with 4000 scc of CO 2 . d Carbonated suspension with 8000 scc of CO 2 . e Uncarbonated paste. f Paste deriving from a carbonated suspension with 2000 scc of CO 2 . g Paste deriving from a carbonated suspension with 4000 scc of CO 2 . h Paste deriving from a carbonated suspension with 8000 scc of CO 2 . Red arrows: crystal structures.
By comparing Fig. 1a with Fig. 1b–d , it is possible to assess the influence of CO 2 injections on the morphology of the particles that constitute a cement suspension. By comparing Fig. 1e with Fig. 1f–h , it is possible to assess the influence of CO 2 injections on the morphology of the particles that constitute a cement paste deriving from a carbonated cement suspension. By comparing Fig. 1a–d with Fig. 1e–h , it is possible to assess the influence of adding cement to a given suspension, which may or may not be carbonated with CO 2 gas, to achieve a cement paste for use in concrete.
The uncarbonated cement suspension (Fig. 1a ) and paste (Fig. 1e ) show the typical morphology of cement hydration products, with irregular shapes, rough surfaces, and no visible crystals. The injection of CO 2 into a cement suspension and the addition of cement to such suspension influence the morphology and size of cement particles, respectively. As a result of the carbonation, crystals deposited on the surface of most cement particles become visible (Fig. 1b–d and f–h , respectively). Such crystals are uniformly found on the surface of cement particles derived from a cement suspension (Fig. 1b–d ), whereas they appear both on the surface and interlocked within the bulk of cement particles deriving from a cement suspension mixed with additional cement after carbonation (Fig. 1f–h ). Larger amounts of injected CO 2 gas yield the formation of a larger number of crystals (Fig. 1b–d and f–h ). The crystals found on cement particles that derive from cement suspensions always resemble needles for increasing volumes of injected CO 2 (i.e., Fig. 1b–d ). In contrast, the crystals found on cement particles that derive from cement pastes resulting from carbonated cement suspensions resemble needles for relatively limited volumes of injected CO 2 (i.e., for 2000 and 4000 scc, Fig. 1f, g ) but become flakes for larger volumes of CO 2 (i.e., for 8000 scc, Fig. 1h ). Larger and more polydisperse cement particles are obtained when cement is added to a suspension after its preparation, irrespective of whether such suspension does not undergo carbonation (Fig. 1a vs. Fig. 1 e ) or does undergo carbonation (Fig. 1b–d vs. Fig. 1f–h ). Cement particles that derive from carbonated cement suspensions have a size <10 µm (Fig. 1b–d ), whereas cement particles that derive from virtually equivalent carbonated cement suspensions and subsequently mixed with more cement have a size that can exceed 20 µm (Fig. 1f–h ). Energy dispersive spectroscopy (EDS) analyses (Supplementary Fig. 1 ) show that the observed crystals have the major elemental compositions of O (45.1–45.6%), Ca (33.0–34.3%), C (13.1–13.8%), Si (3.0–3.5%), and Fe (1.5–1.7%). Other detected elements including S, Al, and K have elemental weights <1%.
Figure 2 illustrates the results of (a) thermogravimetric analyses (TGA), (b) differential thermal analyses (DTA), and (c) X-ray diffraction (XRD) spectra obtained for both carbonated and uncarbonated cement suspensions. The TGA curves reveal two distinct temperature ranges characterized by significant weight losses (Fig. 2a ). The first range is approximately located between 380 and 440 °C, whereas the second is between 520 and 740 °C. The first range corresponds to a dehydration range associated with the loss of absorbed water in the range of 105 to 400 °C and the CH dehydration in the range of 400–500 °C 37 . The second range corresponds to the decarbonation process typically taking place between 530 and 900 °C 38 . The peaks in the DTA curves at the corresponding temperatures (Fig. 2b ) corroborate these results. Uncarbonated cement suspensions exhibit the highest dehydration peak and the lowest decarbonation peak. As the amount of injected CO 2 increases, the dehydration peak decreases while the decarbonation peak intensifies, with no visible dehydration peak being observed for 8000 scc of injected CO 2 . The decarbonation peaks shift to higher temperatures with an increasing amount of injected CO 2 . Meanwhile, the decarbonation peak temperatures for uncarbonated cement and carbonated cement with 2000, 4000, and 8000 scc of CO 2 are 656, 668, 678, and 702 °C, respectively. The XRD spectra (Fig. 2c ) substantiate the results discussed so far by indicating the presence of calcium carbonate in the form of calcite and aragonite in the carbonated cement, along with the typical constituents of cement represented by C3S, C2S, ettringite, C–S–H, and portlandite; additionally, these results enable to remark the lack of visible peaks of calcite and aragonite in the uncarbonated cement. Therefore, the observed crystals on the surfaces of carbonated cement particles (Fig. 1 ) are CaCO 3 and not cement hydration products. As the amount of injected CO 2 increases, the relative intensity of most of the peaks also increases, indicating an increase in the overall crystallization degree.
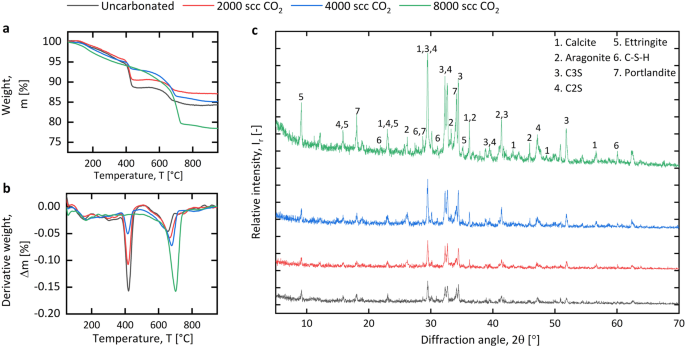
a TGA curves of cement suspensions. b DTA curves of cement suspensions. c XRD spectra of carbonated and uncarbonated cement suspensions.
Figure 3 illustrates key variables related to the efficacy of the proposed approach: (a) pH and temperature variations of the suspensions during their preparation and carbonation; carbon capture performance expressed as (b) CO 2 capture efficiency, (c) CO 2 capture mass, and (d) carbonation degree of the resulting concrete as a function of the CO 2 treatment duration; and influences on (e) absolute unconfined compressive strength of uncarbonated and carbonated concrete and (f) normalized strength of carbonated relative to uncarbonated concrete.

a Suspension pH ( n = 3 independent experiments) and temperature at different stages of the preparation process (raw data reported in Supplementary Tables 1 and 2 ). b CO 2 capture efficiency with different CO 2 injection durations ( n = 3 independent experiments; raw data reported in Supplementary Table 3 ). c Carbonation degree of carbonated cement with different CO 2 injection durations ( n = 3 independent experiments; raw data reported in Supplementary Table 4 ). d CO 2 capture mass per unit volume of concrete with different CO 2 injection durations ( n = 3 independent experiments; raw data reported in Supplementary Table 5 ). e Unconfined compressive strength of the concrete specimens as a function of the curing time and the volume of injected CO 2 ( n = 10 independent experiments; raw data reported in Supplementary Table 6 ). f Normalized strength relative to the uncarbonated material (raw data reported in Supplementary Table 7 ). Error bars show standard deviations.
The preparation of the cement suspension starts with the use of DI-H 2 O only, which has a pH of 7 at an ambient room temperature of 21.2 °C (Fig. 3a ). Next, DI-H 2 O is saturated with CO 2 gas, yielding a pH of ~5.3 without any significant temperature change. Afterwards, cement is added to the CO 2 -saturated DI-H 2 O (with no concurrent CO 2 injection), leading to an increase in pH up to the value of 12.60 ± 0.05 and a surge in the solution temperature from 21.2 to 23.1 °C under stabilized conditions. Finally, CO 2 is injected in the cement suspension for a time of up to 40 min, leading to an instantaneous decrease in pH from 12.60 ± 0.05 to 12.18 ± 0.08 during the considered time, which increases up to the value of around 12.6 under stable conditions (see the “Methods” section). After 40 min of carbonation, the stable and instant pH values are 12.18 and 12.63, corresponding to OH − concentrations of 0.015 and 0.043 M, respectively. Temperature drastically increases at the beginning of the CO 2 injection, but such an increase becomes less pronounced as carbonation progresses, stabilizing around 39.5 °C after 30 min.
The CO 2 capture efficiency decreases for longer injection durations, from 45.4% for a 10-min injection (2000 scc) to 26.2% for a 40-min injection (8000 scc) (Fig. 3b ). Despite this decrease, the carbonation degree increases with the amount of injected CO 2 (Fig. 3c ), starting from 3.3% when cement suspensions are uncarbonated and placed in contact with CO 2 from ambient air and achieving 17.2% for a CO 2 injection lasting 40 min. The absolute mass of captured CO 2 also increases with a higher amount of injected CO 2 (Fig. 3d ). Specifically, 5.2, 7.7, and 12.0 kg of CO 2 per unit volume of concrete are captured during 10, 20, and 40 min of injection, respectively.
Figure 3e, f shows the unconfined compressive strength of concrete specimens obtained from uncarbonated and carbonated cement suspensions after 3, 7, 28, and 56 days of curing. The strength shows a typical increase with the curing time due to ageing, irrespective of whether the concrete derives from cement suspensions that underwent carbonation or not (Fig. 3e ). Meanwhile, reference to the average strength of the prepared concrete specimens allows us to identify several noteworthy trends. Although the average strength of carbonated concrete is always higher than the strength of uncarbonated concrete at early stages of curing (i.e., at 3 days), such strength can be higher or lower than the average strength of uncarbonated concrete at successive stages of curing (i.e., at 7, 28, and 56 days) depending on the amount of injected CO 2 (Fig. 3f ). Concrete deriving from cement suspensions free of carbonation displays average strengths of 22.9 ± 4.0, 31.0 ± 2.5, 35.4 ± 2.7, and 36.5 ± 4.8 MPa after 3, 7, 28, and 56 days, respectively. After 3 days of curing, the average strength of concrete deriving from cement suspensions that underwent carbonation is at least 10% higher than the average strength of uncarbonated concrete. After 7, 28, and 56 days, the average strength of concrete deriving from cement suspensions that underwent carbonation is generally smaller (down to 20.7%) than the average strength of uncarbonated concrete for 2000 scc and 4000 scc of injected CO 2 . In contrast, after 7, 28, and 56 days, the average strength of carbonated concrete is generally higher (up to 23.1%) than the strength of uncarbonated concrete for 8000 scc of injected CO 2 . Besides these considerations, an analysis of the standard deviation of these latter data suggests that the actual average strength of carbonated concrete may not be statistically higher than that of uncarbonated concrete. Meanwhile, it does indicate that the strength of carbonated concrete is at least not compromised compared to the strength of uncarbonated concrete for 8000 scc of injected CO 2 .
CaCO 3 precipitation and its impact on concrete strength
The strength of concrete strongly depends on the constituents and the chemical reactions characterizing such material. The proposed carbonation approach induces changes in the constituents and reactions that characterize concrete, which influence the structure and properties of such material.
In general, CaCO 3 precipitates in three main polymorphs: calcite, aragonite, and vaterite. Calcite is the stable polymorph and exhibits thermostability 39 , 40 . Aragonite is generally less stable than calcite and transitions to such polymorph at a relatively slow rate under ambient conditions 39 . Vaterite is unstable and hence uncommon in natural environments, transforming into aragonite and calcite at temperatures ranging from 0 to 30 °C and 60 to 80 °C, respectively 39 , 40 . CaCO 3 is recognized as calcite when it decomposes in TGA within the range 650–900 °C, whereas is considered as aragonite when it decomposes within the range 530–650 °C 38 . Meanwhile, weight losses in TGA between 500 and 700 °C are attributed to poorly crystalline CaCO 3 , whereas weight losses between 700 and 900 °C are attributed to highly crystalline CaCO 3 37 , 41 .
The results of this work indicate that carbonated cement suspensions subsequently used to manufacture concrete undergo the precipitation of CaCO 3 crystals that vary in quantity and morphology (Fig. 1 ). The quantity of CaCO 3 precipitations essentially depends on the amount of injected CO 2 and increases for larger volumes of injected CO 2 . The morphology of CaCO 3 precipitations depends on the crystal polymorph type and the crystallization degree (i.e., the ratio of crystalline to amorphous precipitated minerals). As TGA results support that decarbonation occurs between 530 and 900 °C (Fig. 2a ), the achieved CaCO 3 precipitations are a mixture of calcite and aragonite, as also substantiated by the XRD results (Fig. 2c ). The results also indicate a shift toward higher temperatures of the decomposition and decarbonation peaks for different CO 2 treatments (Fig. 2b ). Thus, changes in the polymorph type and crystallization degree take place.
The formation of a mixture of different CaCO 3 polymorphs can be attributed to two formation pathways of such compounds due to the hydration and carbonation of concrete. During the hydration and carbonation of concrete, which occur nearly simultaneously (although carbonation is faster than hydration 23 ), two main compounds contribute to the precipitation of CaCO 3 : calcium silicate hydrate (C–S–H) and calcium hydroxide (Ca(OH) 2 ). Another two compounds, represented by calcium aluminate (Ca 3 Al 2 O 6 ) and calcium aluminoferrite (Ca 4 Al 2 Fe 2 O 10 ), can also form as a result of CO 2 injections but typically in a significantly smaller fraction than calcium silicates. The reason is that calcium aluminate has a fast hydration reaction rate but remains rather inactive with CO 2 42 , and calcium aluminoferrite also shows limited reactivity with CO 2 43 . Since C 3 S and C 2 S are practically insoluble in water, the direct carbonation of them is rather slow.
The hydration reaction of calcium silicates (tricalcium silicate, C 3 S; dicalcium silicate, C 2 S) yields C–S–H and Ca(OH) 2 as follows:
In turn, Ca(OH) 2 reacts with CO 2 and forms CaCO 3 as follows:
In addition, C–S–H can also react with CO 2 and form CaCO 3 as follows:
CaCO 3 crystals formed via these two pathways yield different polymorphs: CaCO 3 formed from Ca(OH) 2 tends to be calcite, whereas CaCO 3 formed from C–S–H tends to be aragonite 38 , 44 . Available carbonation studies either take for granted that CaCO 3 precipitations are calcite or do not discuss the polymorph type. However, an analysis of these studies 20 suggests that CaCO 3 precipitations consist of a mixture of both calcite and aragonite even in these cases.
The changes in the polymorph type and crystallization degree can be attributed to temperature variations because these variables are strongly sensitive to temperature 40 . Following the formation of a calcite–aragonite mixture through pathways ( 3 ) and ( 4 )–( 6 ), a transition of aragonite to calcite characterizes any cement-based material exposed to CO 2 (e.g., naturally present in the air or artificially injected). In the proposed carbonation approach, the injection of CO 2 into a suspension involves intense exothermic reactions that enhance the temperature rise that would naturally occur due to cement hydration (Fig. 3 ). This enhanced temperature rise has two main effects. First, it accelerates the transition of aragonite into calcite, with a larger proportion of aragonite turned into calcite for a larger amount of injected CO 2 (at a given flow rate) because of more significant temperature variations (Fig. 3 ). Second, it increases the crystallization degree of precipitated CaCO 3 crystals. Both outcomes can be inferred from the shift of the decarbonation peak towards higher temperatures for a larger amount of injected CO 2 (Fig. 2b ).
CaCO 3 precipitations in freshly cast concrete are generally recognized to enhance the early-stage strength of such material through so-called accelerated curing 21 , 45 . This result partly derives from the formation of dense calcium carbonate layers in some of the material pores 46 and partly from the significant degree of cement reaction, either through carbonation or hydration, which is much higher than that achieved through typical steam curing regimes. The extra nucleation seeds provided by CaCO 3 precipitations are the main factor of such acceleration 21 . Meanwhile, having more cement constituents reacted, whether through carbonation or hydration, is also beneficial for early-stage strength development 47 . This evidence has been observed via fresh concrete carbonation 20 , 32 , 48 and hardened concrete carbonation 44 , 49 , 50 .
In contrast, CaCO 3 precipitations and/or crystal transitions at later stages are typically detrimental to the strength of concrete. This evidence results from the fact that aragonite typically has a higher density (2.94 g/cm 3 ) than calcite (2.71 g/cm 3 ) 51 , which implies that polymorph transitions inevitably induce changes in the volume and shape of these crystals in the concrete structure, leading to deleterious cracking 44 , 52 , 53 . For this reason, carbonation approaches are considered successful when they do not affect the strength of concrete due to the addition of CaCO 3 crystals. Accordingly, typical carbonation approaches show, at best, unchanged values of strength, especially after 28 days 23 .
By injecting CO 2 into a cement suspension during mixing, the carbonation approach proposed in this work provides three key opportunities:
It employs less energy compared to that required to carbonate fresh and hardened concrete, as CO 2 gas is injected into a liquid that has a viscosity comparable to water (i.e., significantly inferior to fresh concrete).
It allows harnessing the large surface area of cement particles floating in the water and engaging only a portion of fresh cement with reactions with CO 2 , as cement is added to a suspension after its carbonation to achieve a paste for use in concrete. While achieving CaCO 3 precipitations around some cement particles, this approach avoids the enclosure of most cement particles. This ability is favorable because cement particles encapsulated by CaCO 3 crystals cannot yield the formation of C–S–H and involve an aggregation of CaCO 3 -coated particles into clusters with interconnected and unwanted pores, ultimately leading to deteriorations in the strength of concrete 20 .
It fosters temperature variations during the CO 2 treatment of a cement suspension, resulting in the precipitation of more stable and crystalline CaCO 3 for larger temperature variations associated with larger amounts of injected CO 2 (at a given flow rate). This ability is favorable because stable CaCO 3 crystals remain unaffected by polymorph transitions during the subsequent concrete preparation with additional cement and aggregates, curing, and lifetime.
The obtained results specifically allow us to postulate that the variable strength achieved for variable amounts of injected CO 2 is due to a variable conversion of CO 2 into more stable and crystalline forms of CaCO 3 . In particular, when 2000 and 4000 scc of CO 2 are injected in a cement suspension, the proportion of CO 2 that is turned into stable and crystalline forms of CaCO 3 is limited and subsequently results in unwanted polymorph transitions and volume changes. In contrast, when 8000 scc of CO 2 is injected in a cement suspension, the proportion of CO 2 that is turned into stable and crystalline forms of CaCO 3 is more significant and subsequently involves minimal polymorph transitions. As a result, the strength of concrete remains uncompromised through the presence of durable and strong calcite deriving from the conversion of CO 2 into solid crystals (Fig. 1 ).
In order to substantiate the proposed mechanism behind the performance of this carbonation approach, experiments involving the injection of the same amount of CO 2 (i.e. 8000 scc) with different flow rates over different timeframes (i.e., the reference flow rate of 200 sccm over 40 min and the two additional flow rates of 100 and 400 sccm during 80 and 10 min, respectively) were designed and conducted (see the “Methods” section). The results are shown in Fig. 4 . The injection of higher CO 2 flow rates in cement suspensions results in faster temperature increases in such suspensions per unit time (Fig. 4a ). Maximum temperatures of 16.5, 20.4, and 22.2 °C are measured for flow rates of 100, 200, and 400 sccm after 70, 52, and 45 min, respectively. However, at the time the same volume of 8000 scc of CO 2 is injected in cement suspensions, temperatures of 16.2, 19.1, and 13.8 °C are recorded for 100, 200, and 400 sccm, respectively. Therefore, the highest temperature is achieved for the reference flow rate of 200 sccm. All carbonated specimens with a volume of 8000 scc of CO 2 exhibit a higher strength compared to the uncarbonated concrete, regardless of the applied flow rates or the curing time (Fig. 4b ). This evidence supports that there is a threshold value of injected CO 2 and interconnected amount of CaCO 3 crystals in concrete that overcomes the negative influence of possible polymorph transitions and yields a strength increase as opposed to a strength decrease compared to uncarbonated concrete. Meanwhile, the greatest strength characterizes the concrete deriving from a cement suspension carbonated with a flow rate of 200 sccm compared to 100 or 400 sccm at any curing time, supporting that there is a threshold temperature value for which CaCO 3 crystals mostly transition to more stable calcite instead of remaining aragonite compounds, or for which an increase in the crystallization degree is triggered. Accordingly, the concrete deriving from a cement suspension carbonated with a flow rate of 200 sccm is the only one that presents both CaCO 3 needles and flakes on its constituting cement particles, whereas the concrete deriving from a cement suspension carbonated with a flow rate of 100 and 400 sccm only presents CaCO 3 needles (Fig. 4c–h ).
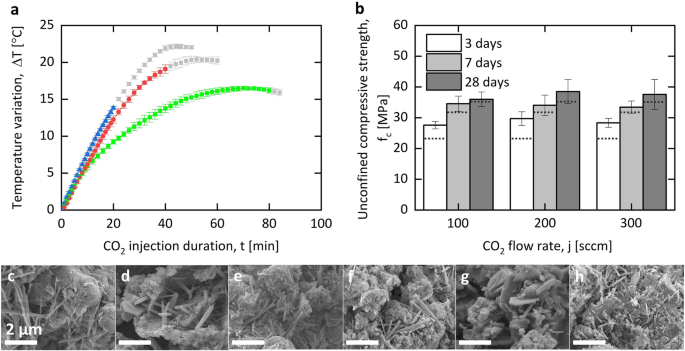
a Trends of temperature variations in the carbonated cement suspension for different CO 2 flow rates ( n = 3 independent experiments; raw data reported in Supplementary Table 8 ; error bars show standard deviations). b Compressive strength of concrete deriving from a paste placed in contact with different CO 2 flow rates relative to the reference uncarbonated concrete (reference values are dashed; n = 5 independent experiments; raw data reported in Supplementary Table 9 ; error bars show standard deviations); and SEM images of the CaCO 3 crystals formed on the cement particles deriving from a suspension subjected to c 100 sccm, d 200 sccm, and e 400 sccm, or a paste resulting from a suspension subjected to f 100 sccm, g 200 sccm, h 400 sccm.
Figure 5 summarizes the key stages involved in the strength development of concrete resulting from the proposed carbonation approach. The proposed approach allows to engineer the sequence of hydration and carbonation reactions and tailor them to specific quantities of cement.
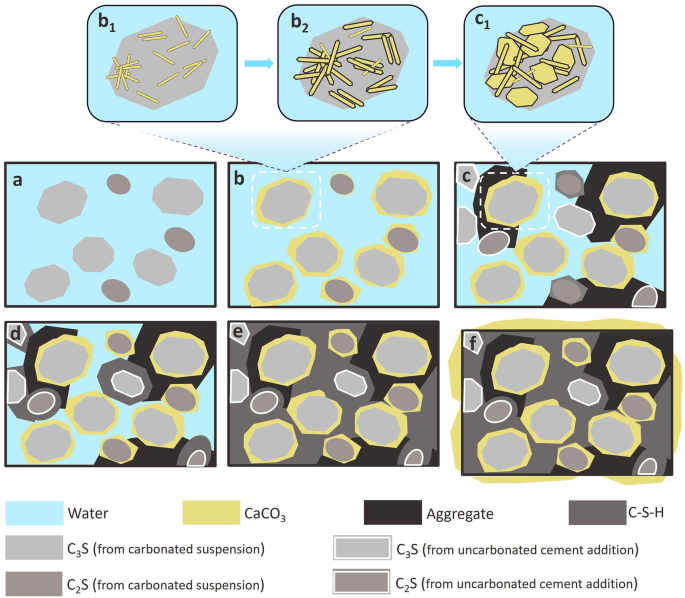
a Injection of CO 2 into a cement suspension. b Growth of CaCO 3 crystals in such a suspension (inlets b 1 and b 2 show the growth and transition of CaCO 3 crystals in the cement suspension). c Addition of cement and aggregates to suspension (inlet c 1 shows the transition of CaCO 3 crystals in the cement paste). d Dominant hydration of cement in concrete. e Development of interparticle bonding within concrete. f Optional carbonation of hardened concrete.
The proposed approach starts with the mixing of a small portion of cement powder with water while CO 2 is injected in the system (Fig. 5a ). Carbonation and hydration reactions commence concurrently, but carbonation is faster than hydration at this stage.
As CO 2 injection continues, CaCO 3 precipitates in the cement suspension while temperature rises (Fig. 5b ). Depending on the local environmental conditions, CaCO 3 crystals undergo polymorph transitions and changes in polymerization degree, covering a wider number and surface of C 3 S/C 2 S particles as time progresses (Fig. 5b1, b2 ). The precipitation of CaCO 3 in a suspension involves that most polymorph transitions and volume changes of such crystals occur early, limiting the potential for cracking and deleterious effects on the structure, properties, and behavior of concrete during its curing and lifetime.
Following the CO 2 injection, the second portion of cement powder and aggregates are introduced (Fig. 5c ), previously precipitated CaCO 3 crystals can be characterized by morphology changes, and new CaCO 3 crystals can be formed because of the contact with atmospheric CO 2 (Fig. 5c1 ). At this stage, mixing is performed as in any classical approach to manufacturing concrete. Thereafter, the CaCO 3 crystals can act as nucleation seeds for subsequent hydration of C 3 S and C 2 S, accelerating the hydration process (Fig. 5d ) and contributing to the enhanced early-age strength of concrete.
As the curing process continues, the degree of hydration increases and C–S–H fills the concrete pores as in typical concrete curing (Fig. 5e ). This process is crucial to achieving interparticle bonding within the concrete matrix, ensuring strength development.
Finally, the concrete can optionally undergo an additional step of carbonation in its hardened state (Fig. 5f ), as in classical carbonation approaches of hardened concrete. In this context, carbonation can still characterize some quantity of cement and form a carbonated layer on the external surface of the concrete.
Carbon capture capacity, kinetics, and efficiency
Carbonation approaches should not only be evaluated in terms of their impacts on the structure and properties of carbonated materials, but also in terms of their ability to effectively store CO 2 in durable, solid crystal forms as a result of their CO 2 capture capacity, kinetics, and efficiency. The carbon capture capacity reflects the theoretical number of alkaline compounds that can react with CO 2 and form stable carbonates, representing the theoretical CO 2 sequestration potential of any carbonation approach. The CO 2 reaction kinetics determines the amount of CO 2 that can be captured for a given CO 2 injection duration. The CO 2 capture efficiency results from variable CO 2 sequestration capacities and reaction kinetics.
The main reactive alkaline compound in cementitious materials is Ca(OH) 2 , which comes from the hydration of C 3 S and C 2 S (Eqs. ( 1 ) and ( 2 )). This compound typically gives such materials a pH value between 12 and 13. In some instances, the pH can nonetheless exceed a value of 13 due to the presence of KOH or NaOH 54 . In this work, the pH of a well-stabilized cement suspension is 12.60 ± 0.05 (Fig. 3a ). This value closely aligns with the value of 12.33 that characterizes the pH of a saturated Ca(OH) 2 solution:
This result suggests that Ca(OH) 2 is the primary source of OH - in the considered suspension system. According to Eq. ( 3 ), Ca(OH) 2 reacts with CO 2 and forms insoluble CaCO 3 . However, according to Eq. ( 4 )–( 6 ), when Ca(OH) 2 is formed through Eqs. ( 1 ) and ( 2 ), C–S–H is concurrently formed in these reactions and can also react with CO 2 in conjunction with Ca(OH) 2 . Therefore, through a combination of Eqs. ( 1 )–( 6 ), the CO 2 -active component in cement is equivalent to CaO from a stoichiometric standpoint, allowing for the simplification of the previous equations to the following:
Thus, the carbon capture capacity can be calculated based on the CaO content in the materials.
To determine a more universal carbon capture capacity value for the proposed approach, a typical Type I cement composition is used as reference (Table 2 ). CaO constitutes 61.23% of the cement, resulting in ~30.6 g of CaO in the experimental setup employing 50 g of cement. In principle, according to Eq. ( 8 ), ~12,200 scc (24 g) of CO 2 can be captured and precipitated as CaCO 3 in the considered cement suspension if every molecule of CaO were to react with CO 2 . In practice, 2992 scc of CO 2 are captured when 8000 scc of CO 2 are injected into the cement suspension (Fig. 3 ). Therefore, the achieved CO 2 sequestration capacity is markedly smaller than the theoretical sequestration capacity of the carbonated cement suspension due to two reasons: (1) as CO 2 is injected, the surfaces of cement particles progressively become partly or entirely covered by CaCO 3 crystals (Fig. 1 ), and hence less reactive to CO 2 ; (2) as CO 2 is injected for a limited time, not all the CO 2 can react with the available cement particles.
The obtained results also indicate a decrease in the CO 2 reaction rate, which is attributed to a decrease in the OH - concentration in the material and a corresponding decrease in pH. An analysis of the reaction kinetics corroborates this evidence. Since a series of chemical and physical reactions develop during the proposed carbonation process, it is essential to know which one is the rate-control step. CaCO 3 precipitation relies on the concentrations of the two reactants, Ca 2+ and CO 3 2− , as indicated by the following equation:
The presence and concentration Ca 2+ and CO 3 2− in the aqueous suspension rely on their reaction routes.
On the one hand, the presence of CO 3 2− comes from CO 2 dissolution in an aqueous solution, which follows a series of chemical equilibria. First, CO 2 transitions from a gaseous to an aqueous environment:
As CO 2 comes in contact with H 2 O, the formation and ionization of carbonic acid take place, which supply H + and CO 3 2− according to the following:
As CO 2 injection occurs, the concentration of CO 2 (g) can be considered infinite (Eq. ( 11 )). The consumption rate of H + plays a crucial role in shifting the chemical equilibrium to the right and increasing the concentration of CO 3 2− (Eqs. ( 11 )–( 13 )). The following acid-base neutralization reaction is very fast:
Therefore, the dissolution of Ca(OH) 2 is the rate-determining step, according to the following:
As Eq. ( 15 ) is the main reaction that generates OH − in the system, the pH turns out to be the indicator of the overall reaction rate. During CO 2 injection, the pH decreases from 12.60 to 12.18, leading to a decrease in OH − concentration from 0.040 to 0.016 M and, hence, a concentration change of 0.024 M (Fig. 3 ). This OH − concentration change is larger than half of the initial OH − concentration. This decrease in OH − concentration affects the rate of CO 2 dissolution, which provides CO 3 2− . Notably, the temperature increase during the CO 2 injection also impacts the Ca(OH) 2 solubility (for Ca(OH) 2 , K sp (22 °C) ≈ 7.95 × 10 −5 ; K sp (40 °C) ≈ 5.4 × 10 −5 ). The OH − concentration under saturated conditions decreases from 0.043 to 0.038 M under such temperature increase and involves a concentration change of 0.005 M. However, the observed OH − concentration decrease is significantly larger than the OH − concentration drop caused by temperature. Therefore, the primary limitation to OH − concentration maintenance still comes from Ca(OH) 2 dissolution.
On the other hand, Ca 2+ comes directly from dissolution of Ca(OH) 2 . The reactions involved are as follows:
Equation ( 16 ) indicates the formation of Ca(OH) 2 . Equation ( 17 ) indicates the dissolution of Ca(OH) 2 . Equation ( 18 ) indicates the diffusion of ions from the particle surface to the bulk solution. Under unagitated conditions, the rate-controlling step is the diffusion step expressed in Eq. ( 18 ) 55 . However, in a vigorously agitated system like the one that characterizes the proposed approach to inject CO 2 into a cement suspension while it is mixed, diffusion is accelerated, and the rate-controlling step is the dissolution of Ca(OH) 2 at the surface instead 56 , as expressed in Eq. ( 17 ). Therefore, the decreased CO 2 capture rate is caused by a decrease in the OH - concentration in the material and a corresponding decrease in pH.
Most fresh concrete carbonation approaches have limited CO 2 capture efficiency of up to 10% 20 . The proposed carbonation approach achieves a CO 2 capture efficiency that surpasses 45% when injecting 2000 scc of CO 2 into the system and remains high at 26.2% when injecting a volume of 8000 scc CO 2 (Fig. 3 ). In this context, the following aspects are noteworthy: (1) the developed experiments are performed by using an uncapped beaker container, which does not prevent the dispersion of CO 2 into the atmosphere; (2) the experiments do not incorporate any looping system, which could further allow to enhance the treatment efficacy by lengthening the retention time of CO 2 in the suspension; (3) any upscaled application of such an approach would lengthen the retention time, enhancing CO 2 capture efficiency. Therefore, significant margins for improvement characterize upscaled implementations of the proposed carbonation approach, which is already more advantageous than state-of-the-art approaches. Emerging carbonation approaches involving the carbonation of wastewater are also being proposed for applications in concrete manufacturing and may be considered as a valuable pathway 57 , 58 . Notably, the approach proposed in this work could also carbonate appropriately treated wastewater instead of fresh water for the purpose of concrete manufacturing.
Carbon footprint
Carbon footprint is another key metric that can help determine the competitiveness of any CO 2 capture technology. Based on a Cradle-to-Gate Life Cycle Assessment (LCA), classical cement manufacturing has a global warming potential of 519 kg CO 2 -eq per functional unit, with 311.4 kg CO 2 -eq from fuel burning and 207.6 kg CO 2 -eq from limestone calcination. By assuming to carbonate the same functional unit of cement with the proposed approach for subsequent use in concrete, the global warming potential is reduced to 500.3 kg CO 2 -eq. In other words, the production of one ton of cement leads to a reduction of 18.7 kg CO 2 -eq in global warming potential. When applied to the entire global cement production, this amounts to a substantial reduction of 82.28 million tons of CO 2 emissions 59 , 60 . Put into perspective, this reduction is approximately equivalent to the annual CO 2 emissions of 18 million automobiles resorting to combustion 61 .
Promisingly, the developed LCA calculations unfavorably consider various aspects in the calculations, which would likely result in even more positive outcomes in the carbon footprint of industrial applications of the proposed technique. First, the carbon footprint calculations use a CO 2 capture efficiency of 26.2%. However, the actual CO 2 capture efficiency in upscaled applications of the proposed approach can be significantly higher due to the longer retention time of CO 2 in the mixer CO 2 . Second, CO 2 injection is considered a one-round procedure. However, the CO 2 injection in an upscaled process can be recursively applied or implemented with a looping system, increasing the CO 2 capture efficiency. Third, the proportion of carbonated cement for a functional unit is set at 1/4, which aligns with the laboratory conditions considered in this work. However, this proportion can be increased in an upscaled process, as long as the strength of the carbonated concrete is not compromised.
In summary, the innovative suspension-based concrete carbonation approach developed here not only demonstrates a reduced footprint but also exhibits potential for further scalability, rendering it an attractive candidate for industrial applications. This approach effectively addresses two prominent concerns within the domain of carbon capture through concrete carbonation. The first concern pertains to the potential weakening of concrete strength as a result of carbonation, necessitating additional cement usage to compensate for strength loss. This study reveals that by facilitating carbonate crystal depositions in the cement suspension phase, the proposed approach can, in fact, maintain an uncompromised strength. The second concern relates to the energy consumption associated with the carbonation treatment process, which encompasses CO 2 purification, pumping, transportation, and the use of high-pressure vessels. The cumulative effects of these processes could render the concrete carbonation approach carbon-positive 5 . However, the approach developed in this study minimizes energy consumption during CO 2 introduction through simplified and less rigorous procedures.
Conclusions
This work introduced an approach to trap CO 2 into fresh concrete by carbonating a portion of its cement in suspension. Laboratory experiments and LCA calculations support that the developed approach: (1) involves an active absorption of CO 2 during concrete manufacturing as opposed to conventional concrete production procedures; (2) benefits from a CO 2 capture efficiency of up to 45%; and (3) yields carbonated concrete with an uncompromised strength. The study provides a mechanistic analysis of the physico-chemical processes that characterize the proposed carbonation approach, which not only may underpin future enhancements of the proposed method but also inform the development of other approaches to carbonate fresh concrete and cementitious materials. By modifying classical concrete manufacturing processes only minimally, the proposed carbonation approach offers promise for industrialization. The possibility to enhance the treatment efficacy of the proposed approach in industrial scenarios, as described in this work, further supports its potential to decarbonize the cement and concrete industries.
The promise of the carbonation approach proposed in this work is assessed through four central sets of experiments:
Set 1 : The first set of experiments applies CO 2 injections to cement suspensions.
Set 2 : The second set of experiments imposes the same CO 2 injections on virtually equivalent cement suspensions, which are subsequently mixed with additional cement to achieve a cement paste of the desired water-to-cement ratio.
Set 3 : The third set of experiments imposes the same CO 2 injections to virtually equivalent cement suspensions, which are subsequently mixed with additional cement and aggregates to achieve a concrete mix design.
Set 4 : The fourth set of experiments imposes different CO 2 injection flow rates but reaches the same volume of injected CO 2 via different injection durations in cement suspensions.
Set 1 allows determining the chemical and physical properties of carbonated cement suspensions. Set 2 allows assessing the chemical and physical properties of cement pastes deriving from carbonated cement suspensions. Set 3 allows assessing the strength of concrete deriving from carbonated cement suspensions subsequently mixed with additional cement and aggregates. Set 4 allows to expand on the mechanistic understanding of the physico-chemical processes that characterize the proposed carbonation approach with specific reference to the role of temperature and amount of injected CO 2 on the results of the carbonation. Experiments without any CO 2 injection are also performed for reference.
Materials preparation
The materials addressed by experimental sets 1–3 are prepared by using a 150 mL beaker and following the steps detailed hereafter (Fig. 6 ). Initially, 100 mL of deionized water (DI-H 2 O) with a resistivity of 18.2 MΩ cm is introduced into the beaker. Next, CO 2 gas of high purity (99.9%) is introduced into the DI-H 2 O at a flow rate of 200 standard cubic centimeters per minute (sccm) for a duration of 20 min while stirring is continuously imposed. This process ensures the establishment of a CO 2 -saturated condition and maintains a consistent initial condition for each test. Meanwhile, experiments support that the absence of this step does not lead to different outcomes associated with the carbonation process of the cement suspensions. Subsequently, 50 g of Type 1 Portland cement (CEM I 42.5R) obtained from Vigier Cement Inc. is added to the CO 2 -saturated DI-H 2 O. Afterwards, CO 2 gas is injected into the prepared cement suspension for 10, 20, and 40 min. CO 2 injection flow rate is controlled by a mass flow controller (Alicat MC-300SCCM-D). The carbonated cement suspension achieved at this stage is the material addressed by experimental set 1, which, after preparation is moved to a Petri dish, dried in a CO 2 -free desiccator under 0% relative humidity and room temperature (~22 °C) for 24 h, and pulverization in an agate mortar for subsequent characterization.
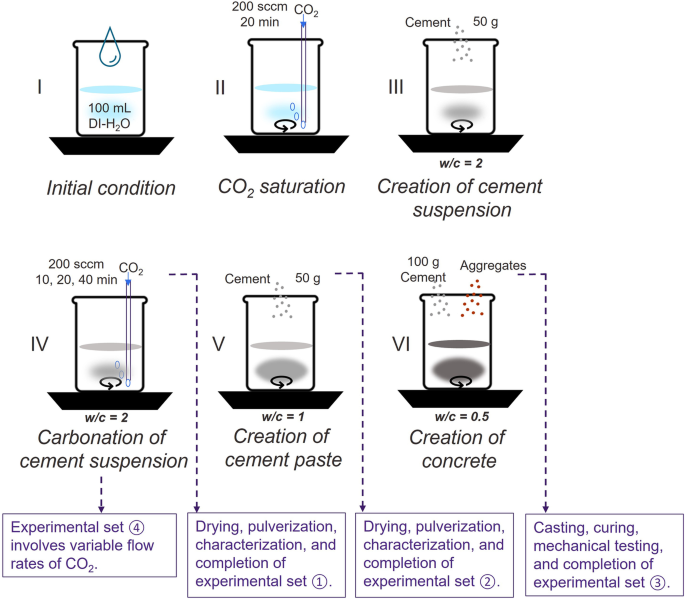
(Step I) Initial condition; (Step II) CO 2 saturation; (Step III) Creation of cement suspension; (Step IV) Carbonation of cement suspension; (Step V) Creation of cement paste; (Step VI) Creation of concrete.
After the achievement of a carbonated cement suspension, experimental set 2 involves the addition of another 50 g of cement to achieve a fresh cement paste that is stirred for another 10 min. The cement paste achieved at this stage is the material addressed by experimental set 2, which is subjected to characterization following the same procedure employed for experimental set 1.
After the achievement of a cement paste deriving from a carbonated cement suspension, experimental set 3 involves the addition and mixing of cement and natural rounded crystal quartz sands and gravel sourced from Carlo Bernasconi AG Inc. to achieve a concrete mix design of a prescribed mass of constituents (Table 1 ). The resulting concrete achieved at this stage is the material addressed by experimental set 3, which is promptly molded into cylindrical specimens measuring 33 mm in diameter and 67 mm in height. A general-purpose lubricant (WD-40) is applied to the inner walls of the molds to facilitate the demolding process. The specimens are demolded following casting and a curing period of 24 h in a desiccator with a relative humidity of 100% and no CO 2 . Subsequently, they are subjected to curing in a dedicated room with a relative humidity of 100% for various durations: 3, 7, 28, and 56 days. Finally, the resulting concrete specimens are subjected to testing. Each experimental condition is analyzed based on the results of ten replicates.
Two sets of considerations are noteworthy with respect to the approach proposed here. On the one hand, although concrete preparation standards for laboratory use, such as ASTM C192 62 , do not specify a particular type of water, it is indeed recognized that tap water is more commonly utilized for concrete manufacturing as opposed to the deionized water employed for this work. Nevertheless, deionized water was chosen for this study to minimize variations in the properties (e.g., mineral content, pH, CO 2 saturation level) of the materials to be carbonated and hence achieve optimal control of the experimental conditions for the sake of this scientific investigation. In alignment with industrial approaches, the life cycle assessment conducted for this study indeed considered tap water, rather than deionized or distilled water, to provide representative results of real-world conditions.
On the other hand, although concrete mix designs with variable (e.g., lower) quantities of cement may be used in practice compared to those used for this work, such an aspect does not compromise the observed performance of the proposed approach to carbonate fresh concrete (see the “Results” section). In fact, only a minimal fraction of the total amount of cement that characterizes the concrete mix design chosen for this work is initially carbonated through the proposed approach. As this amount of cement is limited and lower than that considered in most concrete mix designs employed in practice, and its carbonation yields a carbon capture efficiency that remains superior compared to state-of-the-art approaches (even if not 100% efficient, as shown in the “Results” section and highlighted in the “Discussion” section), it is possible to consider the proposed approach, and especially its overarching methodological principle, as valuable alternatives compared to state-of-the-art methods.
Materials characterization
The characterization of the cement suspensions and cement pastes resorts to pH and temperature measurements, scanning electron microscopy (SEM), X-ray diffraction analyses (XRD), and thermogravimetric analyses (TGA). The characterization of the concrete resorts to unconfined compressive strength tests.
pH and temperature measurements resort to an Elite pocket tester by Thermo Scientific. They allow us to measure the considered variables during the preparation and possible carbonation of cement suspensions and pastes. The reported pH values during carbonation refer either to immediate measurements after a certain duration of CO 2 injection (“instant pH “ in Fig. 3a ) or to measurements collected for stabilized solutions after stirring them for 10 min to let excessive hydroxide donors (if present) to dissolve (“equalized pH” in Fig. 3a ).
SEM analyses use JEOL JSM-7900FLV equipment facilitated with energy-dispersive X-ray spectroscopy (EDS). They allow us to characterize the morphology of dried particles of cement and CaCO 3 crystals.
XRD analyses use an STOE-STADI-P powder diffractometer equipped with an asymmetrically curved Germanium monochromator (CuK α 1 radiation, λ = 1.54056 Å) and 1D silicon strip detector (MYTHEN2 1K from DECTRIS). The line-focused Cu X-ray tube is operated at 40 kV and 40 mA. Intensity data from diffraction angles of 5°–70° (2 θ ) are collected for 45 min per sample. Phase identification is made by Rietveld refinements using the GSAS-II software for semi-quantitative analysis. Inorganic crystal structure database (ICSD) is used to obtain the standard spectra of crystals. Specifically, ICSD 15194, ICSD 15471, ICSD 18166, ICSD 79550, ICSD 87951, ICSD155395, and ICSD 162744 spectra are used, corresponding to aragonite, portlandite, calcite, belite, C–S–H, ettringite, and alite, respectively.
TGAs employ Netzsch’s simultaneous thermal analysis system and allow to quantify key features of CaCO 3 crystal precipitations from weight loss data, including decarbonation peaks, CO 2 capture rates, and carbonation degree. Approximately 20 mg of the powdered samples are subjected to TGA. The samples are heated from room temperature to 1000 °C at a heating rate of 10 °C/min under an inert N 2 atmosphere. The weight loss occurring between 500 and 900 °C is determined as the total loss of CO 2 resulting from the decomposition of carbonates. The CO 2 capture efficiencies are calculated as follows:
The carbonation degree is calculated as follows:
The theoretical CO 2 sequestration capacity is calculated via the composition of cement outlined in Table 2 . A typical composition of type I cement is utilized to calculate a based on the consideration of calculating a universal value of such an approach to provide a concept of capacity level. While Na 2 CO 3 and K 2 CO 3 both provide alkalinity, they do not have a significant effect on the CO 2 capture capacity calculation in this study because they are both highly soluble in water and CO 3 2− content in the suspension is thus not affected. The alkali content does influence the reaction kinetics, but the composition in Table 2 is solely used for capacity calculation.
Unconfined compressive strength tests are performed with a universal testing machine equipped with circular platens and a closed-loop hydraulic system manufactured by MTS Inc. These tests impose a displacement rate of 0.18 mm/min according to the ASTM C39 63 . The concrete specimens are initially capped using a sulfur-based capping compound (H-2959) obtained from Humboldt Mfg. Co. Capping is applied to achieve smooth bearing surfaces on both ends of the specimens and limit possible stress concentrations that would affect the results of the tests, thereby targeting the achievement of uniform loading conditions during the compression tests.
Carbon footprint analysis
The carbon footprint analysis developed as a part of this study is conducted based on an Environmental Product Declaration provided by CEMEX Polska Sp 64 . The Environmental Product Declaration adheres to standard EN 15804 65 and is verified by ISO 14025 66 . The primary analysis is conducted using a “Cradle-to-Gate” Life Cycle Assessment (LCA). The functional unit considered in the LCA is 1 ton of cement. The LCA process system boundary is shown in Fig. 7 . By following the same “Cradle-to-Gate” scenario as in the existing LCA, the carbon footprint of the developed suspension-based carbonation approach is evaluated within the scope of cement production, considering the additional CO 2 injection process required by such an approach. In this manner, the net reduction of global warming potential characterizing a standard cement production serving subsequent use for concrete production can be assessed.
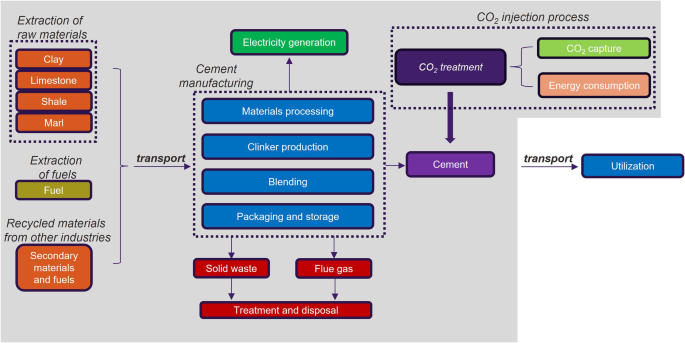
Gray box: Cement production involved in the LCA calculation; Out of the gray box: the subsequent route of cement.
The following assumptions are made to perform the calculations:
40% of the total CO 2 emissions deriving from a cement plant is attributed to fuel combustion, whereas 60% is attributed to limestone calcination 67 .
Flue gas from a cement clinker calcination plant is used as the CO 2 source, with a CO 2 content of 21.76% (dry basis). CO 2 is regarded as the sole active constituent in the gas.
25% of the total employed cement in a given volume of concrete is considered to be carbonated with CO 2 in suspension (i.e., the same ratio applied in the laboratory test), whereas the remaining 75% is subsequently employed after treatment for concrete manufacturing.
Tap water instead of deionized water is used in the calculation for consistency with the classical concrete preparation scenario. Water consumption serving concrete mixing step is excluded because the same amount of water is consumed in a classical concrete manufacturing process.
Commercial Air Pumps (EcoPlus Eco Air 7, each with a power of 200 W) are used for CO 2 injection into the suspension, and their energy consumption is duly considered for the developed carbonation approach.
Each kWh of electricity consumption emits 0.39 kg CO 2 -eq, in alignment with the average value of the US electricity grid 68 .
Irrespective of whether concrete derives from a functional unit of cement carbonated with the proposed approach or not, its following use in practice is assumed to be the same. Therefore, the CO 2 emissions associated with concrete use and recycling are excluded.
A CO 2 injection volume of 8000 scc per 50 g cement is considered as a reference for the carbonation approach. The corresponding CO 2 capture rate of 26.2% (i.e., the lower capture rate achieved in the laboratory experiments for a CO 2 injection lasting 40 min) is also considered.
The continuous mixing process of the suspension-based fresh concrete carbonation approach is considered a part of the mixing process with subsequent concrete mixing with additional cement and aggregates; thus, it is excluded from the calculation.
Data availability
The raw data in support of most of the quantitative figures reported in this work are reported in the Supplementary Information. All of the other data are available from the corresponding author upon reasonable request.
Pörtner, H.-O. et al. Climate Change 2022: Impacts, Adaptation, and Vulnerability. Contribution of Working Group II to the Sixth Assessment Report of the Intergovernmental Panel on Climate Change . IPCC 2022: Summary for Policymakers 3–33 ( I ntergovernmental Panel on Climate Change, 2022).
Deutz, S. & Bardow, A. Life-cycle assessment of an industrial direct air capture process based on temperature–vacuum swing adsorption. Nat. Energy 6 , 203–213 (2021).
Article CAS Google Scholar
Le Quere, C. et al. Temporary reduction in daily global CO 2 emissions during the COVID-19 forced confinement. Nat. Clim.Change 10 , 647 (2020).
Article Google Scholar
Andrew, R. M. Global CO 2 emissions from cement production, 1928–2018. Earth Syst. Sci. Data 11 , 1675–1710 (2019).
Ravikumar, D. et al. Carbon dioxide utilization in concrete curing or mixing might not produce a net climate benefit. Nat. Commun. 12 , 855 (2021).
Batuecas, E. et al. Recycling CO 2 from flue gas for CaCO 3 nanoparticles production as cement filler: a life cycle assessment. J. CO 2 Util. 45 , 101446 (2021).
Wang, J. F., Dai, Y. P. & Gao, L. Exergy analyses and parametric optimizations for different cogeneration power plants in cement industry. Appl. Energy 86 , 941–948 (2009).
Kabir, G., Abubakar, A. I. & El-Nafaty, U. A. Energy audit and conservation opportunities for pyroprocessing unit of a typical dry process cement plant. Energy 35 , 1237–1243 (2010).
Cazacliu, B. & Ventura, A. Technical and environmental effects of concrete production: dry batch versus central mixed plant. J. Clean. Prod. 18 , 1320–1327 (2010).
Valderrama, C. et al. Implementation of best available techniques in cement manufacturing: a life-cycle assessment study. J. Clean. Prod. 25 , 60–67 (2012).
Puertas, F. et al. Ceramic wastes as alternative raw materials for Portland cement clinker production. Cement Concrete Compos. 30 , 798–805 (2008).
Badjatya, P. et al. Carbon-negative cement manufacturing from seawater-derived magnesium feedstocks. Proc. Natl Acad. Sci. USA 119 , e2114680119 (2022).
Article CAS PubMed PubMed Central Google Scholar
Oh, D. Y., Noguchi, T., Kitagaki, R. & Park, W. J. CO 2 emission reduction by reuse of building material waste in the Japanese cement industry. Renew. Sust. Energy Rev. 38 , 796–810 (2014).
Sanna, A., Dri, M., Hall, M. R. & Maroto-Valer, M. Waste materials for carbon capture and storage by mineralisation (CCSM)—a UK perspective. Appl. Energy 99 , 545–554 (2012).
Stefaniuk, D., Hajduczek, M., Weaver, J. C., Ulm, F. J. & Masic, A. Cementing CO2 into C–S–H: a step toward concrete carbon neutrality. PNAS Nexus 2 , pgad052 (2023).
Article PubMed PubMed Central Google Scholar
Zhang, Y. H., Wang, R. X., Liu, Z. Y. & Zhang, Z. P. A novel carbonate binder from waste hydrated cement paste for utilization of CO. J. CO2 Util. 32 , 276–280 (2019).
Slegers, P. A. & Rouxhet, P. G. Carbonation of the hydration products of tricalcium silicate. Cement Concrete Res. 6 , 381–388 (1976).
Bukowski, J. M. & Berger, R. L. Reactivity and strength development of CO 2 activated non-hydraulic calcium silicates. Cement Concrete Res. 9 , 57–68 (1979).
Monkman, S., MacDonald, M., Hooton, R. D. & Sandberg, P. Properties and durability of concrete produced using CO 2 as an accelerating admixture. Cement Concrete Compos. 74 , 218–224 (2016).
He, Z., Li, Z. & Shao, Y. X. Effect of carbonation mixing on CO 2 uptake and strength gain in concrete. J. Mater. Civil Eng. 29 , 04017176 (2017).
Qian, X., Wang, J. L., Fang, Y. & Wang, L. Carbon dioxide as an admixture for better performance of OPC-based concrete. J. CO2 Util. 25 , 31–38 (2018).
Taiheiyo-cement. Successful Development of Manufacturing System “CARBOCATCH” to Fix CO 2 into Fresh Concrete . Taiheiyo cement newsletter (2023).
Liu, Z. & Meng, W. N. Fundamental understanding of carbonation curing and durability of carbonation-cured cement-based composites: a review. J. CO 2 Util. 44 , 101428 (2021).
Liu, B. J. et al New perspectives on utilization of CO 2 sequestration technologies in cement-based materials. Constr. Build. Mater. 272 , 121660 (2021).
Zhang, D., Ghouleh, Z. & Shao, Y. X. Review on carbonation curing of cement-based materials. J. CO2 Util. 21 , 119–131 (2017).
Cui, H. Z., Tang, W. C., Liu, W., Dong, Z. J. & Xing, F. Experimental study on effects of CO2 concentrations on concrete carbonation and diffusion mechanisms. Constr. Build. Mater. 93 , 522–527 (2015).
Zhan, B. J., Poon, C. S. & Shi, C. J. Materials characteristics affecting CO 2 curing of concrete blocks containing recycled aggregates. Cement Concrete Compos. 67 , 50–59 (2016).
Kashef-Haghighi, S. & Ghoshal, S. CO 2 sequestration in concrete through accelerated carbonation curing in a flow-through reactor. Ind. Eng. Chem. Res. 49 , 1143–1149 (2010).
Chen, T., Gao, X. & Qin, L. Mathematical modeling of accelerated carbonation curing of Portland cement paste at early age. Cement Concrete Res. 120 , 187–197 (2019).
Zhan, B. J., Xuan, D. X., Poon, C. S. & Shi, C. J. Effect of curing parameters on CO 2 curing of concrete blocks containing recycled aggregates. Cement Concrete Compos. 71 , 122–130 (2016).
Zajac, M., Irbe, L., Bullerjahn, F., Hilbig, H. & Ben Haha, M. Mechanisms of carbonation hydration hardening in Portland cements. Cement Concrete Res. 152 , 106687 (2022).
Monkman, S. & MacDonald, M. Carbon dioxide upcycling into industrially produced concrete blocks. Constr. Build. Mater. 124 , 127–132 (2016).
Monkman, S. & MacDonald, M. On carbon dioxide utilization as a means to improve the sustainability of ready-mixed concrete. J. Clean. Prod. 167 , 365–375 (2017).
He, Z., Jia, Y., Wang, S., Mahoutian, M. & Shao, Y. Maximizing CO 2 sequestration in cement-bonded fiberboards through carbonation curing. Constr. Build. Mater. 213 , 51–60 (2019).
Monkman, S. & Shao, Y. Carbonation curing of slag-cement concrete for binding CO 2 and improving performance. J. Mater. Civ. Eng. 22 , 296–304 (2010).
Morshed, A. Z. & Shao, Y. Optimized process window for fresh concrete carbonation curing. Can. J. Civ. Eng. 41 , 986–994 (2014).
Dixit, A., Du, H. J. & Pang, S. D. Carbon capture in ultra-high performance concrete using pressurized CO 2 curing. Constr. Build. Mater. 288 , 123076 (2021).
Villain, G., Thiery, M. & Platret, G. Measurement methods of carbonation profiles in concrete: thermogravimetry, chemical analysis and gammadensimetry. Cement Concrete Res. 37 , 1182–1192 (2007).
Ogino, T., Suzuki, T. & Sawada, K. The formation and transformation mechanism of calcium-carbonate in water. Geochim. Cosmochim. Acta 51 , 2757–2767 (1987).
Wray, J. L. & Daniels, F. Precipitation of calcite and aragonite. J. Am. Chem. Soc. 79 , 2031–2034 (1957).
Rostami, V., Shao, Y. X., Boyd, A. J. & He, Z. Microstructure of cement paste subject to early carbonation curing. Cement Concrete Res. 42 , 186–193 (2012).
Zhang, D. & Shao, Y. X. Enhancing chloride corrosion resistance of precast reinforced concrete by carbonation curing. ACI Mater. J. 116 , 3–12 (2019).
Google Scholar
Wang, D. & Chang, J. Comparison on accelerated carbonation of β-CS, Ca(OH), and CAF: reaction degree, multi-properties, and products. Constr. Build. Mater. 224 , 336–347 (2019).
Xue, Q. et al. CO 2 -induced evolution of chemical, structural and mechanical properties of reinforced concrete: a review. Constr. Build. Mater. 353 , 129069 (2022).
Zhang, D., Cai, X. H. & Jaworska, B. Effect of pre-carbonation hydration on long-term hydration of carbonation-cured cement-based materials. Constr. Build. Mater. 231 , 104337 (2020).
Lv, H. L., Wu, Y. Z., Fang, Z. N. & Zhou, S. C. Deterioration behavior of reinforced concrete beam under compound effects of acid-salt mist and carbon dioxide. Constr. Build. Mater. 77 , 253–259 (2015).
Zhan, B. J., Xuan, D. X., Poon, C. S. & Shi, C. J. Mechanism for rapid hardening of cement pastes under coupled CO 2 –water curing regime. Cement Concrete Compos. 97 , 78–88 (2019).
Zhang, D. & Shao, Y. X. Early age carbonation curing for precast reinforced concretes. Constr. Build. Mater. 113 , 134–143 (2016).
Gonen, T. & Yazicioglu, S. The influence of mineral admixtures on the short and long-term performance of concrete. Build. Environ. 42 , 3080–3085 (2007).
Leemann, A., Nygaard, P., Kaufmann, J. & Loser, R. Relation between carbonation resistance, mix design and exposure of mortar and concrete. Cement Concrete Compos. 62 , 33–43 (2015).
Bragg, W. L. The refractive indices of calcite and aragonite. Proc. R. Soc. Lond. A 105 , 370–386 (1924).
Zhao, K., Liang, Y. N., Ji, T., Lu, Y. & Lin, X. J. Effect of activator types and concentration of CO 2 on the steel corrosion in the carbonated alkali-activated slag concrete. Constr. Build. Mater. 262 , 120044 (2020).
Bernal, S. A., Provis, J. L., de Gutierrez, R. M. & van Deventer, J. S. J. Accelerated carbonation testing of alkali-activated slag/metakaolin blended concretes: effect of exposure conditions. Mater. Struct. 48 , 653–669 (2015).
Yousuf, S., Shafigh, P. & Ibrahim, Z. The pH of cement-based materials: a review. J. Wuhan Univ. Technol. 35 , 908–924 (2020).
Ritchie, I. M. & Xu, B. A. The kinetics of lime slaking. Hydrometallurgy 23 , 377–396 (1990).
Giles, D. E., Ritchie, I. M. & Xu, B. A. The kinetics of dissolution of slaked lime. Hydrometallurgy 32 , 119–128 (1993).
Lu, L. et al. Wastewater treatment for carbon capture and utilization. Nat. Sustain. 1 , 750–758 (2018).
Monkman, S., Janke, T. & Hanmore, A. NRG COSIA Carbon XPRIZE: carbon-dioxide mineralization in recycled concrete wash water (vol 5, pg 553, 2021). Clean Energy-China 6 , 779–779 (2022).
Tiseo, I. Carbon dioxide emissions from the manufacture of cement worldwide from 1960 to 2021. In Carbon Dioxide Emissions from the Manufacture of Cement Worldwide from 1960 to 2021 ) (Statista, 2023).
Jaganmoha, M. Production volume of cement worldwide from 1995 to 2022. In Production Volume of Cement Worldwide from 1995 to 2022 ) (Statista, 2023).
Environmental Protection Agency US. Greenhouse gas emissions from a typical passenger vehicle. In Greenhouse Gas Emissions from a Typical Passenger Vehicle ) (Environmental Protection Agency US, 2023).
ASTM Standard C192/C192M. Standard Practice for Making and Curing Concrete Test Specimens in the Laboratory. (ASTM International, West Conshohochen, PA, USA, 2002).
ASTM Standard C39/C39M. Compressive Strength of Cylindrical Concrete Specimens. (ASTM International, West Conshohocken, PA, USA, 2005).
Markowska-Cisak, R. Environmental Product Declaration Type III—Group of Cements CEM I 52.5 . In Environmental Product Declaration Type III—Group of Cements CEM I 52.5 ) (CEMEX, 2021).
CEN. EN15804:2012+A2 Sustainability of construction works - environmental product declaration - core rules for the product category of construction product. (2019).
International Organization for Standardization (ISO). Environmental Labels and Declarations - Environmental labelling Type III - Guiding principles and procedures. European Standard ISO 14025.3, (Geneva, 2004).
Jordal, K. et al. CEMCAP—making CO 2 capture retrofittable to cement plants. Energy Proc. 114 , 6175–6180 (2017).
Administration USEI. How much carbon dioxide is produced per kilowatthour of U.S. electricity generation? In How Much Carbon Dioxide is Produced per Kilowatthour of U.S. Electricity Generation? ) (Administration USEI, 2021).
Download references
Acknowledgements
The authors would like to acknowledge CEMEX Innovation Holding Ltd. for its financial support. The authors would also like to acknowledge the NUANCE Center and IMSERC at Northwestern University. This work made use of the EPIC facility of Northwestern University’s NUANCE Center, which has received support from the SHyNE Resource (NSF ECCS-2025633), the IIN, and Northwestern’s MRSEC program (NSF DMR-1720139). This work made use of the IMSERC Physical Characterization facility at Northwestern University, which has received support from the Soft and Hybrid Nanotechnology Experimental (SHyNE) Resource (NSF ECCS-2025633), and Northwestern University.
Author information
Authors and affiliations.
Department of Civil and Environmental Engineering, Subsurface Opportunities and Innovations Laboratory, Northwestern University, 2145 Sheridan Road, Evanston, IL, 60208, USA
Xiaoxu Fu & Alessandro F. Rotta Loria
CEMEX Innovation Holding AG – Brügg Branch, Römerstrasse 13, CH-2555, Brügg, Switzerland
Alexandre Guerini
CEMEX Innovation Holding AG, General-Guisan-strasse 6, CH-6300, Zug, Switzerland
Davide Zampini
You can also search for this author in PubMed Google Scholar
Contributions
Xiaoxu Fu: conceptualization, methodology, data analysis, and writing—original draft; Alexandre Guerini: methodology and writing—reviewing and editing; Davide Zampini: methodology and writing—reviewing and editing; Alessandro F. Rotta Loria: conceptualization, project administration, supervision, and writing—reviewing and editing.
Corresponding author
Correspondence to Alessandro F. Rotta Loria .
Ethics declarations
Competing interests.
The authors declare no competing interests.
Peer review
Peer review information.
Communications Materials thanks the anonymous reviewers for their contribution to the peer review of this work. Primary Handling Editor: Jet-Sing Lee.
Additional information
Publisher’s note Springer Nature remains neutral with regard to jurisdictional claims in published maps and institutional affiliations.
Supplementary information
Rights and permissions.
Open Access This article is licensed under a Creative Commons Attribution 4.0 International License, which permits use, sharing, adaptation, distribution and reproduction in any medium or format, as long as you give appropriate credit to the original author(s) and the source, provide a link to the Creative Commons licence, and indicate if changes were made. The images or other third party material in this article are included in the article’s Creative Commons licence, unless indicated otherwise in a credit line to the material. If material is not included in the article’s Creative Commons licence and your intended use is not permitted by statutory regulation or exceeds the permitted use, you will need to obtain permission directly from the copyright holder. To view a copy of this licence, visit http://creativecommons.org/licenses/by/4.0/ .
Reprints and permissions
About this article
Cite this article.
Fu, X., Guerini, A., Zampini, D. et al. Storing CO 2 while strengthening concrete by carbonating its cement in suspension. Commun Mater 5 , 109 (2024). https://doi.org/10.1038/s43246-024-00546-9
Download citation
Received : 16 January 2024
Accepted : 17 June 2024
Published : 26 June 2024
DOI : https://doi.org/10.1038/s43246-024-00546-9
Share this article
Anyone you share the following link with will be able to read this content:
Sorry, a shareable link is not currently available for this article.
Provided by the Springer Nature SharedIt content-sharing initiative
Quick links
- Explore articles by subject
- Guide to authors
- Editorial policies
Sign up for the Nature Briefing: Anthropocene newsletter — what matters in anthropocene research, free to your inbox weekly.

Academia.edu no longer supports Internet Explorer.
To browse Academia.edu and the wider internet faster and more securely, please take a few seconds to upgrade your browser .
Enter the email address you signed up with and we'll email you a reset link.
- We're Hiring!
- Help Center
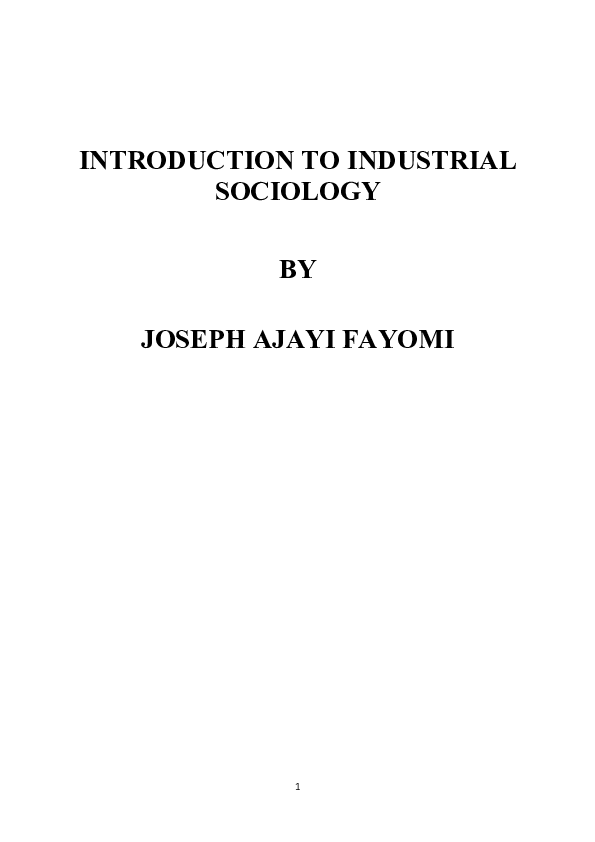
INTRODUCTION TO INDUSTRIAL SOCIOLOGY

Introduction to Industrial Sociology
Loading Preview
Sorry, preview is currently unavailable. You can download the paper by clicking the button above.
RELATED TOPICS
- We're Hiring!
- Help Center
- Find new research papers in:
- Health Sciences
- Earth Sciences
- Cognitive Science
- Mathematics
- Computer Science
- Academia ©2024

COMMENTS
research and writing in the field of industrial. sociology. These issues are approached under three principal rubrics, not wholly exclusive. of each other: (i) technical and methodo-. logical questions, (2) problems of interests. and values arising from the "applied" or "practical" character of research, and (3) possible areas of research that ...
Study of the formal structure of economic organizations - the. vision of labor and the lines of communication and authority is conducted mainly as part of the study of administration. though this type of investigation is relatively well developed. regard to some kinds of organizations, such as hospitals, its.
Explore the latest full-text research PDFs, articles, conference papers, preprints and more on INDUSTRIAL SOCIOLOGY. Find methods information, sources, references or conduct a literature review on ...
Directions of Research in Industrial Sociology* Thomas H. Patten, Jr. University of Detroit A fruitful beginning point for sociological analyses of industry is the concept of status. This concept can be used by itself or in conjunction with other theoretical ideas in the analysis of such diverse areas as work-group behavior, bureaucracy, super?
cal studies to enhance our understanding of work in industrial society. This chapter presents an overview of this work. It is intended to be intelligible both to those possessing a prior knowl edge of sociology as well as those new to the subject. A selection of the sociological perspectives and themes on work in industrial
T oday, however, IR institutionalism is besieged, on. all sides, by an army of academic critics. from neo-classical economics, managerial. [13:01 18/12/2007 5055-Blyton-Ch03.tex] Paper: a4 Job No ...
Corpus ID: 142808771; Industrial sociology and industrial relations : an assessment of the contribution which industrial sociology can make towards understanding and resolving some of the problems now being considered by the Royal Commission
The Journal of Management Studies is a multidisciplinary business and management journal advancing the fields of management and organization.
1951. nOCIOLOGISTS HAVE done much work on motivation, yet most of this ois concerned with the capitalist entrepreneur who attracted attention as LJthe key individual in the system of free enterprlse.l As…. Expand. 24. Semantic Scholar extracted view of "INDUSTRIAL SOCIOLOGY: THEORY, RESEARCH AND TEACHING-SOME PROBLEMS AND PROPOSALS" by T ...
The substantive scope of sociology is an asset, not a liability. 3.4 Many sociologists have called for a tight engagement between sociology as a science and sociology as activism. Throughout its history, sociology has never existed in a social vacuum. Pressing social issues have persistently been a fertile ground for research problems.
This research paper wills review the leather Industrial development and its impacts on the society, case of Oromia region. The study will uses primary and secondary data sources. Survey questionnaires, Interview with key respondents will use while field visit with community leaders to have deep understanding of the situation.
His research looks at the effects of the Fourth Industrial Revolution and how this will affect capital-labour dynamics. Alan Southern is at the University of Liverpool Management School. His research looks at how the whole economy can become socialised, arguing that social organisations, the public sector and the role of labour will be critical ...
Industrial Sociology is a relatively young branch of sociology. While Durkheim and Max Weber made some analysis of industrial institutions, industrial sociology as a separate branch got its push due to the famous experiments at Hawthorne Works in Chicago, of the Western Electric Company, conducted by George Elton Mayo and his associates between 1924 and 1932.
Ames Research Center; Software. Internet Arcade ... Industrial Sociology and Industrial Relations . Exceptional insight provided in this seminal work! Its' simplicity in presenting an eternal truth about human behavior is its' strength. It's a shame Mr Fox' paper is not mandatory reading for all aspiring leaders and managers. 378 ...
Title. Industrial Sociology and Industrial Relations: An Assessment of the Contribution which Industrial Sociology Can Make Towards Understanding and Resolving Some of the Problems Now Being Considered by the Royal CommissionIndustrial sociology and industrial relations. 3Issue 3 of Research papers, Great Britain Royal Commission on Trade ...
This paper discussed Sociology and Industrial Life in a modern society. It explains the operations of the industrial Revolution as a precursor to society and discipline and presupposes the factory system, industrialization and modernity as the outcome of contemporary societies. The paper used a content analysis involving secondary data such as ...
However, the subdiscipline of industrial sociology is generally considered to have begun with the famous Western Electric research program conducted at the Hawthorne Works in Chicago (Whyte 1968). These studies, conducted during much of the Great Depression, were designed to understand the factors involved in worker productivity (Simpson 1989).
Industrial Sociology, also known as 'the study of work organizations' a term which came into use in the ... But for proper understanding of the paper and for us to dig into the foundation ... That research activity eventually became known as industrial sociology and represented, for a time, one of the most vibrant sociology sub disciplines. For ...
Recent Trends in Industrial Sociology. Paper read at the Eighteenth Annual Convention of the American Catholic Sociological Society, Marquette University, Milwaukee, Wisconsin, December 27-29, 1956. The field of industrial sociology has been divided by J. B. Knox into three rather eonvenient areas. The loeus of study may be "human relations in ...
This paper discussed Sociology and Industrial Life in a modern society. It explains the operations of the industrial Revolution as a precursor to society and discipline and presupposes the factory system, industrialization and modernity as the outcome of contemporary societies. ... This research paper of industrial development and its impact on ...
1. Industrial sociology and industrial relations: an assessment of the contribution which industrial sociology can make towards understanding and resolving some of the problems now being considered by the Royal Commission. 1966, H.M.S.O. in English.
This paper discussed Sociology and Industrial Life in a modern society. It explains the operations of the industrial Revolution as a precursor to society and discipline and presupposes the factory system, industrialization and modernity as the outcome of contemporary societies. ... He joined in Dept of Industrial Research in Harvard University ...
While sociology can sometimes feel like a subject with many more questions than answers, SOC5 is refreshing in its slightly more concrete methodology. When quantitative and qualitative methods are both considered, I have felt that sociology becomes slightly more approachable. I highly recommend anyone with the option to take the paper!'
They bring a wealth of academic, research, and leadership abilitiesMINNEAPOLIS / ST. PAUL (07/01/2024)—University of Minnesota College of Science and Engineering Dean Andrew Alleyne has named four new department heads in the college. All bring a wealth of academic, research, and leadership abilities to their departments.Department of Chemical Engineering and Materials ScienceProfessor Kevin ...
Cement is a key constituent of concrete and offers a large sequestration potential of carbon dioxide (CO2). However, current concrete carbonation approaches are hindered by low CO2 capture ...
The method of this research was crosssectional with 34 unit samples of food stalls in Mulyorejo Urban Village. The samples were inserted in plastic and tested in the laboratory with the Escherichia coli parameter. ... They also conduct research and publish scholarly papers and books. Industrial sociology graduates can choose to become lecturers ...