To read this content please select one of the options below:
Please note you do not have access to teaching notes, craft beer – a systematic literature review and research agenda.
International Journal of Wine Business Research
ISSN : 1751-1062
Article publication date: 3 November 2021
Issue publication date: 5 May 2022
In recent years, the craft beer (CB) industry has gained impetus and has experienced significant growth in scientific publications. This study aims to present a systematic review of the literature on CB in areas related to economic and business sciences.

Design/methodology/approach
Based on the data from Scopus, Web of Science and a set of articles not indexed to these databases until June 2021, a total of 132 articles were included for analysis, using bibliometric and content analysis techniques.
The study allowed us to identify that CB has four main clusters/themes of research, namely, CB industry and market, marketing and branding, consumer behavior and sustainability. Detailed information on the clusters is provided. In addition, the results showed that publications addressing CB have grown significantly from 2015 onwards and are dispersed across many journals, with none assuming a clear leadership. Quantitative approaches account for more than half of publications.
Research limitations/implications
This study is a useful guide for academics intending to develop studies with CB. It provides a framework to structure future research by identifying existing literature clusters and proposes several research propositions.
Practical implications
The findings from this study are useful for CB companies to get an overview of the main issues affecting the CB industry and market to be able to adapt their strategies and stay aligned with market tendencies in the four main clusters identified.
Originality/value
This is the first systematic review of CB. Therefore, it provides a significant contribution to frame and strengthening the literature on CB and serves as a reference for future research. Based on the content analysis and cluster identification, the findings portray the status of current research. Accordingly, a set of research opportunities are offered.
- Consumer behaviour
- Globalization
- Systematic literature review
- Conceptual/theoretical
Acknowledgements
The authors thank NECE-UBI, Research Centre for Business Sciences, Research Centre funded by FCT – Fundação para a Ciência e a Tecnologia, IP, under the project UIDB/04630/2020.
Nave, E. , Duarte, P. , Rodrigues, R.G. , Paço, A. , Alves, H. and Oliveira, T. (2022), "Craft beer – a systematic literature review and research agenda", International Journal of Wine Business Research , Vol. 34 No. 2, pp. 278-307. https://doi.org/10.1108/IJWBR-05-2021-0029
Emerald Publishing Limited
Copyright © 2021, Emerald Publishing Limited
Related articles
All feedback is valuable.
Please share your general feedback
Report an issue or find answers to frequently asked questions
Contact Customer Support
Open Access is an initiative that aims to make scientific research freely available to all. To date our community has made over 100 million downloads. It’s based on principles of collaboration, unobstructed discovery, and, most importantly, scientific progression. As PhD students, we found it difficult to access the research we needed, so we decided to create a new Open Access publisher that levels the playing field for scientists across the world. How? By making research easy to access, and puts the academic needs of the researchers before the business interests of publishers.
We are a community of more than 103,000 authors and editors from 3,291 institutions spanning 160 countries, including Nobel Prize winners and some of the world’s most-cited researchers. Publishing on IntechOpen allows authors to earn citations and find new collaborators, meaning more people see your work not only from your own field of study, but from other related fields too.
Brief introduction to this section that descibes Open Access especially from an IntechOpen perspective
Want to get in touch? Contact our London head office or media team here
Our team is growing all the time, so we’re always on the lookout for smart people who want to help us reshape the world of scientific publishing.
Home > Books > New Advances on Fermentation Processes
Craft Beers: Current Situation and Future Trends
Submitted: 13 February 2019 Reviewed: 02 October 2019 Published: 27 November 2019
DOI: 10.5772/intechopen.90006
Cite this chapter
There are two ways to cite this chapter:
From the Edited Volume
New Advances on Fermentation Processes
Edited by Rosa María Martínez-Espinosa
To purchase hard copies of this book, please contact the representative in India: CBS Publishers & Distributors Pvt. Ltd. www.cbspd.com | [email protected]
Chapter metrics overview
1,922 Chapter Downloads
Impact of this chapter
Total Chapter Downloads on intechopen.com

Total Chapter Views on intechopen.com
Overall attention for this chapters
During the twentieth century, the consolidation of large multi-national beer companies and the homogenization of the specified beer types have led to a considerable growth in the beer industry. However, the growing demand by consumers of a single and distinctive product, with a higher quality and better sensory complexity, is allowing for a new resurgence of craft beer segment in recent years. This chapter reviews some different alternatives of innovation in the craft brewing process: from the bottle fermented beers with non-Saccharomyces yeast species, to the use of special malts or specific adjuncts, hop varieties, water quality, etc. All of them open a lot of new possibilities to modulate flavor and other sensory properties of beer, reaching also new consumers looking for a specific story in one of the oldest fermented beverages.
- non-Saccharomyces
- new adjuncts
- bottle fermentation
Author Information
María jesús callejo *.
- Universidad Politécnica de Madrid, Spain
Wendu Tesfaye
María carmen gonzález, antonio morata.
*Address all correspondence to: [email protected]
1. Introduction
Beer brewing is an established ancient art in different civilization and cultures, but there is no a precise and unanimous agreement on the origin of beer. Recent evidences predominantly based on the archeological and historical evidences explain the origin of brewing across time and space [ 1 ]. The timespan for its existence differs over a wide range of geography, from as far back as “The Neolithic Revolution” to the early horizon in South America. It commenced in the agricultural or “Neolithic” revolution period as early as 9000 BC with the advent of the Sumerians in the lowlands of the Mesopotamian alluvial plane [ 2 , 3 ]. Evidence of rice-based fermented beverage has been found in between 7000 and 5000 BC in China [ 4 , 5 , 6 , 7 ] and ancient Mesopotamia back to about 6000 BC [ 8 , 9 , 10 ]. Similarly, in Northern Africa highlighting Egypt at about 3500 BC [ 11 ], in Europe around 3000 BC [ 12 , 13 ] and in South America 900-200 BC [ 14 , 15 , 16 ], locally fermented alcoholic beverages have been produced. Recent starch [ 17 ] and chemical residue studies [ 18 ] extend this period as far as 11,000 BC. In broader terms, all these fermented beverages may be considered as a craft beer based on the production scale.
2. Craft beer: as a movement from bottom to top fermentation. The reemergence of craft brewing
Different cultures and different civilization historically produced a number of fermented beverages/beer with different raw materials, which allowed them to have different attributes and different names. Beer is a relatively simple fermented product, mainly water in its composition, which makes easily produced locally; however, for a long time the difficulty to move long distances permits to flourish craft brewers everywhere in the world [ 19 ]. However, at present, it is not an impediment due to technological advances and transportation progress.
The craft beer movement or revolution began in the USA after the 13 years of national prohibition of alcohol or “the noble experiment” 1919–1933. In 1965, Fritz Maytag, the man of the craft beer renaissance, bought the Anchor Steam Beer Company of San Francisco with a capacity of 50,000 barrels and developed it as a craft brewery outlet [ 20 , 21 ]. Regarding the USA, this was the milestone to the expanding innovation and an increasing trend in terms of production and sales of beers with differentiated quality.
Even though this movement marked a shift in several countries recently, to mention some, in 1988 the earliest brewpub lay foundation in Italy [ 22 ], while in the Netherlands the craft revolution rouse during the year 1981 [ 23 ], in Australia, craft brewing started late 1984 [ 24 ]. At the same time, it is very difficult to put a time limit for the beginning of craft beer production in some European countries like the UK, Belgium and Germany where these countries were either with a long tradition in “special beers” or the historical existence of small and local producers back to the 1970s [ 25 , 26 ].
3. Craft beer: statistical viewpoint
One of the indicators for the expansion of craft beer renaissance in different countries is the statistical approach. However, there is no common shared definition of craft beer but different associations and entities of different countries remark based on the size of the firm, production volume raw materials used to produce such drink, degree of independence and way to brew [ 27 ]. Even though there is data scarcity on the numbers of microbreweries of different countries, in Table 1 , those microbreweries actually existing in different countries of the five continents are represented, where beer production is traditional or its consumption is highly relevant at present. The statistical data reflected in this chapter encompasses all beer producers recognized as craft brewery, artisanal brewery, microbrewery, independent brewery, specialty brewery, Brewpub, local brewery, Regional brewery and Contract brewing company in accordance with the regulation rules of different countries without establishing any distinctions among them.
2008 | 2009 | 2010 | 2011 | 2012 | 2013 | 2014 | 2015 | 2016 | 2017 | |
---|---|---|---|---|---|---|---|---|---|---|
Europe | 1755 | 2123 | 2407 | 2670 | 3094 | 3616 | N/A | N/A | N/A | N/A |
Czech Republic1 | 57 | 51 | 65 | 90 | 20 | 207 | 238 | 202 | 350 | 402 |
France1 | N/A | 263 | 322 | 373 | 293 | 504 | 566 | 690 | 850 | 1000 |
Germany1 | 594 | 628 | 646 | 659 | 665 | 668 | 677 | 723 | 738 | 824 |
The Netherlands | N/A | N/A | 115 | N/A | N/A | N/A | 222 | 380 | 434 | N/A |
Poland | 79 | 89 | 107 | 133 | 164 | 212 | 263 | 308 | N/A | N/A |
Spain | 21 | 27 | 46 | 70 | 114 | 203 | 314 | 409 | 465 | 502 |
The UK | 671 | 694 | 778 | 898 | 1250 | 1440 | 1414 | 1828 | 2198 | 2378 |
America | ||||||||||
The USA | 1321 | 1596 | 1754 | 2016 | 2420 | 2898 | 3739 | 4544 | 5424 | 6266 |
Canada | N/A | N/A | 277 | N/A | N/A | N/A | N/A | 610 | 612 | N/A |
Africa | ||||||||||
South Africa | 22 | 22 | 27 | 34 | 54 | 63 | N/A | N/A | N/A | N/A |
Asia | ||||||||||
Japan | N/A | N/A | 208 | N/A | N/A | N/A | N/A | 222 | N/A | N/A |
Oceania | ||||||||||
Australia | N/A | N/A | 172 | N/A | N/A | N/A | N/A | 358 | 410 | N/A |
New Zealand | N/A | N/A | 62 | 59 | 65 | 79 | 98 | 111 | 130 | N/A |
Microbrewery expansion in the last decade sample countries by continent.
[ 24 , 26 ].
Even though Mergers and acquisitions seem to reduce the number of major brewing firms, the total production in volume is not affected. This tendency provides an opportunity to the merged breweries to take advantage over the microbreweries in terms of economical scale and increased market share. Despite the macrobrewers dominance, worldwide craft beer numbers are increasing at a rapid rate [ 28 ].
4. New tendencies: is the glass half-full or half-empty for brewers?
The last two decades brewing landscape continues to rise in number of micro and craft breweries almost everywhere in the world. As it is shown below ( Table 1 ), in the last decade, from 2008 to 2017, the number of craft breweries significantly increased globally. In fact it passed from 671 to 2378 in the UK (traditionally beer-producing and beer-drinking country), and from 1321 to 6266 in the USA, an increase of 354 and 474% respectively, within the same period (not traditional beer producer country). This increase in number of craft breweries and production volume run up to an increase in compound annual growth rate (CAGR) within the sector. Craft brewing continues to take market share away from the largest brewing companies. According to Brewers Association report (the U.S. beer sales volume growth 2017, National beer sales and production statistical data), the overall U.S. beer volume sales were down 1% in 2017, whereas craft brewer sales continued to grow at a rate of 5% by volume, reaching 12.7% of the U.S. beer market by volume. Craft production grew the most for microbreweries. Retail dollar sales of craft increased 8%, up to $26.0 billion, and now account for more than 23% of the $111.4 billion U.S. beer market [ 27 ]. Percentage of craft beer producers (2013–2017) can be seen in Figure 1 .

Percentage of craft beer producers (2013–2017).
There are various factors, which favored this increase in overall craft beer consumption. These factors include per capita income growth, the availability of alternatives toward the production of successful and high levels of quality beers, increased health concerns, and the emergence of new government regulations that affects directly the sustainability issue and consistency and innovation among many others.
5. Craft and special beers: classification
A single beer style, lager beer, has long been the main dominant beer in the world market. However, a worldwide change in trend for the last decade has been registered due to the growing interest in craft and specialized beer [ 34 ]. A significant growth in the number of breweries, the variety of styles and the total volume of production had been observed in previous years [ 35 ].
But the reasons for the growth are multiple: first, increase in the demand for high more flavorful and stronger beers [ 34 , 36 ]. This is particularly important in the case of American consumers, often not satisfied with the dominant in the market American pale lagers. An increase in flavors (malted barley, chestnut, honey flavored) and a more readily quality perceived are the main factors to choose craft beer instead of commercial beer between habitual beer drinkers [ 37 ]. Second, exclusivity and “unique drinking experiences” are also highly rated by craft beer consumers [ 34 , 38 ]. Finally, even though traditional brands of beer are closely linked to very specific places [ 39 ], craft beer is part of a broader neolocalism movement in which people are demanding goods and services that have a connection with the local community [ 36 ].
Taking into account that all beer types evolve from the combination and relationships among ingredients, processing, packaging, marketing and culture, it is therefore necessary to establish some criteria to establish differences between special and craft beers.
This section analyzes the main criteria for classifying beers as special or craft beers ( Figure 2 ).

Main criteria to classify craft and special beers.
The first element taken into account is the production output of beer per year ( criterion 1 ). Craft beer are characterized by small production output and their “small,” “independent,” and “traditional” character. These characteristics are compatible with others which have been traditionally used to classify beer styles and now they are assuming new importance and making possible to enrich traditional beer brewing: we refer to type of fermentation and yeast strain selection ( criterion 2 ). Here, we will look at non- Saccharomyces brewing yeasts which require special attention [ 40 , 41 ]. While malted barley remains the main source of sugars for fermentation in the production of beer, the ingredients can be changed based on the region and preference of the consumer. Innovative ingredients in wort production can be used as a valuable source of variation in craft beer production ( criterion 3 ) The two last criteria are relatively recent and novel and are related with the development of special beers in the perspective on health and nutrition ( criterion 4 ) and with the use of emerging technologies in brewing ( criterion 5 ).
5.1 Production output of beer per year (scope of craft beer)
The annual beer production allows distinguishing between larger breweries mass-producing beer (annual production capacity of up to 6 million barrels) and craft beers or “small” scale breweries (less than 6 million barrels; where 1 BBL = 339 12 oz bottles of beer or 235 half-liter bottles of beer) [ 26 , 42 ].
Minimum production quantity: Nanobreweries .
The place of sale of beer: production is sold outside (Microbreweries) or on the same floor of production ( Brewpub ).
Brewing companies that outsource their production to other already established breweries ( Contract Brewing Company ).
Over 50% or more of their volume production focuses on all-malt beers and/or their malt flagship ( Regional Craft Brewery ).
The American craft brewing industry assumes that in addition to low volume production, further requirements are expected by the craft beers [ 36 ]. They are independent in that and not more than 25% of the business is owned by another member of the alcohol industry who is not a craft brewer. Traditional ingredients (water, malt, hops, yeast) must also be used in the brewing process although innovation in terms of reinterpreting historic beer styles or developing new styles is a hallmark of the industry.
5.2 Selection of the yeast strain and type of fermentation
The main brewing classification criterion particularly relies on the selection of the yeast strain and type of fermentation [ 35 , 41 ]. Two types of brewing yeast were originally classified based on their flocculation behavior during fermentation.
Beers are classified into two large groups according to the yeast strain and type of fermentation: Ale beers and Lager beers. Ale yeasts or top-fermenting yeasts , which are Saccharomyces cerevisiae strains, rise up to the surface of the vessel with the escaping carbon dioxide gas bubbles and become entangled in the fermentation head, facilitating their collection by skimming.
Ale yeast fermentation temperature ranges between 15 and 20°C. Lager yeast or bottom-fermenting yeast does not rise and becomes entrapped in the foam but settles out at the end of the fermentation. Lager worts often ferment at lower temperatures (8–14°C) than ale yeasts and are therefore much slower.
Ale beers represent only a small percentage of the total beer consumption. They are very common in Britain, Germany, Canada’s eastern provinces, the United States and, last but not least, Belgium. Until the sixteenth century, ale was the main type of beer in Europe [ 43 ].
Standard/ordinary bitter (Britain), English pale ale (Britain), Mild (Britain), Brown Porter (Britain), Robust Porter (Britain), Dry stout (Ireland), Sweet stout (Britain), Kölsch (Germany, Cologne), Lambic (Belgium), Rauchbier (Germany) and Weizen/Weissbier (Germany) are some examples of ale beer types.
Lager beer is the dominant style in almost all countries and represents more than 90% of the beer produced worldwide [ 43 ].
Some principal Lager beer types are: German Pilsner (Pils) (Germany), Bohemian Pilsener (Czech Republic), Classic American Pilsner (United States), Vienna Lager (Austria), Oktoberfest/Märzen (Germany), Dark American Lager (United States), Munich Dunkel (Germany), Schwarzbier (Black Beer) (Germany), Maibock/Helles Bock (Germany), Traditional Bock (Germany), Doppelbock (Germany), Eisbock (Germany).
In all beers cited, the flavor-active compounds such as acids, alcohols, aldehydes, ketones and esters are produced by yeast during fermentation. Although there are many strains of brewing yeast ( Saccharomyces cerevisiae ) for beer production, the choice of suitable yeasts to produce desirable tastes and flavors in beer is very important and significant.
5.2.1 Use of non- Saccharomyces
Several non- Saccharomyces yeasts can be used successfully in the making of craft beers with interesting possibilities. Yeasts such as Lachancea thermotolerans , Torulaspora delbrueckii , Hanseniaspora vineae and Schizosaccharomyces pombe can help to modulate acidity, aroma, mouthfeel or even color [ 41 , 44 ]. As the final alcoholic degree in beers is lower than in wines, and normally ranging between 4 and 8% vol, the use of medium fermentative power non- Saccharomyces species is possible because most of these yeasts are able to ferment reaching this ethanol level.
Lachancea thermotolerans is trending yeast in fermented beverages because of its ability to ferment until 4–9% vol producing high amounts of lactic acid from sugars. Therefore, it can be used to decrease pH of beverages [ 45 , 46 , 47 ]. Moreover, interesting effects in beer aroma can be reached by the production of fruity esters [ 48 ]. The use of L. thermotolerans has been also described in beer technology [ 49 , 50 ]. In the brewing of craft beers, L. thermotolerans can be used not only in the primary fermentation of the wort but also during the second fermentation in bottle to produce the suitable foam and CO 2 pressure. However, the most interesting application is in the production of sour beers because of the natural biological acidification during wort fermentation [ 46 ]. Moreover, even when the early use of L. thermotolerans has been proposed in winemaking in which the use of suitable species of these yeasts can produce pH reductions of 0.5 pH units [ 47 ] and the use in beer technology is even more effective due to the lower buffer effect in beer compared with wine. In our lab, we reached pH reductions of 1 pH unit [ 51 ]. The sensory effect of this acidity is described as a citric acidity without dairy hints because of the low production of acetoin and diacetyl [ 47 ]; moreover, the volatile acidity produced by L. thermotolerans is very low compared to volatile acidity produced by selected S. cerevisiae .
Torulaspora delbrueckii is another versatile yeast suitable for beer production. It has a medium fermentative power and improves the formation of fruity esters in addition to a low production of volatile acidity. These characteristics make it a good yeast for the initial fermentation of the must and the subsequent in bottle [ 50 ]. Also it is possible the use of this yeast sequentially or in mixed cultures with S. cerevisiae [ 52 ] or S. pombe [ 53 ]. It has been described as yeast able to decrease volatile acidity during fermentation. The ability to ferment sugars easily reaching 7–9% vol makes it interesting also for secondary bottle fermentation [ 52 ]. The production of 2-phenylethyl acetate, a floral ester with positive floral aroma, is increased during fermentation with T. delbrueckii ; moreover, high amounts of 3-ethoxy propanol are formed by this species [ 52 ]. The release of polysaccharides is also improved by the fermentation with T. delbrueckii affecting mouthfeel and structure [ 54 ].
Hanseniaspora vineae is an apiculate yeast able to produce fresh and complex fermentation, increasing fruity aroma and producing full bodied structure [ 55 ]. It is possible to find strains with fermentative power close to 9% vol, which facilitate its use not only for primary fermentation but also for bottle fermentation. Moreover, it is a persistent yeast that can be found until the end of the alcoholic fermentation in wines and therefore also in beers because of the lower alcoholic degree. During the fermentation with H. vineae , an increase in the concentration of acetyl esters, benzenoids, and sesquiterpenes [ 56 , 57 ], and a decrease in the contents of alcohols and acids occurs. Intense either β-glucosidase or β-xylosidase activities has been described in some strains of H. vineae increasing the levels of hotrienol and 2,6-dimethyl-3,7-octadien-2,6-diol during fermentation [ 58 ]. It is especially noticeable the production of 2-phenylethyl acetate by H. vineae [ 55 ], compared with other Hanseniaspora / Kloeckera species.
Schizosaccharomyces pombe is a fission yeast able to produce maloalcoholic fermentation, and some strains can reach 13–15% vol of ethanol during fermentation [ 59 , 60 ]. The peculiar metabolism of S. pombe produces an intense degradation of malic acid together with a significant release of pyruvate in the fermentative media [ 60 ]. S. pombe is especially resistant to some common preservatives such as sulfur dioxide, actidione, benzoic acid, and dimethyl dicarbonate [ 59 , 61 ]. The main drawback of this yeast is the high production of volatile acidity. Concerning its structure this species has a peculiar and dense 2-layer cell wall. The autolysis produces the release of high amount of polysaccharides during maturation improving the mouth feel of beers [ 62 ]. This property can be especially interesting to produce full-bodied and soft bottle-aged beers. Moreover, we have observed intense bottle fermentation with good foam properties. The aromatic profile in beers is fruity and fresh when this is yeast is used specially in bottle fermentation.
5.3 Innovative ingredients
Raw material in wort production and parameters in production lead to produce an unlimited number of beer types. It might be argued that beer is a horizontally differentiated product . [ 35 ]. In fact, beers are quite similar in most respects but small differences in their composition can greatly affect both appearance and flavor [ 63 ].
We are going to examine each one of the raw materials separately.
5.3.1 Water
Water is quantitatively the main ingredient of beers; it forms more than 90% and often even more than 94% of the final product. The chemical composition of water has a determinant effect on beer properties and contributes significantly to the final beer flavor. The balance of minerals in brewing water will affect the flavor character and flavor perception of malt, hops, and by-products of fermentation. It may also influence the performance of yeast, which in turn influences the flavor, aroma, and mouthfeel of beer.
Chemical composition of water of the localities where famous beer styles were originated are very different in approximate ionic concentrations (in ppm). The chemical composition of water of Pilzen, Munich, Dortmund or Vienna is typical between Lager examples. Burton-on-Trent, Dublin or Edinburgh are typical between ale examples.
Malted barley is the main source for fermentable sugars used by yeasts in the traditional brewing of beers [ 64 ].
Depending on the conditions (time and temperature), pale or amber-colored or even dark malts are obtained; the color being due to caramelization of sugars and to Maillard-type reactions [ 65 ]. The variety of barely and the malting process influences the type and quality of beer [ 66 ]. To elaborate craft beer, the right malt is a key factor because craft beers include high proportion of adjuncts and enzymatic activity of malt has to ensure adequate hydrolysis of all the starch present in the wort.
5.3.3 Adjuncts
Malted barley is the main source for fermentable sugars used by yeasts in the traditional brewing, Other grains, malted or not, have been included to provide fermentable carbohydrates to the wort in addition to those from malt [ 63 ]. In former times, most cereals were used for malting, emmer, oats, spelt wheat, bread wheat were widely used and, in Estonia, rye was used up until the nineteenth century [ 67 ]. Outside Europe, millet, rice, maize and tuber plants have been, and are still, commonly used.
Bogdan and Kordialik-Bogacka [ 64 ] estimate that 85–90% of beer worldwide is now produced with adjuncts. Traditionally they had been used because they lead to reduce the cost of raw materials. When adjuncts are selected as unmalted grains, they present the added advantage of improved sustainability, by reducing reliance on the malting process [ 68 ] and its associated cost.
Craft brewing is increasing the use of adjuncts [ 68 ] because they lead to create a unique beer flavor/aroma [ 69 ]. Figure 3 shows the influence of different concentrations of roasted malt addition on sensory properties of beer.

Effect of roasted barley addition on beer sensory properties.
Appropriately chosen adjuncts can contribute to light or dark colors, improved colloidal or foam stability and prolongation beer shelf-life [ 64 ]. The flavor profile can also be changed by altering the sugar and amino acid spectra in wort.
Hops ( Humulus lupulus L.) are almost exclusively consumed by the brewing industry. Although hops are only a minority ingredient, they have significant impact on the sensory properties of beer [ 65 ]. It contributes not only to bitter flavor but also with the particular character of the selected hop variety [ 66 ].
This is mainly due to its particular chemical composition in: the hops resins, the hop oil and hop polyphenols [ 70 ].
In the closing years of the twentieth century, the hop became an icon of the “craft beer revolution” that swept across the United States. The “hopped up” vats created more flavorful and aromatic beers, making them more akin to European specialty varieties than anything seen in United States markets since before prohibition. The hops also became an effective marketing tool [ 39 ] from a nutritional and health point of view. It had recently come to light the effect antiviral and anti-HIV of xanthohumol, a phenylated flavonoid isolated from hops [ 66 ].
5.4 Perspective on health and nutrition
This section also includes a part on special or craft beers, which meet the new consumer requirements related with health and nutrition. In this context, it should include categories such as [ 66 ] light or low-calorie beers, low alcohol or non-alcohol beers, gluten free beers and functional beers.
5.4.1 Light beers
Light beer is a relatively new product on the market. Light beers contain at least one-third less calories than conventional beers [ 71 ]. However, these products are not widely accepted in Europe compared to North America and Australasia because of their lack of fullness in the taste and low bitterness compared with conventional beer. Enhanced hop character and addition of a low level of priming syrup have been proposed to the production of a low-calorie beer with a well-balanced and full beer flavor [ 38 ].
From a nutritional point of view [ 71 ], light beer contains less carbohydrate than regular beer, low alcohol beer or non-alcoholic beer. Surprisingly, light beer presents more calorie supply than such beers. This may be explained considering that light beer has a significant amount of alcohol (3%) providing a high calorie value.
5.4.2 Low alcohol beers
Low-alcohol beer is a beer with very low- or no-alcohol content. The alcohol by volume (ABV) limits depends on laws in different countries. In recent years, there has been an increased market share for low alcohol beers . This is mainly due to health and safety reasons and increasingly strict social regulations [ 72 ]. The alcohol-free beers also claim beneficial effects of healthy beer components with a simultaneous effect of the lower energy intake and complete absence of negative impacts of alcohol consumption.
According to Blanco et al. [ 73 ], the dealcoholization processes that are commonly used to reduce the alcohol content in beer have negative consequences to beer flavor. Several processes (physical and biological) have been developed for the production of low-alcohol or alcohol-free beer [ 74 ]. The physical processes include thermal and membrane processes such as thin-layer evaporation; falling film vacuum evaporation; continuous vacuum rectification; reverse osmosis; and dialysis. The biological processes include cold contact process (CCP); arrested fermentation; and use of special yeasts ( S. ludwigii ).
Overall, the taste defects in alcohol-free beer are mainly attributed to loss of aromatic esters, insufficient aldehydes, reduction or loss of different alcohols, and an indeterminate change in any of its compounds during the dealcoholization process or as a consequence of incomplete fermentation [ 73 ].
5.4.3 Gluten free
The market segment for gluten free (GF) products continues to grow rapidly and gluten free beers are a niche market with increasing demand [ 75 , 76 ].
Beer is considered unsuitable for people suffering from gluten intolerance, but with some modification and removal of proteins which occur during traditional beer processing. The majority of the precipitated protein remains in the spent grain after the lautering process and only a small proportion of gluten passes from malt to sweet wort. A study conducted by [ 77 ], in twenty-eight commercial beers, found that 10 of the tested beers contained less than 20 ppm gluten.
There are different alternatives for the reduction of gluten levels below the legislative gluten-free threshold (≤20 ppm) (EC No. 41/2009, 2009), on a daily basis, including precipitation and enzymatic hydrolysis. Deglutinization treatments by enzymatic process were proposed by Fanari et al. [ 78 ].
Furthermore, gluten free beers can be produced using gluten free cereals and pseudocereals . Currently only sorghum, rice, maize, millet, and buckwheat appear to be successful GF beer ingredients, while others have only shown adjunct possibilities. Among cereals, Teff is gaining a lot of popularity in GF beer production. Teff grain nutrients are promising and it is also an excellent GF alternative for people with celiac disease and other gluten allergy. Though the α- and β-amylase activities of teff malt are lower than that of barley, it has sufficient level of enzyme activities to be used as a raw material for malting [ 79 ] and GF beer production. Mayer et al. [ 80 ] has also prepared a GF beer from all-rice malt with sufficient endogenous enzyme activity for degradation of the rice components.
A third approach is the production of yeast fermented beverages based on fermentable sugars/syrups [ 75 ]. The search for new gluten-free brewing materials is still in its infancy and researchers in this field of study are continuously researching on the malting, mashing, fermentation conditions [ 78 ].
5.4.4 Functional beer
There is also scope for positioning low-calorie beers as a source of good carbohydrates, such as the soluble fiber and prebiotics derived from the β-linked glucans and arabinoxylans in the cereal walls [ 81 ]. Because these carbohydrates are neither metabolized by the brewing yeast nor they do not contribute toward calorie count but exert health benefits. Prebiotics are dominantly oligosaccharides that are nondigestible to human being but selectively stimulate growth and activity of beneficial bacteria (probiotics) in the human gastrointestinal tract.
Further, β-glucans could enhance stress tolerance of intestinal lactobacilli, which may have a positive impact on survival of probiotics. Nonetheless, high molecular weight b-linked glucan materials may have a negative impact on filtration efficiency and optimization of a filtration process will be required.
Probiotics are not limited to bacteria, and there is a well-known probiotic yeast strain of S. cerevisiae var. boulardii . A novel unfiltered and unpasteurized probiotic beer could be produced by fermenting wort with a probiotic strain of S. cerevisiae . A new category of functional beer could be the specialty beer of the future, given the rising consumer recognition and acceptance of probiotics [ 38 ].
5.5 Use of new technologies
Emerging technologies as high hydrostatic pressure (HHP) and ultra-high pressure homogenization (UHPH) open new possibilities in beer production. Both technologies are considered as cold techniques allowing the control of microorganisms in beverages [ 82 ]. Even when some temperature increasing is produced that can be quantified in 2–3°C/100 MPa in HHP [ 83 ] by compression adiabatic heat and until 100°C but just for 0.2 s in UHPH because of intense shear forces and impact [ 84 ]. The use of HHP is able to eliminate yeasts at pressures of 400 MPa-10 min but Gram-positive bacteria needs 600 MPa-10 min and spores remain unaffected even with these pressures [ 85 ]. Also it has the drawback of being a discontinuous technology. UHPH is now currently highly developed being a fast technology with a good industrial scale-up with equipment that are working at a flow of 10,000 l/h ( https://www.ypsicon.com/ ). Moreover, UHPH is a continuous technology and able to produce sterilization due to the extreme impacts and shear forces produced when the fluid pumped at 300 MPa cross the depressurization valve [ 84 ]. In beer production theoretically is possible to pump the beer at 300 MPa and release the pressure until 4 bar, later is possible to make a sterile iso-barometric bottling. The intense de-polymerization produced by UHPH can also disaggregate colloidal particles improving the beer structure and stability. Potentially it is possible to produce the mechanically lysis of the yeasts formed during fermentation increasing the amount of small size polysaccharides.
Other interesting technology that can be quite useful in beer production and sterilization is pulsed light (PL). This technology produces high energy light during a very short time (few μs) with a strong capacity to inactivate microorganisms and spores allowing sterilization [ 85 ]. The light is applied by flash lamps with a range spectra of 160–2600 nm with an intensity 105 folds the sunlight intensity at the seaside level. Power peak can reach 35 MW. PL technology is also a cold technology being a gentle process with sensory quality of beverages. This technique can be applied continuously during beer processing previously to packaging. It is also possible to use this technology to sterilize bottles or packages.
The use of these new technologies opens new possibilities in the processing and preservation of beer. UHPH and PL can be applied in a continuous way being efficient and easily implemented at industrial scale. Both sterilization technologies have a gentle repercussion in sensory quality of beverages.
6. Future trends
The development of new craft and special beers will be focused in the improvement on sensory properties and differentiation. Moreover, health care connotations are essential and should be supported by traditional processes but improved with both new biotechnologies and emerging processes.
- 1. Hayden B, Canuel N, Shanse J. What was brewing in the Natufian? An archaeological assessment of brewing technology in the Epipaleolithic. Journal of Archaeological Method and Theory. 2012; 20 (1):102-150. DOI: 10.1007/s10816-011-9127-y
- 2. Dineley M, Dineley G. Neolithic ale: Barley as a source of malt sugars for fermentation. In: Fairbairn AS, editor. Plants in Neolithic Britain and Beyond. Oxford: Oxbow; 2000. pp. 137-154
- 3. Cabras I, Higgins DM. Beer, brewing, and business history. Business History. 2016; 58 :609-624
- 4. McGovern P, Zhang J, Tang J, Zhang Z, Hall G, Moreau R, et al. Fermented beverages of pre- and proto-historic China. Proceedings of the National Academy of Sciences of the United States of America. 2004; 101 (51):17593-17599
- 5. Meussdoerffer FG. A comprehensive history of beer brewing. In: Esslinger HM, editor. Handbook of Brewing. Weinheim: Wiley-VCH Verlag GmbH & Co.; 2009. pp. 1-42
- 6. Bai J, Huang J, Rozelle S, Boswell M. Beer battles in China: The struggle over the World’s largest beer market. In: Swinnen JFM, editor. The Economics of Beer. Oxford: Oxford University Press; 2011. pp. 267-286
- 7. Jiajing W, Li L, Terry B, Linjie Y, Yuanqing L, Fulai X. Revealing a 5,000-y-old beer recipe in China. Proceedings of the National Academy of Sciences of the United States of America. 2016; 113 (23):6444-6448
- 8. Hardwick WA. History and antecedents of brewing. In: Hardwick WA, editor. Handbook of Brewing. New York: Marcel Dekker; 1994. pp. 37-52
- 9. Cortacero-Ramirez S, De Castro MHB, Segura-Carretero A, Cruces-Blanco C, Fernandez-Gutierrez A. Analysis of beer components by capillary electrophoretic methods. Trends in Analytical Chemistry. 2003; 22 (7):440-455
- 10. Michel C. L’alimentation au Proche-Orient ancien: Les sources et leur exploitation. Dialogues d'Histoire Ancienne. 2012; 7 :17-45
- 11. Maksoud SA, Hadidi MN, Amer WN. Beer from the early dynasties (3500-3400 cal. B.C.) of Upper Egypt, detected by archaeochemical methods. Vegetation History and Archaeobotany. 1994; 3 (4):219-224
- 12. Nelson M. The barbarian's Beverage: A History of Beer in Ancient Europe. London/New York: Routledge; 2005. DOI: 10.4324/9780203309124. Available from: https://scholar.uwindsor.ca/llcpub/26
- 13. Poelmans E, Swinnen JFM. From monasteries to multinationals (and back): A historical review of the beer economy. The Journal of Wine Economics. 2011; 6 (2):196-216
- 14. Moore J. Pre-Hispanic beer in coastal Peru: Technology and social context of prehistoric production. American Anthropologist. 1989; 91 (3):682-695
- 15. Burger RL, Van Der Merwe NJ. Maize and the origin of Highland Chavín civilization: An isotopic perspective. American Anthropologist. 1990; 92 (1):85-95
- 16. Hastorf CA, Johannessen S. Pre-Hispanic political change and the role of maize in the Central Andes of Peru. American Anthropologist. 1993; 95 (1):115-138
- 17. Liua L, Wanga J, Rosenbergb D, Zhaoc H, Lengyeld G, Nadel D. Fermented beverage and food storage in 13,000 y-old stone mortars at Raqefet cave, Israel: Investigating Natufian ritual feasting. Journal of Archaeological Science: Reports. 2018; 21 :783-793
- 18. Perruchinia E, Glatza C, Haldb MM, Casanac J, Toneyd JL. Revealing invisible brews: A new approach to the chemical identification of ancient beer. Journal of Archaeological Science. 2018; 100 :176-190
- 19. Howard PH. Too big to ale? Globalization and consolidation in the beer industry. In: Patterson MW, Pullen NH, editors. The Geography of Beer: Regions, Environment, and Society. Dordrecht, The Netherlands: Springer; 2014; pp. 155-165
- 20. Sewell SL. The spatial diffusion of beer from its Sumerian origins to today. In: Patterson M, Hoalst-Pullen N, editors. The Geography of Beer: Regions, Environment, and Society. Dordrecht: Springer; 2014. pp. 23-29. https://doi.org/10.1007/978-94-007-7787-3_3
- 21. Elzinga K, Tremblay C, Tremblay V. Craft beer in the United States: History, numbers, and geography. The Journal of Wine Economics. Dordrecht, Springer; 2015; 10 (3):242-274. DOI: 10.1017/jwe.2015.22
- 22. Garavaglia C. Birth and Diffusion of Craft Breweries in Italy. In: Garavaglia C, Swinnen J, editors. Economic Perspectives on Craft Beer: A Revolution in the Global Beer Industry. London: Palgrave Macmillan; 2017
- 23. van Dijk M, Kroezen J, Slob B. From Pilsner Desert to craft beer oasis: The rise of craft brewing in the Netherlands. In: Economic Perspectives on Craft Beer. Cham, Switzerland: Palgrave McMillan; 2017. pp. 259-293. DOI: 10.1007/978-3-319-58235-1_10. ISBN: 978-3-319-58235-1
- 24. Sammartino A. Craft brewing in Australia, 1979-2015. In: Garavaglia C, Swinnen J, editors. Economic Perspectives on Craft Beer: A Revolution in the Global Beer Industry. London/New York: Palgrave Macmillan; 2018. pp. 397-423
- 25. Depenbusch L, Ehrich M, Pfizenmaier U. Craft Beer in Germany–New Entries in a Challenging Beer Market. In: Garavaglia C, Swinnen J, editors. Economic Perspectives on Craft Beer: A Revolution in the Global Beer Industry. London, New York: Palgrave Macmillan; 2018
- 26. Garavaglia C, Swinnen J. The craft beer revolution: An international perspective. Choices. 2017; 32 (3):1-8. Available from: http://www.choicesmagazine.org/choices-magazine/theme-articles/global-craft-beer-renaissance/the-craft-beer-revolution-an-international-perspective
- 27. Brewers Association. 2019. Retrieved from: https://www.brewersassociation.org/statistics/ [Accessed: April 1, 2019]
- 28. Bamforth C, Cabras I. Interesting times: Changes for brewing. In: Cabras I, Higgins D, Preece D, editors. Brewing, Beer and Pubs: A Global Perspective. London: Palgrave Macmillan; 2016. pp. 13-33
- 29. The Brewers of Europe. Beer Statistics—2018 and Previous Years. 2018. Retrieved from: https://brewersofeurope.org/site/index.php [Accessed: April 1, 2019]
- 30. Australia and New Zealand Banking Group Limited (ANZ). New Zealand craft beer industry insights: ANZ industry reports. 2017. Available from: https://comms.anz.co.nz/businsights/article/report.html?industry=Craft%20Beer [accessed: April 1, 2018]
- 31. Tripes S, Dvořák J. Strategic forces in the Czech brewing industry from 1990-2015. Acta Oeconomica Pragensia. 2017; 3 :3-38
- 32. Rogerson CM, Collins KGE. Developing beer tourism in South Africa: International perspectives. African Journal of Hospitality, Tourism and Leisure. 2015; 4 (1):1-15
- 33. Beer Canada. 2015 Industry Trends . 2016. Available from: http://www.beercanada.com/sites/default/files/2015_industry_trends_final.pdf
- 34. Gómez-Corona C, Lelievre-Desmas M, Buendía HBE, Chollet S, Valentin D. Craft beer representation amongst men in two different cultures. Food Quality and Preference. 2016; 53 :19-28
- 35. Clemons EK, Gao GG, Hitt LM. When online reviews meet hyperdifferentiation: A study of the craft beer industry. Journal of Management Information Systems. 2006; 23 (2):149-171
- 36. Reid N, McLaughlin RB, Moore MS. From yellow fizz to big biz: American craft beer comes of age. Focus on Geography. 2014; 57 (3):114-125
- 37. Smith S, Farrish J, McCarroll M, Huseman E. Examining the craft brew industry: Identifying research needs. International Journal of Hospitality Beverage Management. 2017; 1 (1):3
- 38. Yeo HQ , Liu SQ. An overview of selected specialty beers: Developments, challenges and prospects. International Journal of Food Science & Technology. 2014; 49 (7):1607-1618
- 39. Kopp P. The global hop: An agricultural overview of the brewer’s gold. In: Patterson M, Hoalst-Pullen N, editors. The Geography of Beer. Dordrecht: Springer; 2014
- 40. Tataridis P, Kanelis A, Logotetis S, Nerancis E. Use of non- Saccharomyces Torulaspora delbrueckii yeast strains in winemaking and brewing. Zbornik Matice Srpske za Prirodne Nauke. 2013; 124 :415-426
- 41. Callejo MJ, González C, Morata A. Use of non- Saccharomyces yeasts in bottle fermentation of aged beers. In: Kanauchi M, editor. Brewing Technology. Rijeka, Croatia: IntechOpen; 2017. DOI: 10.5772/intechopen.68793. Available from: https://www.intechopen.com/books/brewing-technology/use-of-non-saccharomyces-yeasts-in-bottle-fermentation-of-aged-beers
- 42. Kleban J, Nickerson I. To brew, or not to brew-that is the question: An analysis of competitive forces in the craft brew industry. Journal of the International Academy for Case Studies. 2012; 18 (3):59
- 43. Pavsler A, Buiatti S. Non-lager beer. In: Beer in Health and Disease Prevention. London, United Kingdom: Academic Press; 2009. pp. 17-30
- 44. Budroni M, Zara G, Ciani M, Comitini F. Saccharomyces and non- Saccharomyces starter yeasts. In: Kanauchi M, editor. Brewing Technology. Rijeka, Croatia: IntechOpen; 2017. DOI: 10.5772/intechopen.68792. Available from: https://www.intechopen.com/books/brewing-technology/saccharomyces-and-non-saccharomyces-starter-yeasts
- 45. Gobbi M, Comitini F, Domizio P, Romani C, Lencioni L, Mannazzu I, et al. Lachancea thermotolerans and Saccharomyces cerevisiae in simultaneous and sequential co-fermentation: A strategy to enhance acidity and improve the overall quality of wine. Food Microbiology. 2013; 33 :271-281. DOI: 10.1016/j.fm.2012.10.004
- 46. Morata A, Loira I, Tesfaye W, Bañuelos MA, González C, Suárez Lepe JA. Lachancea thermotoleran s applications in wine technology. Fermentation. 2018; 4 :53. DOI: 10.3390/fermentation4030053
- 47. Morata A, Bañuelos MA, Vaquero C, Loira I, Cuerda R, Palomero F, et al. Lachancea thermotolerans as a tool to improve pH in red wines from warm regions. European Food Research and Technology. 2019; 245 :885-894. DOI: 10.1007/s00217-019-03229-9
- 48. Escott C, Morata A, Ricardo-da-Silva JM, Callejo MJ, González MC, Suarez-Lepe JA. Effect of Lachancea thermotolerans on the formation of polymeric pigments during sequential fermentation with Schizosaccharomyces pombe and Saccharomyces cerevisiae . Molecules. 2018; 23 :2353. DOI: 10.3390/molecules23092353
- 49. Domizio P, House JF, Joseph CML, Bisson LF, Bamforth CW. Lachancea thermotolerans as an alternative yeast for the production of beer. Journal of the Institute of Brewing. 2016; 122 :599-604. DOI: 10.1002/jib.362
- 50. Callejo MJ, García Navas JJ, Alba R, Escott C, Loira I, González MC, et al. Wort fermentation and beer conditioning with selected non- Saccharomyces yeasts in craft beers. European Food Research and Technology. 2019; 245 :1229-1238. DOI: 10.1007/s00217-019-03244-w
- 51. Vanooteghem M. Impact of non- Saccharomyces fermentations on the flavour profile of craft beer [MS thesis]. Madrid, Spain: Technical University of Madrid; 2019
- 52. Loira I, Vejarano R, Bañuelos MA, Morata A, Tesfaye W, Uthurry C, et al. Influence of sequential fermentation with Torulaspora delbrueckii and Saccharomyces cerevisiae on wine quality. LWT - Food Science and Technology. 2014; 59 :915-922. DOI: 10.1016/j.lwt.2014.06.019
- 53. Loira I, Morata A, Comuzzo P, Callejo MJ, González C, Calderón F, et al. Use of Schizosaccharomyces pombe and Torulaspora delbrueckii strains in mixed and sequential fermentations to improve red wine sensory quality. Food Research International. 2015; 76 :325-333. DOI: 10.1016/j.foodres.2015.06.030
- 54. Comitini F, Gobbi M, Domizio P, Romani C, Lencioni L, Mannazzu I, et al. Selected non- Saccharomyces wine yeasts in controlled multistarter fermentations with Saccharomyces cerevisiae . Food Microbiology. 2011; 28 :873-882. DOI: 10.1016/j.fm.2010.12.001
- 55. Martin V, Valera MJ, Medina K, Boido E, Carrau F. Oenological impact of the Hanseniaspora/Kloeckera yeast genus on wines—A review. Fermentation. 2018; 4 :76. DOI: 10.3390/fermentation4030076
- 56. Martin V, Giorello F, Fariña L, Minteguiaga M, Salzman V, Boido E, et al. De novo synthesis of benzenoid compounds by the yeast Hanseniaspora vineae increases the flavor diversity of wines. Journal of Agricultural and Food Chemistry. 2016; 64 :4574-4583
- 57. Martin V, Boido E, Giorello F, Mas A, Dellacassa E, Carrau F. Effect of yeast assimilable nitrogen on the synthesis of phenolic aroma compounds by Hanseniaspora vineae strains. Yeast. 2016; 33 :323-328
- 58. López S, Mateo JJ, Maicas S. Characterisation of Hanseniaspora isolates with potential aroma enhancing properties in Muscat wines. South African Journal of Enology and Viticulture. 2014; 35 :292-303
- 59. Suárez-Lepe JA, Palomero F, Benito S, Calderón F, Morata A. Oenological versatility of Schizosaccharomyces spp. European Food Research and Technology. 2012; 235 :375-383
- 60. Loira I, Morata A, Palomero F, González C, Suárez-Lepe JA. Schizosaccharomyces pombe : A promising biotechnology for modulating wine composition. Fermentation. 2018; 4 :70. DOI: 10.3390/fermentation4030070
- 61. Escott C, Loira I, Morata A, Bañuelos MA, Suárez-Lepe JA. Wine spoilage yeasts: Control strategy. In: Morata A, Loira I, editors. Yeast-Industrial Applications. London, UK: InTech; 2017. pp. 89-116
- 62. Palomero F, Morata A, Benito S, Calderón F, Suárez-Lepe JA. New genera of yeasts for over-lees aging of red wine. Food Chemistry. 2009; 112 :432-441
- 63. Buiatti S. Beer composition: An overview. In: Beer in Health and Disease Prevention. London, United Kingdom: Academic Press; 2009. pp. 213-225
- 64. Bogdan P, Kordialik-Bogacka E. Alternatives to malt in brewing. Trends in Food Science & Technology. 2017; 65 :1-9
- 65. De Keukeleire D. Fundamentals of beer and hop chemistry. Quimica Nova. 2000; 23 (1):108-112
- 66. Sohrabvandi S, Mortazavian AM, Rezaei K. Health-related aspects of beer: A review. International Journal of Food Properties. 2012; 15 (2):350-373
- 67. Behre KE. The history of beer additives in Europe—A review. Vegetation History and Archaeobotany. 1999; 8 (1-2):35-48
- 68. Kok YJ, Ye L, Muller J, Ow DSW, Bi X. Brewing with malted barley or raw barley: What makes the difference in the processes? Applied Microbiology and Biotechnology. 2019; 103 (3):1059-1067
- 69. Schnitzenbaumer B, Arendt EK. Brewing with up to 40% unmalted oats ( Avena sativa ) and sorghum ( Sorghum bicolor ): A review. Journal of the Institute of Brewing. 2014; 120 (4):315-330
- 70. Steenackers B, De Cooman L, De Vos D. Chemical transformations of characteristic hop secondary metabolites in relation to beer properties and the brewing process: A review. Food Chemistry. 2015; 172 :742-756
- 71. Blanco CA, Caballero I, Barrios R, Rojas A. Innovations in the brewing industry: Light beer. International Journal of Food Sciences and Nutrition. 2014; 65 (6):655-660
- 72. Brányik T, Silva DP, Baszczyňski M, Lehnert R, e Silva JBA. A review of methods of low alcohol and alcohol-free beer production. Journal of Food Engineering. 2012; 108 (4):493-506
- 73. Blanco CA, Andrés-Iglesias C, Montero O. Low-alcohol beers: Flavor compounds, defects, and improvement strategies. Critical Reviews in Food Science and Nutrition. 2016; 56 (8):1379-1388
- 74. Montanari L, Marconi O, Mayer H, Fantozzi P. Production of alcohol-free beer. In: Beer in Health and Disease Prevention. London, United Kingdom: Academic Press; 2009. pp. 61-75
- 75. Hager AS, Taylor JP, Waters DM, Arendt EK. Gluten free beer—A review. Trends in Food Science & Technology. 2014; 36 (1):44-54
- 76. Watson HG, Vanderputten D, Van Landschoot A, Decloedt AI. Applicability of different brewhouse technologies and gluten-minimization treatments for the production of gluten-free (barley) malt beers: Pilot-to industrial-scale. Journal of Food Engineering. 2019; 245 :33-42
- 77. Guerdrum LJ, Bamforth CW. Prolamin levels through brewing and the impact of prolyl endoproteinase. Journal of the American Society of Brewing Chemists. 2012; 70 :35-38
- 78. Fanari M, Forteschi M, Sanna M, Zinellu M, Porcu MC, Pretti L. Comparison of enzymatic and precipitation treatments for gluten-free craft beers production. Innovative Food Science & Emerging Technologies. 2018; 49 :76-81
- 79. Gebremariam MM, Zarnkow M, Becker T. Teff ( Eragrostis tef ) as a raw material for malting, brewing and manufacturing of gluten-free foods and beverages: A review. Journal of Food Science and Technology. 2014; 51 (11):2881-2895
- 80. Mayer H, Ceccaroni D, Marconi O, Sileoni V, Perretti G, Fantozzi P. Development of an all rice malt beer: A gluten free alternative. LWT- Food Science and Technology. 2016; 67 :67-73
- 81. Bamforth CW. Beer, carbohydrates and diet. Journal of the Institute of Brewing. 2005; 111 (3):259-264
- 82. Morata A, Loira I, Vejarano R, González C, Callejo MJ, Suárez-Lepe JA. Emerging preservation technologies in grapes for winemaking. Trends in Food Science & Technology. 2017; 67 :36-43. DOI: 10.1016/j.tifs.2017.06.014
- 83. Bañuelos MA, Loira I, Escott C, Del Fresno JM, Morata A, Sanz PD, et al. Grape processing by high hydrostatic pressure: Effect on use of non- Saccharomyces in must fermentation. Food and Bioprocess Technology. 2016; 9 :1769-1778. DOI: 10.1007/s11947-016-1760-8
- 84. Loira I, Morata A, Bañuelos MA, Puig-Pujol A, Guamis B, González C, et al. Use of ultra-high pressure homogenization processing in winemaking: Control of microbial populations in grape musts and effects in sensory quality. Innovative Food Science and Emerging Technologies. 2018; 50 :50-56
- 85. Morata A, Loira I, Vejarano R, Bañuelos MA, Sanz PD, Otero L, et al. Grape processing by high hydrostatic pressure: Effect on microbial populations, phenol extraction and wine quality. Food and Bioprocess Technology. 2015; 8 :277-286. DOI: 10.1007/s11947-014-1405-8
© 2019 The Author(s). Licensee IntechOpen. This chapter is distributed under the terms of the Creative Commons Attribution 3.0 License , which permits unrestricted use, distribution, and reproduction in any medium, provided the original work is properly cited.
Continue reading from the same book
Published: 05 February 2020
By Octavio García-Depraect, Daryl Rafael Osuna-Laveag...
1156 downloads
By Yonglan Tian, Huayong Zhang and Edmond Sanganyado
995 downloads
By Pascal Drouin, Lucas J. Mari and Renato J. Schmidt
1505 downloads
More than just a beer—the potential applications of by-products from beer manufacturing in polymer technology
- Open access
- Published: 24 September 2021
- Volume 5 , pages 765–783, ( 2022 )
Cite this article
You have full access to this open access article
- Aleksander Hejna ORCID: orcid.org/0000-0001-9125-6164 1
7066 Accesses
13 Citations
1 Altmetric
Explore all metrics
Beer is the most popular alcoholic beverage in the world, and its popularity is continuously growing. Currently, global beer production is estimated at around 2 billion hectoliters. Nevertheless, the increasing production capacity implicates the rising issue of generated by-products—brewers’ spent grain, spent hops, spent yeast, and wastewater. They are generated in massive amounts, so having in mind the current pro-ecological trends, it is crucial to look for their utilization methods. Among the possibilities, particular attention should be drawn to polymer technology. This sector can efficiently use different lignocellulosic materials, which could be applied as fillers for polymer composites or sources of particular chemical compounds. Moreover, due to their chemical composition, brewing industry by-products may be used as functional fillers and additives. They could be introduced to enhance the materials’ resistance to oxidation, microbes, or fungi. These issues should be considered especially important in the case of biodegradable polymers, whose popularity is growing over the last years. This paper summarizes the literature reports related to the composition and potential applications of the brewing industry by-products in polymer technology. Moreover, potential directions of research based on the possibilities offered by the brewing industry by-products are presented.
Similar content being viewed by others

Aldehydes as Wort Off-Flavours in Alcohol-Free Beers—Origin and Control
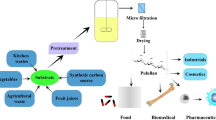
Microbial pullulan for food, biomedicine, cosmetic, and water treatment: a review
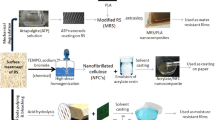
Lignocellulosic Agricultural Biomass as a Biodegradable and Eco-friendly Alternative for Polymer-Based Food Packaging
Avoid common mistakes on your manuscript.
1 Introduction
As a process aimed at beer production, brewing is known for thousands of years [ 1 ]. Depending on the times and mainly the development of humanity and science, the specifics of this process were changing [ 2 ]. Figure 1 presents the current general scheme of beer production. Generally, the brewing process consists of the following stages, starting from the malt: crushing of malt, mashing, lautering, filtration, boiling, and fermentation. At first, malt is crushed to break apart the kernel and facilitate the extraction of sugars during mashing [ 3 ].
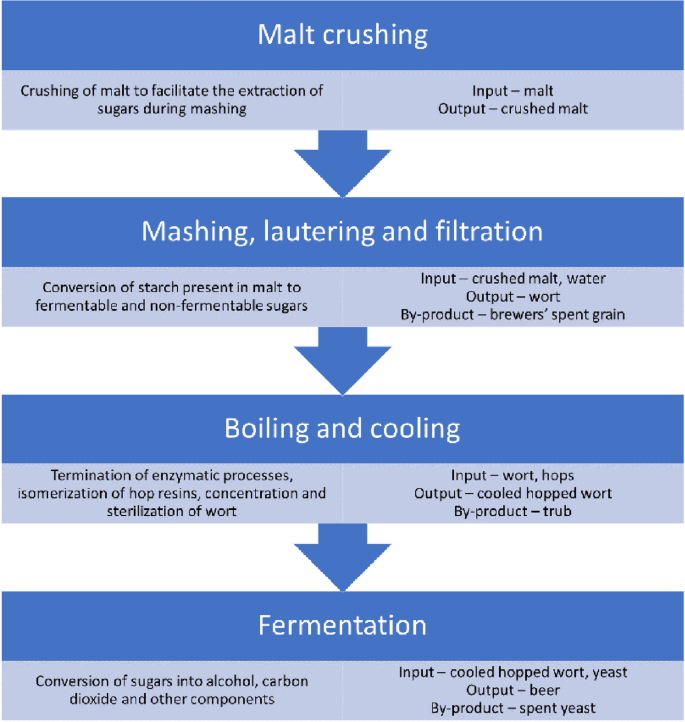
The general scheme of beer production with an indication of generated by-products
Then, crushed malt is mixed with hot water in a mash tun creating the cereal mash. Naturally occurring enzymes present in the malt convert the starch extracted from the malt into simpler fermentable and non-fermentable sugars in the saccharification process [ 4 ]. To enhance the yield of mashing, in the end, the mas is often heated up to 76–78 °C, which is called mashout, and sprinkled with additional water during sparging [ 5 ]. Such processes are implemented to reduce mash viscosity, free up more starch, and extract additional sugars [ 6 ]. As a result, a sugar-rich liquid called wort is obtained after separation from the solid residue of mashing called the brewers’ spent grain (BSG) [ 7 ].
The wort is moved to the kettle, where it is boiled with hops (and sometimes other ingredients). The boiling process aims to terminate the enzymatic processes, precipitate proteins, concentrate and sterilize the wort, as well as extract compounds from hops to beer and isomerize hop resins to add bitterness to beer [ 8 ]. Moreover, boiling may induce caramelization and Maillard reactions in wort [ 9 ]. In the end, the hopped wort is cooled down and separated from the trub, which includes the hop residues and colloidal proteins coagulated during boiling [ 10 ].
Cooled and hopped wort is transferred to the fermentation tanks and pitched with yeast, converting the fermentable sugars into alcohol and carbon dioxide. Moreover, depending on the type of yeast, different reactions occur, which account for the beer’s final taste and aroma profile [ 11 ]. After complete fermentation, the beer is separated from the surplus yeast, called spent yeast, conditioned in the additional tank, and packed into bottles, casks, or cans [ 12 ].
2 Beer production statistics
Beer is the most popular alcoholic beverage globally and the third most popular beverage after water and tea [ 13 ]. The global beer production size is relatively stable in the last decade and accounts for 1.91–1.97 billion hectoliters [ 13 ]. The leading producer is China, whose production in 2019 equaled 376.5 million hectoliters and accounted for ~ 20% of global production [ 14 ]. The Americas occupy the following positions—the United States, Brazil, and Mexico with 210.3, 144.8, and 124.2 million hectoliters, respectively [ 15 ]. Considering Europe, the size of production exceeds 420 million hectoliters annually [ 16 ]. The share of particular continents in the global beer production is presented in Fig. 2 . The biggest producer is Germany, the country with great brewing traditions. In 2019, Germans produced 91.6 million hectoliters of beer, mostly pilsener and wheat beer [ 17 ]. In Europe, Germany is followed by Poland and the UK, whose production is around 40 million hectoliters [ 18 ]. It is also essential to mention Russia as a critical producer with 77.4 million hectoliters produced in 2019 [ 19 ]. Russia is one of the few countries where beer is not the most popular alcoholic beverage, with a higher vodka share [ 20 ]. Presented data indicate that global beer production is distributed across all the regions of the world. Therefore, research activities related to the manufacturing, consumption, and health aspects and the utilization of by-products of beer production are essential because these aspects may affect an enormous number of people worldwide.
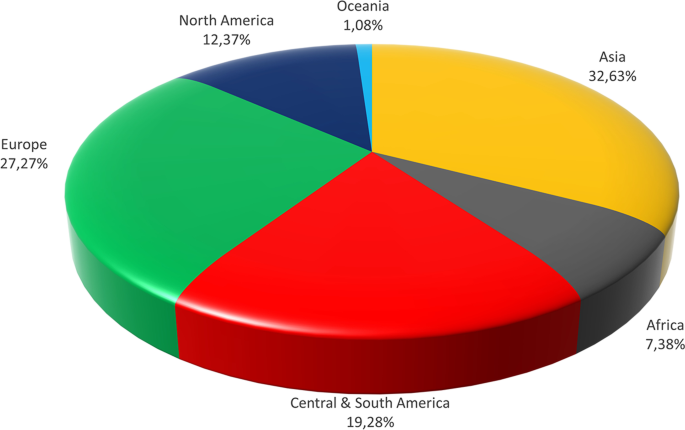
The share of particular continents in the global beer production
3 Beer production by-products
Conventionally, beer is produced from barley and, to a noticeably lesser extent, from wheat [ 21 ]. Such a production model is commonly applied in Europe. Moreover, in Germany, such issues are regulated by Germany’s Beer Purity Law, originating from the medieval Bayreisches Reinheitsgebot [ 22 ]. Nevertheless, in different regions of the world, other starch sources are also applied, such as maize in America [ 23 ], rice in Asia [ 24 ], or sorghum in Africa [ 25 ]. Considering hops and yeast, their use is associated with the desired beer style. The market offers an enormous range of hops and yeast varieties [ 26 , 27 ].
According to the brewing scheme presented in Fig. 1 , the main by-products of beer manufacturing are the brewers’ spent grain, trub removed after wort boiling, and spent yeast. The generation of brewing by-products is very similar globally, with possible differences in their composition, depending on the location. These materials are currently often unutilized and present hardly any market value. Generally, considering the current pro-environmental trends in scientific and industrial activities partially stimulated by the changing law regulations established worldwide, waste and by-products management are essential [ 28 ]. Except for the environmental motivations, the application of such materials in various production processes can generate added value for the resulting products (enhanced performance or new properties) or reduce their manufacturing costs [ 29 ].
One of the industry branches where by-products from beer manufacturing could be potentially applied in polymer technology. This sector of the industry is enormously dependent on petroleum price and availability [ 30 ]. It is commonly known that its resources are constantly shrinking, and it is essential to seek new raw materials, which could substitute petroleum, beneficially from renewable resources [ 31 ]. In the following sections, the literature reports on the applications of brewing by-products in polymer technology would be discussed.
3.1 Brewers’ spent grain
3.1.1 overview.
The main and the most abundant by-product generated by the brewing industry is brewers’ spent grain. It is generated in high amounts and accounts for over 85% of beer manufacturing by-products [ 32 ]. During the mashing process, around 69% of the initial malt mass is extracted and converted to sugars soluble in wort [ 33 ]. Considering that production of the one hectoliter of beer uses 20 kg of malt, around 6.2 kg of dry BSG is generated. Such values are typical for the most popular beer style worldwide—light lager [ 34 ]. Other beer styles, especially those characterized by higher original gravity, require higher malt loadings, even up to 45 kg for strong stouts or porters [ 35 ]. Keeping in mind the size of the global beer production, almost 12 million tonnes of brewers’ spent grain is generated worldwide. The biggest producer, China, accounts for over 2.3 million tonnes, followed by the USA with 1.3 million tonnes, while European production generates around 2.6 million tonnes of BSG [ 34 ].
3.1.2 Composition
One of the main drawbacks of BSG as a potential raw material for other processes is its high moisture content, exceeding 75% [ 36 ]. Together with the presence of polysaccharides, this factor makes the BSG a perishable material. Drying of brewers’ spent grain, which could be easily performed using conventional dryers, might noticeably enhance its attractiveness for other industry branches, including polymer technology [ 37 ]. Moreover, BSG is characterized by the relatively low activation energy values during drying, comparable to other food industry by-products such as carrots, beans, or general vegetable waste [ 38 ]. The drying of BSG requires less energy than, e.g., olive processing by-products, probably due to their high lipid content [ 38 ].
The brewers’ spent grain can be characterized by a similar composition to various lignocellulose materials except for the high moisture content. As presented in Table 1 , the total content of carbohydrates in BSG is around 50%, which is noticeably lower compared to other lignocellulose waste materials, e.g., barley straw (56%) [ 39 ], rye, or oat straw (66–68%) [ 40 ], sunflower or cotton stalks (72–73%) [ 40 ]. Such a phenomenon is attributed to the partial reduction of carbohydrate content during mashing when starch is removed [ 41 ].
Moreover, brewers’ spent grain contains from 10 to even 28% of lignin, depending on the reports [ 48 ] and a significant amount of proteins, which is attributed to their high content in barley grain [ 52 ]. The detailed composition of proteins in BSG may differ depending on the determination method, source of by-product, and applied malts, mostly crop species [ 33 ]. According to Robertson et al. [ 53 ], the glutamine is the primary amino acid of BSG (around 19–20% of total proteins), followed by a proline (~ 9%), asparagine, and leucine (both ~ 8%), arginine, phenylalanine, and valine (~ 7%). On the other hand, Waters et al. [ 54 ] reported that histidine is present in the highest amount exceeding 26% of total proteins, followed by glutamine (~ 16%), lysine (~ 14%), and leucine (~ 6%), while the content of other amino acids does not exceed 5%. Nevertheless, irrespectively of the detailed composition of amino acids, BSG should be considered as protein-rich material.
Brewers’ spent grain often contains noticeable amounts of phenolics, which may provide additional value for various applications due to their antioxidant and antimicrobial properties [ 55 ]. The main phenolic components of BSG are hydroxycinnamic acids (HCAs) and hydroxybenzoic acids (HBAs), particularly ferulic, p -coumaric, sinapic, syringic, and caffeic acids [ 56 , 57 , 58 ]. The first two compounds are present in the highest amounts, but the literature reports indicate that their contents depend strongly on the type of malts used for brewing [ 59 ]. McCarthy et al. [ 59 ] showed that the roasting of pale barley malt reduced the total HCAs content in BSG by 57%. Also, Moreira et al. [ 60 ] pointed to the reduction in total phenolic content due to increasing malt kilning temperature. For chocolate and black malts, kilned at temperatures exceeding 220 °C, the content of ferulic and p -coumaric acids was reduced by over 50%. The effect was significantly smaller for melanoidin and carared malts, which were subjected to a temperature in the range of 120–160 °C.
Generally, the structures of the major phenolic acids present in brewers’ spent grain are presented in Fig. 3 . Their detailed composition in particular BSG samples and other by-products, may noticeably differ depending on the method of their extraction, selection of solvents, and method of the quantitative analysis [ 35 ]. Nevertheless, despite the differences in reported contents of HCAs and other phenolics, they significantly enhance the antioxidant activity of BSG compared to other lignocellulose materials.
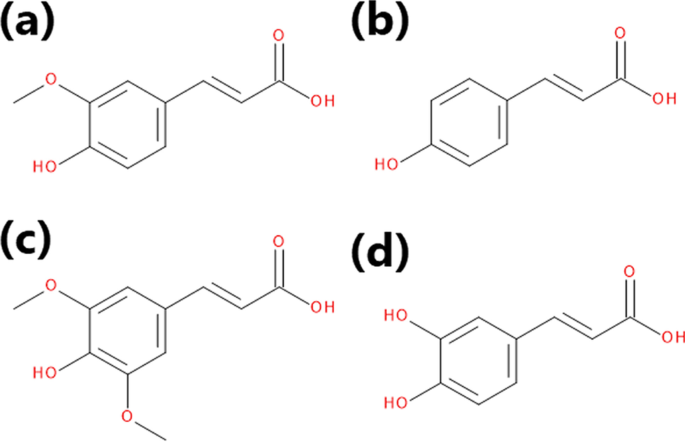
The structures of the major phenolics of BSG: a ferulic, b p -coumaric, c sinapic, and d caffeic acid
These compounds mentioned above present in BSG are considered strong antioxidants and may enhance the stability of polymeric materials [ 61 , 62 , 63 ]. Considering the antioxidant activity of BSG, it may contain noticeable amounts of melanoidins, mainly when it originated from the production of darker beers. Melanoidins are generated during Maillard reactions occurring between carbonyl groups of reducing sugars and amino groups of amino acids present in proteins [ 64 ]. As a result, the complex mixture of higher molecular weight oligomeric and polymeric compounds is obtained [ 65 ]. They are responsible for the browning reactions of various food products after applying temperature, e.g., during baking, frying, or cooking [ 66 ].
Moreover, the brewers’ spent grain contains multiple micro- and macroelements, mostly silicon, phosphorous, calcium, and magnesium [ 32 ]. Combining their content with the presence of vitamins, primarily B3, B4, and B5, the BSG is often investigated in food additives [ 48 , 67 ].
3.1.3 Current applications and potential in polymer technology
Animal feed.
Nowadays, the main application of brewers’ spent grain is low-value animal feed with relatively low market value. It is often sold to farmers, mainly in a wet state [ 68 ]. It is associated with the composition of this by-product, particularly protein content [ 45 ]. As a result, Belibasakis and Tsirgogianni [ 69 ] and Sawadogo et al. [ 70 ] reported the enhanced milk production for cows fed with BSG. Also, other works reported the beneficial impact on animal nutrition, including fish, pigs, and chickens [ 71 , 72 , 73 ]. In the case of lack of potential recipients of BSG for animal feed, this by-product may be deposited in the fields, where in moderate amounts, it can act as natural fertilizer [ 55 ].
Considering the nutrition, brewers’ spent grain was also investigated as a human food ingredient. Because of its composition and origin, it was introduced into bakery products as a flour substitute [ 74 ]. Ground BSG is characterized by a darker color than the lightest types of flour, so it could not be applied in white bread [ 75 ]. Nevertheless, BSG showed a very beneficial impact on the bread protein content due to its composition, increasing it by ~ 50%, when only 10% of traditional flour was replaced [ 76 ]. Combining the protein content with the lack of starch (which is removed during mashing—see Fig. 1 ), the caloric density of bread containing 10% of BSG may be even 7% lower compared to the conventional bread [ 77 ]. Except for the bakery products, brewers’ spent grain can be incorporated into other high-fiber foods [ 78 , 79 , 80 , 81 ]. In general, the food-sector applications of brewers’ spent grain were comprehensively discussed in the excellent review works of McCarthy et al. [ 82 ], Lynch et al. [ 48 ], and lately Rachwał et al. [ 83 ].
Energy production
Like other types of waste biomass, BSG was also investigated in energy production, which may be implemented within the brewery, leading to reduced production costs [ 84 ]. It can be directly combusted. However, it may cause problems related to the high nitrogen content and resulting generation of nitrogen oxides [ 85 ]. Such an effect can be reduced by the application of pyrolysis [ 86 ]. Another possibility is converting BSG into charcoal bricks, which increases its calorific value from ~ 20 to 27 MJ/kg [ 87 , 88 ]. Multiple works also reported microbial fermentation of brewers’ spent grain into bioethanol [ 89 , 90 , 91 ] or biogas [ 92 , 93 , 94 , 95 ], which can be applied as biofuels.
Fermentation
Considering fermentation, BSG was investigated as a growth medium [ 96 ], e.g., substitute of sucrose or glucose in the lactic fermentation [ 97 , 98 , 99 ], medium for pullulan production [ 100 ], and introduced into manufacturing of xylitol [ 101 , 102 , 103 ] or citric acid [ 104 ]. The application of wastes and residues for fermentation is a very common and often investigated approach [ 105 ].
Among the mentioned fermentation products, lactic acid is an exciting compound for polymer technology. It is commonly applied in poly(lactic acid) manufacturing—one of the most popular biodegradable, thermoplastic polyesters [ 106 ]. Over the last years, it attracted much attention due to the application in 3D printing [ 107 ]. Moreover, due to the current law regulations, its popularity in manufacturing packaging materials is increasing, often combined with other, less expensive materials like thermoplastic starch [ 108 ].
Except for lactic acid, other BSG fermentation products, which could be applied in polymer technology are citric acid [ 109 ] and propionic acid [ 110 ]. The first one can be applied as a co-monomer in manufacturing of polyesters [ 111 , 112 , 113 , 114 ] or as a crosslinking agent [ 115 , 116 ]. Propionic acid is a substrate in the production of polymers based on cellulose derivatives, such as cellulose propionate [ 117 ]. Their popularity is growing over the last years due to the current pro-environmental trends related to bio-based polymer materials and their potential novel applications, e.g., in 3D printing [ 118 , 119 , 120 ].
Figure 4 summarizes the potential applications of BSG fermentation products in polymer technology. Nevertheless, to the best of our knowledge, no works deal with the application of BSG fermentation products in polymer technology. More details related to the recent developments in the biotechnological valorization of these by-products were presented recently in the comprehensive review work by Puligundla and Mok [ 121 ].
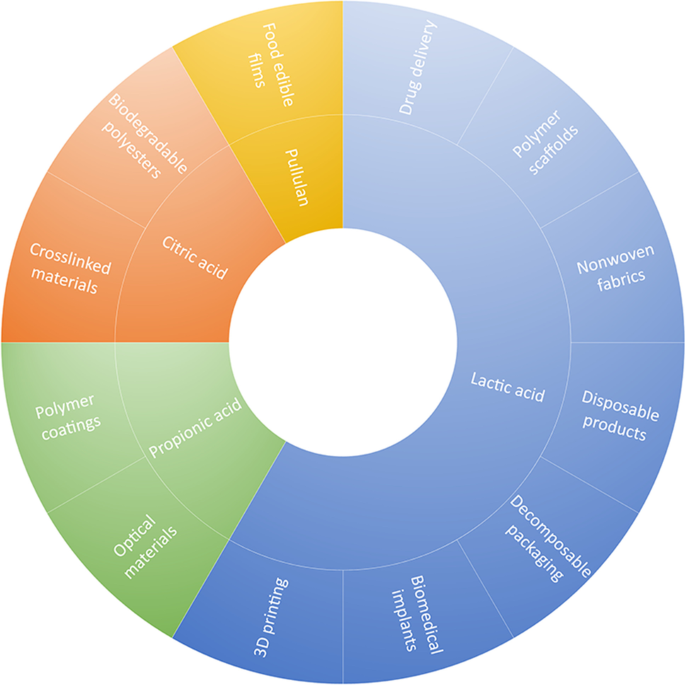
Potential applications of BSG fermentation products in polymer technology
Extraction of celluloses and lignin
Except for the microbial conversion, various compounds present in BSG, which may find application in polymer technology, can be extracted using different techniques [ 122 , 123 , 124 ]. Among the most noticeable components of BSG in terms of polymer technology are celluloses and lignin [ 125 ]. They could be applied as fillers for composites and intermediates in manufacturing other raw materials used in polymer technology.
Mishra et al. [ 126 ] presented the multi-stage process of BSG conversion into cellulose nano-fibers consisting of alkali treatments and bleaching. They isolated nanosized fibers with an average diameter of 4.6 nm, shown by atomic force microscopy. Similar fibers are often investigated as fillers for polymer nanocomposites [ 127 , 128 , 129 ]. The efficient isolation of fibers and removal of other components of BSG was confirmed by thermogravimetric analysis (lack of decomposition step attributed to the presence of lignin) and X-ray diffractometry (gradual increase in crystallinity index). Similar observations were made by dos Santos et al. [ 130 ].
Mussatto et al. [ 131 ] investigated the recovery of lignin from BSG. The by-product was subjected to the soda pulping process, comprehensively described in other work [ 132 ]. The resulting black liquor was treated with sulfuric acid, which enabled the separation of lignin by precipitation. Except for the lignin, the investigated process enabled the removal of ferulic, p -coumaric, p -hydroxybenzoic, vanillic, and syringic acids from black liquor. Such an effect points to the presence of these compounds in obtained lignin, which could be applied, e.g., as a filler for wood-polymer composites.
Quite interesting is also the extraction of arabinoxylans from brewers’ spent grain. They are hemicelluloses found in plants' primary and secondary cell walls, which consist of copolymers of arabinose and xylose [ 133 ]. They play a structural role in plants, and interestingly the significant amounts of phenolic acids are bound to them, so they show noticeable antioxidant activity [ 134 ]. Arabinoxylans and their esters are applied in polymer technology to develop biodegradable and even edible films [ 135 , 136 ], which could be efficiently applied in food packaging applications [ 137 , 138 , 139 ]. They could be enzymatically extracted from BSG in a multi-step procedure [ 140 , 141 , 142 ]. The extraction efficiency can be significantly enhanced by the proper particle size distribution of BSG adjusted by milling and sieving, as proven by Reis et al. [ 143 ]. Moreirinha et al. [ 144 ] used the brewers’ spent grain arabinoxylans to prepare composite films with a different share of nanocellulose. Moreover, selected composite films were modified with ferulic acid or feruloylated arabinoxylo-oligosaccharides obtained from BSG. All prepared films were characterized by the onset of thermal decomposition exceeding typical sterilization or autoclaving temperatures ~ 150 °C. They were characterized by satisfactory mechanical performance, with Young’s modulus in the range of 4.3–7.5 GPa (the highest for composite containing 50% of nanocellulose) and tensile strength of 71–110 MPa (the highest for 75% nanocellulose loading). Modifications of films unfavorably affected the mechanical performance but increased the antioxidant activity determined by DPPH assay from the initial 2% to 64–90%. Also, a noticeable reduction in bacterial ( Staphylococcus aureus ) and fungal ( Candida albicans ) growth is beneficial for the potential applications in food packaging.
Extraction of phenolics
Except for cellulose, hemicellulose, or lignin, also other compounds can be extracted and isolated from brewers’ spent grain. Very auspicious are the phenolics mentioned above, primarily hydroxycinnamic acids, showing antioxidant properties. The BSG should be considered an inexpensive source of these compounds, which could be efficiently applied not only in the food or cosmetic industry [ 145 , 146 , 147 ] but also as potential antioxidants for polymer materials [ 148 , 149 , 150 ]. Phenolics can be recovered from brewers’ spent grain by different variants of extraction, e.g., solid–liquid type or assisted by supercritical fluids, microwaves or ultrasounds, and enzymatic or alkaline reactions [ 151 , 152 , 153 ]. As mentioned above, the yield of their recovery strongly depends on the extraction method and its parameters, e.g., selected solvents [ 154 ]. Similar phenomena were reported for other by-products, e.g., from the coffee industry, also characterized by high phenolics content [ 155 , 156 , 157 ]. The recent advances in the extraction of bioactive compounds, including phenolics, from brewers’ spent grain, were comprehensively summarized in the excellent work of Bonifácio-Lopes et al. [ 158 ]. Nevertheless, to the best of our knowledge, there are no literature works reporting the application of BSG-originated phenolics in polymer materials.
Liquefaction
Considering other, more direct uses of brewers’ spent grain in polymer technology, in our previous work [ 159 ], we used the brewers’ spent grain as a biomass feedstock for crude glycerol-based microwave liquefaction aimed at manufacturing biopolyols for polyurethanes. The general scheme of the process is presented in Fig. 5 . The spectroscopic investigation of obtained polyols revealed that biomass was partially decomposed during the process and reacted with solvent particles. Nevertheless, the efficiency of the process was relatively low, and the hydroxyl values were in the range of 900–1050 mg KOH/g. Such values are very high and could be suitable only for manufacturing very crosslinked and stiff materials. Further studies should include the application of catalysts and optimization of process conditions to enhance its efficiency.
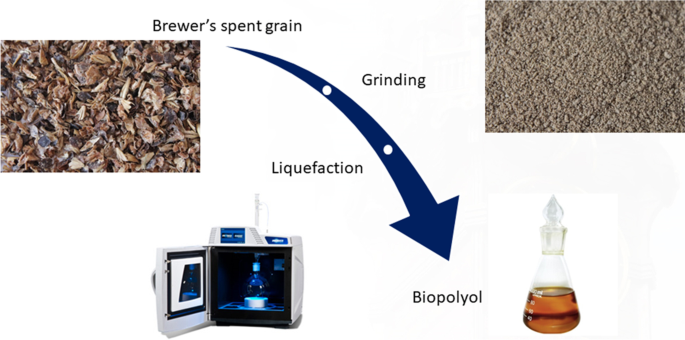
The general scheme of microwave-assisted liquefaction of BSG resulting in biopolyol for polyurethanes [ 159 ]
Filler for polymer composites
On the other hand, the relatively high fiber content in brewers’ spent grain makes it attractive for the preparation of wood-polymer composites, possibly applied in various branches of industry [ 160 ]. Other fillers, either conventionally applied wood flour or waste-based, are characterized by a relatively similar composition [ 161 , 162 ]. Moreover, the presence of proteins, which may act as plasticizers, may facilitate the melt processing of composites [ 163 ].
Revert et al. [ 164 ] introduced the BSG as a filler into the polypropylene matrix. Nevertheless, due to the polarity differences between filler and matrix, the interfacial interactions were fragile, and the significant deterioration of mechanical performance was noted. The only positive effect of the BSG incorporation was the shift of the oxidation onset temperature attributed to the presence of phenolics showing antioxidant activity. The addition of 10–40 wt% of by-product shifted the oxidation onset by 10–23 °C.
Barbu et al. [ 165 ] introduced BSG into particleboards as a partial replacement of wood, which is a common trend in the case of wood-polymer composites [ 166 , 167 , 168 ]. The appearance of BSG without additional treatment (presented in Fig. 6 ) is quite similar to the wood chips, so this by-product can be easily applied as substitute for conventional raw materials. Particleboards bonded with polymeric 4,4′-methylene diphenyl isocyanate, urea–formaldehyde, or melamine urea–formaldehyde resin. Nevertheless, according to the presented results, to maintain the satisfactory level of the mechanical properties, the content of BSG should be kept at low levels (up to 10%). The structural differences associated with the reduction in particle–particle bonding between wood particles were noted at higher loadings due to the high consumption of glue by BSG. Authors suggested that at higher contents, brewers’ spent grain should be combined with innovative glues, based on casein or tannins, which could improve the particle–particle bonding. Similar conclusions about the potential, beneficial share of BSG in wood-based particleboards were provided by Klimek et al. [ 169 ], who also suggested the 10% substitution of wood with BSG.
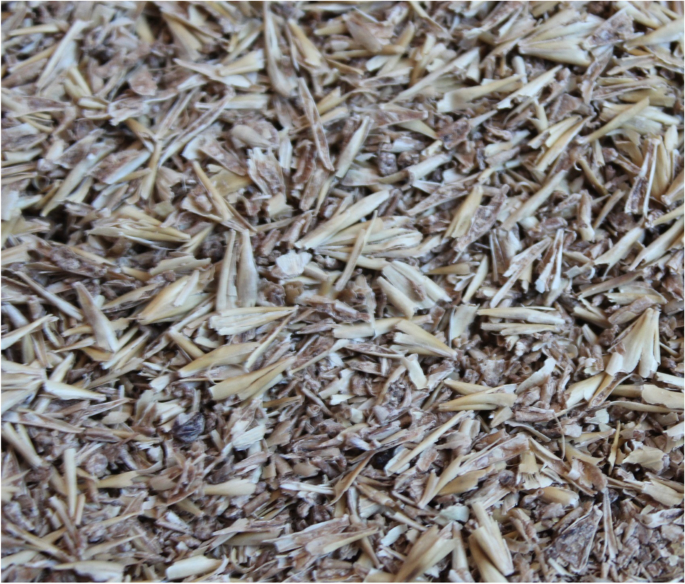
The appearance of BSG without additional treatment
A very interesting application of BSG in polymer technology was also presented by Ferreira et al. [ 170 ], who used it to prepare disposable trays for food packaging. They were obtained by compression molding of BSG with potato starch applied as a continuous phase. The process was aided with the addition of glycerol, water, and in some cases gelatin, chitosan, or glyoxal applied as crosslinkers. After compression, trays were covered with beeswax. Obtained materials were characterized by significantly higher flexural strength and modulus than commercial trays from expanded polystyrene. Independently of the BSG content (from 20 to 80% in the whole tray), trays showed strength in the range of 1.51–2.62 MPa, compared to the 0.64 MPa for commercial material. Also, the modulus was noticeably higher (1.1–2.0 MPa) compared to polystyrene trays (0.28 MPa). Nevertheless, due to their composition and hydrophilic character of applied raw materials, trays showed significantly higher water absorption than polystyrene, which resulted in a significant 80–90% decrease in mechanical parameters. Such an effect was attributed to the plasticizing effect of water. The application of crosslinkers or beeswax slightly reduced the water absorption. However, deterioration was still noted after immersion, and polystyrene trays showed noticeably superior properties. Nevertheless, presented results indicate that such a solution is quite promising, especially for applications that do not require exceptional resistance to water.
Generally, multiple works dealing with the application of brewers’ spent grain in the polymer sector, especially these dealing with its use as a filler for composites or substitute of wood in particleboards, indicate the necessity for modifying this by-product [ 171 , 172 ]. Modifications should be aimed at enhancing the interfacial interactions with polymer matrices by the change of their hydrophilic character. Such an effect could be achieved by thermo-mechanical treatment resulting in non-enzymatic browning in the caramelization process and mostly Maillard reactions, as presented in our previous works [ 34 , 173 ]. It is also essential that melanoidins show noticeable antioxidant activity, which can be very beneficial for the oxidative stability of polymer composites [ 174 ].
In previous works, we reported the application of thermo-mechanically modified BSG as a potential filler for wood-polymer composites [ 175 , 176 ].
In the case of poly(ε-caprolactone) composites, their mechanical performance was noticeably affected by the parameters of thermo-mechanical treatment of BSG [ 175 ]. The increase of modification temperature noticeably affected the interface surface area, resulting in an even 30% increase in composites’ toughness. Dynamic mechanical analysis indicated over 30% drop in the adhesion factor and increased the constrained chain volume exceeding 27%. Such an effect points to the significant enhancement of the interfacial interactions.
On the other hand, modified BSG could be effectively applied to substitute traditional wood flour in polyethylene-based composites [ 176 ]. Nevertheless, due to the differences in the chemical composition between fillers (presence of proteins, lipids, and melanoidins in BSG, lower content of holocellulose compared to wood flour), the composites’ performance was differing. The introduction of BSG resulted in significantly higher values of melt flow index. When wood flour was completely substituted, it increased from 3.23 to 10.56 g/10 min, which was attributed to the drop in viscosity from 1.02 to 0.30 Pa s. A similar effect was noted in our other work [ 163 ]. The density and porosity of materials were slightly reduced with the increasing share of BSG due to the presence of proteins, which may enable better encapsulation of filler particles with polymer macromolecules. However, it also caused changes in the composites’ mechanical performance. Tensile strength and modulus were reduced by ~ 27% and ~ 42%, respectively, but the elongation at break was doubled. Concluding, modified brewers’ spent grain could be introduced as partial replacement of conventional wood flour to engineer materials with desired properties.
Other, less popular methods of BSG utilization include the manufacturing of adsorbents [ 177 , 178 , 179 ], paper [ 47 , 180 ], bricks [ 87 ], or activated carbon [ 181 ]. Brewers’ spent grain-based adsorbents, and activated carbons were found efficient in the removal of dyes [ 182 , 183 , 184 , 185 ], volatile organic compounds from the gas phase [ 186 ], but mostly metal ions from aqueous media [ 187 , 188 , 189 , 190 , 191 ].
3.2 Trub, spent hops
3.2.1 overview.
Trub is generated during filtration of wort before the fermentation. After cooling down, the wort is separated from the trub, accounting for around 0.2–0.4% of the wort volume [ 28 ]. The precipitate contains coagulates of high molecular weight proteins [ 192 ]. They are generated during the heat-induced denaturation of proteins. Except for coagulates, the residual hops may be present in trub, because according to the literature data, around 85% of the initial hops mass introduced into wort is removed as a by-product [ 77 ]. After extracting the compounds that create the flavor, aroma, and bitterness of the final beer, the solid residues of hops are discarded. However, their content in trub depends on the applied technology of beer hopping [ 193 ]. Therefore, the composition of this by-product may be very diverse, depending on the share of coagulates and spent hops.
3.2.2 Composition
Separately, trub contains mostly significant amounts of proteins, even up to 70% of dry matter [ 83 ]. Considering spent hops, the proteins account for around 22–23% of dry matter, with a similar share of fiber [ 194 ]. According to Mathias et al. [ 42 ], around 20% of the residual reducing sugars may also be present in trub. Both trub and spent hops contain relatively low amounts of ash, ~ 2%, and ~ 6%, respectively. Interestingly, this by-product may contain noticeable amounts of essential oils and phenolics due to the hop presence. Such an effect is attributed to the low solubility of various compounds in the wort, e.g., in the case of lupulones [ 195 ]. On the other hand, phenolics such as hydroxycinnamic acids, gallic acid, catechins, anthocyanidines, and others, are precipitated with proteins and removed as a part of hot trub [ 196 ]. The essential oils contain noticeable amounts of terpenes [ 67 ]. Moreover, myrcene, alpha-humulene, or beta-ceryophyllene are present. They account for ~ 47% of the essential oil [ 197 ]. The structures of main components of essential oils are presented in Fig. 7 .
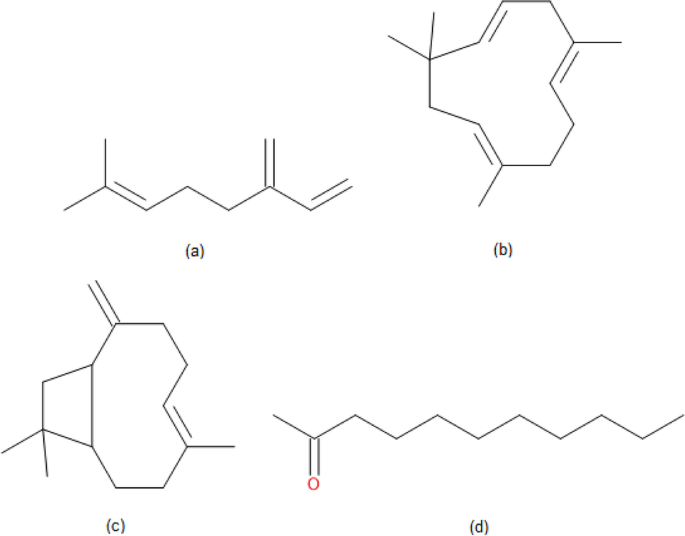
The main components of spent hops essential oil: a myrcene, b α-humulene, c β-caryophyllene, and d 2-undecanone [ 197 ]
3.2.3 Current applications and potential in polymer technology
Contrary to the brewers’ spent grain, the trub and spent hops are significantly less frequently used in animal feeding, despite the beneficial composition, relatively high fiber content, and mostly the presence of proteins. Such an effect is attributed to the presence of hops, particularly bitterness [ 198 ]. Another issue is the low energy value of spent hops, which is around 50% lower than spent grains, despite the relatively high fiber content [ 77 ]. On the other hand, some research works investigated the combination of spent hops with other brewery by-products, which resulted in acceptable feed, e.g., for pigs [ 199 ]. According to the works of Huszcza et al. [ 200 , 201 ], the bitter acids originated from spent hops can be removed or degraded by yeast or fungi. More recent works dealing with the trub and spent hops investigated the reduction of bitterness by the multi-step extraction of bitter compounds [ 202 ]. During extraction, most carbohydrates were removed together with tannins and phenolics, which are often bound to the carbohydrates. As a result, the share of proteins and fat was increased from the initial 26.5% and 5.2% to 70.3% and 9.9%, respectively. Due to the significant drop of phenolics content (from ~ 350 to ~ 125 mg gallic acid equivalents/100 g), the antioxidant activity was also noticeably reduced. Nevertheless, changes resulting from the extraction allowed the use of modified trub in food applications.
Considering the most popular uses of biomass and brewery by-products, except for the animal feed, trub and spent hops may be applied as a soil conditioner and fertilizer, which is associated with their high nitrogen content [ 42 , 203 ].
As mentioned above, the trub and spent hops contain noticeable amounts of essential oils and phenolics. These compounds can be effectively extracted from this by-product and potentially applied in polymer technology. Bedini et al. [ 197 ] reported that the terpenes present in spent hops could be applied as the repellents against the insects, e.g., Rhyzopertha dominica or Sitophilus granarius . Spent hops essential oils were 2–5 times more effective against R. dominica than Laurus nobilis oils [ 204 ] and showed similar repellency against S. granaries as Hyptis suaveolens oils [ 205 ]. Bartmańska et al. [ 206 ] also showed antifungal activity of spent hops extracts against Botrytis cinerea , Fusarium oxysporum , Fusarium culmorum , and Fusarium semitectum . Therefore, the application of spent hops or only their essential oils in manufacturing the packaging materials seems reasonable. The extraction and isolation of essential oils from spent hops can be performed by hydrolysis, steam distillation, but also using supercritical CO 2 or eutectic solvents [ 207 , 208 , 209 , 210 ].
Moreover, this by-product can be applied in fermentation processes as a supplement for microbes due to the high nitrogen content [ 83 ] and the presence of lipids and zinc [ 211 ].
3.3 Spent yeast
3.3.1 overview.
During the initial stage of fermentation, the intensive proliferation of yeast is occurring, so after fermentation and maturation, the surplus of yeast is present and should be removed from beer [ 212 ]. Partially, they are recovered by natural sedimentation, but for higher efficiency, additional filtration or centrifugation may be applied [ 213 ]. The actual amount of this by-product depends on the type of yeast used for fermentation, but it can account even for 15% of total by-products generated during beer production [ 214 ]. Around 0.3 kg of spent yeast is generated per hectoliter of beer. Depending on the properties of yeast, in particular their flocculation, this by-product is characterized by the high moisture content in the range of 75–90%. Therefore, portion of beer is removed along with yeast, which may generate the 1.5–3.0% loss of beer [ 28 ].
3.4 Composition
Considering the composition, spent yeast contains a noticeable amount of carbohydrates and proteins. Among the first group, the most abundant are non-cellulose compounds (25–35%), mainly β-glucans, mannoproteins, and glycogen, followed by cellulose (17–25%) [ 215 ]. Proteins may account for more than 50% of the spent yeast dry mass [ 195 , 216 ]. Jacob et al. [ 217 ] reported even value of 74.3 wt%. Therefore, the spent yeast is characterized by the lowest carbon:nitrogen ratio among the brewing by-products, around 5 [ 42 ]. Literature works report different amino acid compositions of spent yeast. According to Vieira et al. [ 218 ], the most common amino acids in spent yeast are alanine (even over 9%), arginine, aspartic acid, and cysteine. At the same time, Jaeger et al. [ 219 ] indicated that glutamic acid is the most abundant one (~ 15%), followed by histidine, alanine, arginine, and aspartic acid. Such an effect may be associated with the differences between particular Saccharomyces cerevisae strains applied worldwide [ 220 ].
Brewery spent yeast are also a great source of micro- and macroelements, as well as vitamins. Nevertheless, the mineral composition of yeast can differ depending on the length of fermentation and fermentation cycles [ 67 ]. Among the most abundant minerals are sodium, potassium, and magnesium, but noticeable amounts of phosphorous may be present, which could be associated with yeast nutrients in breweries [ 195 ]. Considering the vitamins, the B type is the most popular, especially niacin, thiamin, pantothenate, and riboflavin [ 218 ].
3.4.1 Current applications and potential in polymer technology
Due to the high content of proteins, vitamins, and minerals, the spent yeast is mostly applied as an additive in animal feeding [ 221 ]. It has been repeatedly proven that such application of this by-product shows very positive effects on the animals’ health. It may result in increased milk production, improved microflora, and enhanced resistance to various microorganisms [ 222 , 223 , 224 ]. Except for the animal feed, spent yeast was also investigated in human consumption. However, they show great potential due to the high contents of nucleic acids (6–15%), in particular ribonucleic acid, their use in an unmodified state is somewhat limited [ 216 ]. The ribonucleic acid is metabolized to the uric acid in the human body, which can cause gout [ 225 ]. Generally, the spent yeast application in animal and human nutrition was comprehensively discussed in dedicated review works [ 219 , 226 , 227 , 228 , 229 ].
Another broadly investigated application of spent yeast is cultivating microorganisms and other biotechnological processes [ 230 , 231 ]. Due to the beneficial composition of brewery spent yeast, various microorganisms cultivated on this by-product may grow noticeably faster than other yeast types, as reported by Ferreira et al. [ 232 ]. As a result, the spent yeast may be applied as a very efficient source of multiple enzymes, including proteinases [ 233 ], proteases [ 234 , 235 ], or pectinases [ 236 , 237 ]. Moreover, brewery spent yeast can be applied as nutrients during ethanol or lactic acid fermentation [ 238 , 239 ]. Pietrzak and Kawa-Rygielska [ 240 ] showed that the 5% addition of brewery spent yeast might increase the rate of sugar consumption and ethanol production resulting in even 11% enhancement of ethanol yield. Such an effect was ascribed to the high nitrogen content of the medium.
Other, less popular uses of spent yeast from beer production include methane production or application as biosorbent. Considering the energy production, spent yeast can be considered an excellent substrate for co-digestion purposes with other materials, including swine manure [ 241 ], spent grains [ 242 ], or crude glycerol [ 243 ].
Considering the polymer technology point of view, the brewery spent yeast show hardly any applications. The most promising and the closest to polymer technology is their use in fermentation processes generating lactic or succinic acid. The use of lactic acid in polymer technology was described in Sect. 3.1.3 . The succinic acid can be applied in the manufacturing of polyesters or alkyd resins [ 244 , 245 ].
Rakin et al. [ 246 ] reported that the application of spent yeast might increase the rate and yield of lactic acid fermentation due to the presence of amino acids, vitamins, and minerals. Similar observations were made by Champagne et al. [ 247 ]. According to Radosavljević et al. [ 248 ], the brewery spent yeast, combined even with other brewery by-products, can act as a low-cost fermentation medium for manufacturing of lactic acid.
Considering succinic acid production, the substitution of conventional yeast extracts with hydrolysate of spent brewery yeast as a nitrogen source was successfully achieved by Jiang et al. [ 249 ] and Chen et al. [ 250 ].
Nevertheless, to the best of our knowledge, there are no literature reports directly connecting the brewery spent yeast with the polymer technology.
4 Conclusions, future trends, and developments
The presented review summarized the literature works associated with the by-products of beer production. Their generation during brewing was discussed, and the beer market size, which affects the amount of by-products. Reported data about the composition of brewers’ spent grain, trub, spent hops, and spent yeast indicate that the application in polymer technology should be considered an auspicious method of their utilization, viable from the ecological and economic point of view. Moreover, their chemical composition, particularly the presence of compounds showing antimicrobial, antifungal and antioxidant activity, puts the brewing by-products as potential active fillers providing additional properties to polymer materials and promising intermediates in manufacturing various raw materials for polymer technology.
In order to take full advantage of the brewing by-products potential in polymer technology, future works should focus on the following issues:
Partial substitution of conventional lignocellulosic fillers in wood-polymer composites with brewers’ spent grain, which could enhance the resistance to thermooxidation.
Investigations related to the mechanisms of the enhancement of thermooxidative stability of various polymer materials by antioxidants present in brewing by-products.
The applications of brewing by-products in packaging materials or even edible films for food protection.
Liquefaction of brewing by-products resulting in raw materials for manufacturing of polyurethanes or polyester resins.
K.E. Behre, The history of beer additives in Europe—a review. Veg. Hist. Archaeobot. 8 (1–2), 35–48 (1999). https://doi.org/10.1007/bf02042841
Article Google Scholar
R.W. Unger, A History of Brewing in Holland, 900–1900. (Leiden, Brill, The Netherlands, 2001).
A. Smejtková, P. Vaculík, M. Přikryl, Z. Pastorek, Rating of malt grist fineness with respect to the used grinding equipment. Res. Agr. Eng. 62 (3), 141–146 (2016). https://doi.org/10.17221/41/2015-rae
F.S. De Almeida, C.A. de AndradeSilva, S.M. Lima, Y.R. Suarez, L.H. da CunhaAndrade, Use of Fourier transform infrared spectroscopy to monitor sugars in the beer mashing process. Food Chem. 263 , 112–118 (2018). https://doi.org/10.1016/j.foodchem.2018.04.109
Article CAS Google Scholar
B.V. Humia, K.S. Santos, J.K. Schneider, I.L. Leal, G de Abreu Barreto, T Batista, BA Souza Machado, JI Druzian, LC Krause, M de Costa Mendonca, FF Padilha, Physicochemical and sensory profile of Beauregard sweet potato beer. Food Chem. 312 , 126087 (2020). https://doi.org/10.1016/j.foodchem.2019.126087
S. Violino, S. Figorilli, C. Costa, F. Pallottino, Internet of beer: a review on smart technologies from mash to pint. Foods 9 , 950 (2020). https://doi.org/10.3390/foods9070950
R. Ravindran, S. Jaiswal, N. Abu-Ghannam, A.K. Jaiswal, A comparative analysis of pretreatment strategies on the properties and hydrolysis of brewers’ spent grain. Bioresource Technol. 248 , 272–279 (2018). https://doi.org/10.1016/j.biortech.2017.06.039
D. De Keukeleire, Fundamentals of beer and hop chemistry. Quím. Nova 23 (1), 108–112 (2000). https://doi.org/10.1590/s0100-40422000000100019
M. Hellwig, S. Witte, T. Henle, Free and protein-bound maillard reaction products in beer: method development and a survey of different beer types. J. Agr. Food Chem. 64 (38), 7234–7243 (2016). https://doi.org/10.1021/acs.jafc.6b02649
D.O. Schisler, J.J. Ruocco, M.S. Mabee, wort trub content and its effects on fermentation and beer flavor. J. Am. Soc. Brew. Chem. 40 (2), 57–61 (1982). https://doi.org/10.1094/asbcj-40-0057
D.A. Gee, W.F. Ramirez, A flavour model for beer fermentation. J. I. Brewing 100 (5), 321–329 (1994). https://doi.org/10.1002/j.2050-0416.1994.tb00830.x
J. Pasqualino, M. Meneses, F. Castells, The carbon footprint and energy consumption of beverage packaging selection and disposal. J. Food Eng. 103 (4), 357–365 (2011). https://doi.org/10.1016/j.jfoodeng.2010.11.005
Statista.com, Beer production worldwide from 1998 to 2019 (in billion hectoliters), https://www.statista.com/statistics/270275/worldwide-beer-production . Accessed 01 June 2021.
Statista.com, Beer production worldwide in 2019, by region (in million hectoliters), https://www.statista.com/statistics/202417/beer-output-volumes-of-the-different-continents-in-2010 . Accessed 01 June 2021.
Statista.com, Leading countries in beer production in the Americas in 2019 (in million hectoliters), https://www.statista.com/statistics/202409/beer-production-in-different-american-countries-in-2010 . Accessed 01 June 2021.
The Brewers of Europe, European Beer Trends. Statistic Report, 2020 Edition, https://brewersofeurope.org/uploads/mycms-files/documents/publications/2020/european-beer-trends-2020.pdf . Accessed 01 June 2021.
Statista.com, Ranking of the most popular beer types in Germany from 2017 to 2020, https://www.statista.com/statistics/575329/popular-beer-brands-germany . Accessed 01 June 2021.
Statista.com, Volume of beer produced in Europe in 2019, by country (in 1,000 hectoliters), https://www.statista.com/statistics/444575/european-beer-production-by-country . Accessed 01 June 2021.
Statista.com, Leading 10 countries in beer production of Europe in 2019 (in million hectoliters), https://www.statista.com/statistics/202400/beer-production-in-europe-in-2010 . Accessed 01 June 2021.
USA Beer Ratings, Top 10 beer producing countries, https://usabeerratings.com/en/blog/insights-1/top-10-beer-producing-countries-78.htm . Accessed 01 June 2021.
S. Wunderlich, W. Back, Overview of manufacturing beer: ingredients, processes, and quality criteria, in beer in health and disease prevention, 1 st Edition, ed. By V. Preedy (Academic Press, Cambridge, USA, 2008), pp. 3–16 (2009). https://doi.org/10.1016/b978-0-12-373891-2.00001-8
D. Brandauer, Food quality, in history – the genesis of the Bavarian Purity Law (beer) from 1516 until today, in Food Security, Food Safety, Food Quality . ed. by I. Hartel, R. Budzinowski (Nomos Verlagsges Mbh & Co KG, Baden-Baden, Germany, 2016), pp. 153–164
Google Scholar
W.J.W. Lloyd, Adjuncts. J. I. Brewing 92 (4), 336–345 (1986). https://doi.org/10.1002/j.2050-0416.1986.tb04420.x
K.S. Mallawarachchi, L.R.L.M. Bandara, S.K.D.H.S. Dilshan, T.U. Ariyadasa, S.H.P. Gunawardena, Optimization of mashing process in beer production using rice as an adjunct. 2016 Moratuwa Engineering Research Conference (MERCon, Morutawa, Sri Lanka, 2016), pp. 289–292. https://doi.org/10.1109/MERCon.2016.7480155
R.C. Agu, G.H. Palmer, A reassessment of sorghum for lager-beer brewing. Bioresource Technol. 66 (3), 253–261 (1998). https://doi.org/10.1016/s0960-8524(98)00047-9
E.J. Lodolo, J.L.F. Kock, B.C. Axcell, M. Brooks, The yeast Saccharomyces cerevisiae - the main character in beer brewing. FEMS Yeast Res. 8 (7), 1018–1036 (2008). https://doi.org/10.1111/j.1567-1364.2008.00433.x
O. Oladokun, S. James, T. Cowley, F. Dehrmann, K. Smart, J. Hort, D. Cook, Perceived bitterness character of beer in relation to hop variety and the impact of hop aroma. Food Chem. 230 , 215–224 (2017). https://doi.org/10.1016/j.foodchem.2017.03.031
A.A. Olajire, The brewing industry and environmental challenges. J. Clean. Prod. 256 , 102817 (2020). https://doi.org/10.1016/j.jclepro.2012.03.003
M. Batayneh, I. Marie, I. Asi, Use of selected waste materials in concrete mixes. Waste Manage. 27 (12), 1870–1876 (2007). https://doi.org/10.1016/j.wasman.2006.07.026
H. Nakajima, P. Dijkstra, K. Loos, The recent developments in biobased polymers toward general and engineering applications: polymers that are upgraded from biodegradable polymers, analogous to petroleum-derived polymers, and newly developed. Polymers 9 , 523 (2017). https://doi.org/10.3390/polym9100523
L. Dessbesell, M. Paleologou, M. Leitch, R. Pulkki, C. Xu, Global lignin supply overview and kraft lignin potential as an alternative for petroleum-based polymers. Renew. Sust. Energ. Rev. 123 , 109768 (2020). https://doi.org/10.1016/j.rser.2020.109768
S. Ikram, L. Huang, H. Zhang, J. Wang, M. Yin, Composition and Nutrient Value Proposition of Brewers Spent Grain. J. Food Sci. 82 (10), 2232–2242 (2017). https://doi.org/10.1111/1750-3841.13794
C. Wen, J. Zhang, Y. Duan, H. Zhang, H. Ma, A Mini-Review on Brewer’s Spent Grain Protein: Isolation, Physicochemical Properties, Application of Protein, and Functional Properties of Hydrolysates. J. Food Sci. 84 (12), 3330–3340 (2019). https://doi.org/10.1111/1750-3841.14906
A. Hejna, M. Marć, D. Kowalkowska-Zedler, A. Pladzyk, M. Barczewski, Insights into the thermo-mechanical treatment of brewers’ spent grain as a potential filler for polymer composites. Polymers 13 , 879 (2021). https://doi.org/10.3390/polym13060879
L.F. Guido, M.M. Moreira, Techniques for extraction of brewer’s spent grain polyphenols: a review. Food Bioprocess Technol. 10 (7), 1192–1209 (2017). https://doi.org/10.1007/s11947-017-1913-4
P. Valverde, Barley spent grain and its future. Cerveza y Malta 122 , 7–26 (1994)
E. Mallen, V. Najdanovic-Visak, Brewers’ spent grains: Drying kinetics and biodiesel production. Bioresource Technol. Report. 1 , 16–23 (2018). https://doi.org/10.1016/j.biteb.2018.01.005
J.I. Arranz, M.T. Miranda, F.J. Sepúlveda, I. Montero, C.V. Rojas, Analysis of Drying of Brewers’ Spent Grain. Proceedings. 2 , 1467 (2018). https://doi.org/10.3390/proceedings2231467
R. Slade, A. Bauen, N. Shah, The Commercial Performance of Cellulosic Ethanol Supply-Chains in Europe. Biotechnol. Biofuels 2 , 3 (2009). https://doi.org/10.1186/1754-6834-2-3
P. Nigam, A. Pandey, Biotechnology for Agro-Industrial Residues Utilisation (Springer, Dordrecht, The Netherlands, 2009)
Book Google Scholar
J. Buffington, The economic potential of brewer’s spent grain (BSG) as a biomass feedstock. Adv. Chem. Eng. Sci. 4 , 308–318 (2014). https://doi.org/10.4236/aces.2014.43034
T.R.S. Mathias, V.M.F. Alexandre, M.C. Cammarota, P.P.M. de Mello, E.F.C. Sérvulo, Characterization and determination of brewer’s solid wastes composition. J. I. Brewing 121 (3), 400–404 (2015). https://doi.org/10.1002/jib.229
M. Santos, J. Jiménez, B. Bartolomé, C. Gómez-Cordovés, M. del Nozal, Variability of brewer’s spent grain within a brewery. Food Chem. 80 (1), 17–21 (2003). https://doi.org/10.1016/s0308-8146(02)00229-7
I. Celus, K. Brijs, J.A. Delcour, The effects of malting and mashing on barley protein extractability. J. Cereal Sci. 44 (2), 203–211 (2006). https://doi.org/10.1016/j.jcs.2006.06.003
O. Kanauchi, K. Mitsuyama, Y. Araki, Development of a functional germinated barley foodstuff from brewers’ spent grain for the treatment of ulcerative colitis. J. Am. Soc. Brew. Chem. 59 , 59–62 (2001). https://doi.org/10.1094/ASBCJ-59-0059
S. Aliyu, M. Bala, Brewer’s spent grain: a review of its potentials and applications. Afr. J. Biotechnol. 10 (3), 324–331 (2011). https://doi.org/10.5897/AJBx10.006
S.I. Mussatto, G.J.M. Rocha, I.C. Roberto, Hydrogen peroxide bleaching of cellulose pulps obtained from brewer’s spent grain. Cellulose 15 , 641–649 (2008). https://doi.org/10.1007/s10570-008-9198-4
K.M. Lynch, E.J. Steffen, E.K. Arendt, Brewers’ spent grain: a review with an emphasis on food and health. J. I. Brewing 122 , 553–568 (2016). https://doi.org/10.1002/jib.363
F. Carvalheiro, M. Esteves, J. Parajó, H. Pereira, F. Gırio, Production of oligosaccharides by autohydrolysis of brewery’s spent grain. Bioresource Technol. 91 , 93–100 (2004). https://doi.org/10.1016/S0960-8524(03)00148-2
C. Xiros, E. Topakas, P. Katapodis, P. Christakopoulos, Hydrolysis and fermentation of brewer’s spent grain by Neurospora crassa. Bioresource Technol. 99 , 5427–5435 (2008). https://doi.org/10.1016/j.biortech.2007.11.010
N.G. Meneses, S. Martins, J.A. Teixeira, S.I. Mussatto, Influence of extraction solvents on the recovery of antioxidant phenolic compounds from brewer’s spent grains. Sep. Purif. Technol. 108 , 152–158 (2013). https://doi.org/10.1016/j.seppur.2013.02.015
M. Gupta, N. Abu-Ghannam, E. Gallaghar, Barley for brewing: characteristic changes during malting, brewing and applications of its by-products. Compr. Rev. Food Sci. F. 9 (3), 318–328 (2010). https://doi.org/10.1111/j.1541-4337.2010.00112.x
J.A. Robertson, K.J.A. I’Anson, J. Treimo, C.B. Faulds, T.F. Brocklehurst, V.G.H. Eijsink, K.W. Waldron, Profiling brewers’ spent grain for composition and microbial ecology at the site of production. LWT-Food Sci. Technol. 43 (6), 890–896 (2010). https://doi.org/10.1016/j.lwt.2010.01.019
D.M. Waters, F. Jacob, J. Titze, E.K. Arendt, E. Zannini, Fibre, protein and mineral fortification of wheat bread through milled and fermented brewer’s spent grain enrichment. Eur. Food Res. Technol. 235 , 767–778 (2012). https://doi.org/10.1007/s00217-012-1805-9
D. Yu, Y. Sun, W. Wang, S.F. O’Keefe, A.P. Neilson, H. Feng, Z. Wang, H. Huang, Recovery of protein hydrolysates from brewer’s spent grain using enzyme and ultrasonication. Int. J. Food Sci. Tech. 55 , 357–368 (2020). https://doi.org/10.1111/ijfs.14314
C.B. Faulds, A.I. Sancho, B. Bartolome, Mono- and dimeric ferulic acid release from brewer’s spent grain by fungal feruloyl esterases. Appl Microbiol Biot 60 (4), 489–493 (2002). https://doi.org/10.1007/s00253-002-1140-3
D. Szwajgier, A. Wasko, Z. Targonski, M. Niedzwiadek, M. Bancarzewska, The use of a novel ferulic acid esterase from lactobacillus acidophilus K1 for the release of phenolic acids from brewer’s spent grain. J. I. Brewing 116 (3), 293–303 (2010). https://doi.org/10.1002/j.2050-0416.2010.tb00434.x
A.C. Fărcaş, S.A. Socaci, F.V. Dulf, M. Tofană, E. Mudura, Z. Diaconeasa, Volatile profile, fatty acids composition and total phenolics content of brewers’ spent grain by-product with potential use in the development of new functional foods. J. Cereal Sci. 64 , 34–42 (2015). https://doi.org/10.1016/j.jcs.2015.04.003
A.L. McCarthy, Y.C. O’Callaghan, S. Neugart, C.O. Piggott, A. Connolly, M.A.K. Jansen, A. Krumbein, M. Schreiner, R.J. Fitzgerald, N.M. O’Brien, The hydroxycinnamic acid content of barley and brewers’ spent grain (BSG) and the potential to incorporate phenolic extracts of BSG as antioxidants into fruit beverages. Food Chem. 141 (3), 2567–2574 (2013). https://doi.org/10.1016/j.foodchem.2013.05.048
M.M. Moreira, S. Morais, D.O. Carvalho, A.A. Barros, C. Delerue-Matos, L.F. Guido, Brewer’s spent grain from different types of malt: Evaluation of the antioxidant activity and identification of the major phenolic compounds. Food Res. Int. 54 (1), 382–388 (2013). https://doi.org/10.1016/j.foodres.2013.07.023
K. Moraczewski, M. Stepczyńska, R. Malinowski, B. Budner, T. Karasiewicz, B. Jagodziński, Selected properties of polycaprolactone containing natural anti-aging compounds. Adv. Polym. Technol. 37 , 3499–3510 (2018). https://doi.org/10.1002/adv.22134
K. Moraczewski, M. Stepczyńska, R. Malinowski, T. Karasiewicz, B. Jagodziński, P. Rytlewski, The Effect of Accelerated Aging on Polylactide Containing Plant Extracts. Polymers 11 , 575 (2019). https://doi.org/10.3390/polym11040575
K. Moraczewski, M. Stepczyńska, R. Malinowski, B. Budner, T. Karasiewicz, B. Jagodziński, Selected properties of polylactide containing natural antiaging compounds. Polym. Adv. Technol. 29 (12), 2963–2971 (2018). https://doi.org/10.1002/pat.4416
L.C. Maillard, Action des acides amines sur les sucres; formation de melanoidines par voie méthodique. Compt. Rend. 154 , 66–68 (1912)
CAS Google Scholar
R.C. Borrelli, A. Visconti, C. Mennella, M. Anese, V. Fogliano, Chemical characterization and antioxidant properties of coffee melanoidins. J. Agr. Food Chem. 50 (22), 6527–6533 (2002). https://doi.org/10.1021/jf025686o
G. Alves, P. Xavier, R. Limoeiro, D. Perrone, Contribution of melanoidins from heat-processed foods to the phenolic compound intake and antioxidant capacity of the Brazilian diet. J. Food Sci. Technol. 57 , 3119–3131 (2020). https://doi.org/10.1007/s13197-020-04346-0
A. Karlović, A. Jurić, N. Ćorić, K. Habschied, V. Krstanović, K. Mastanjević, By-products in the malting and brewing industries—re-usage possibilities. Fermentation 6 , 82 (2020). https://doi.org/10.3390/fermentation6030082
T.R. Dhiman, H.R. Bingham, H.D. Radloff, Production response of lactating cows fed dried versus wet brewers’ grain in diets with similar dry matter content. J. Dairy. Sci. 86 , 2914–2921 (2003). https://doi.org/10.3168/jds.S0022-0302(03)73888-0
N.G. Belibasakis, D. Tsirgogianni, Effects of wet brewers grains on milk yield, milk composition and blood components of dairy cows in hot weather. Anim. Feed Sci. Tech. 57 , 175–181 (1996). https://doi.org/10.1016/0377-8401(95)00860-8
L. Sawadogo, H. Sepehri, L.M. Houdebine, Presence of a factor stimulating prolactin and growth hormone secretion in brewers’ spent grains. Reprod. Nutr. Dev. 29 , 139–146 (1989)
T.N.P. Gondwe, J.P. Mtimuni, A.C.L. Safalaoh, Evaluation of brewery by-products replacing vitamin premix in broiler finisher diets. Indian J. Anim. Sci. 69 , 347–349 (1999)
V.I. Kaur, P.K. Saxena, Incorporation of brewery waste in supplementary feed and its impact on growth in some carps. Bioresource Technol. 91 , 101–104 (2004). https://doi.org/10.1016/S0960-8524(03)00073-7
I.D.I. Yaakugh, T.S.B. Tegbe, S.A.S. Olorunju, A.O. Aduku, Replacement value of brewers’ dried grain for maize on performance of pigs. J. Sci. Food Agr. 66 , 465–471 (1994). https://doi.org/10.1002/jsfa.2740660407
A. Ktenioudaki, V. Chaurin, S.F. Reis, E. Gallagher, Brewer’s spent grain as a functional ingredient for breadsticks. Int. J. Food. Sci. Technol. 47 , 1765–1771 (2012). https://doi.org/10.1111/j.1365-2621.2012.03032.x
M.Z. Miranda, M.V.E. Grossmann, E.H. Nabeshima, Utilization of brewers’ spent grain for the production of snacks with fiber. 1. Physicochemical characteristics. Braz. Arch. Biol. Techn. 37 , 483–493 (1994)
H.Z. Hassona, High fibre bread containing brewer’s spent grains and its effect on lipid metabolism in rats. Nahrung 37 , 576–582 (1993). https://doi.org/10.1002/food.19930370609
N.J. Huige, Brewery by-products and effluents, in Handbook of Brewing, ed. By W.A. Hardwick (Marcel Dekker, New York, USA, 1994) pp. 501–550.
E.B. Özvural, H. Vural, I. Gokbulut, O. Ozboy-Ozbas, Utilization of brewer’s spent grain in the production of Frankfurters. Int. J. Food Sci. Technol. 44 , 1093–1099 (2009). https://doi.org/10.1111/j.1365-2621.2009.01921.x
M. Nagy, C.A. Semeniuc, S.A. Socaci, C.A. Pop, A.M. Rotar, C.D. Salagean, M. Tofana, Utilization of brewer’s spent grain and mushrooms in fortification of smoked sausages. Food Sci. Technol. 37 , 315–320 (2017). https://doi.org/10.1590/1678-457x.23816
M.S. Choi, Y.S. Choi, H.W. Kim, K.E. Hwang, D.H. Song, S.Y. Lee, C.J. Kim, K. Hwang, D. Song, S. Lee, C. Kim, Effects of replacing pork back fat with brewer’s spent grain dietary fiber on quality characteristics of reduced-fat chicken sausages. Food Sci. Animal Res. 34 , 158–165 (2014). https://doi.org/10.5851/kosfa.2014.34.2.158
H. Kim, K. Hwang, D. Song, S. Lee, M. Choi, Y. Lim, J. Choi, Y. Choi, H. Kim, C. Kim, Effects of dietary fiber extracts from brewer’s spent grain on quality characteristics of chicken patties cooked in convective oven. J. Am. Soc. Brew. Chem. 33 , 45–52 (2013). https://doi.org/10.5851/kosfa.2013.33.1.45
A. McCarthy, Y. O’Callaghan, C. Piggott, R. Fitzgerald, N. O’Brien, Brewers’ spent grain; bioactivity of phenolic component, its role in animal nutrition and potential for incorporation in functional foods: A review. P. Nutr. Soc. 72 (1), 117–125 (2013). https://doi.org/10.1017/S0029665112002820
K. Rachwał, A. Waśko, K. Gustaw, M. Polak-Berecka, Utilization of brewery wastes in food industry. PeerJ 8 , e9427 (2020). https://doi.org/10.7717/peerj.9427
G. Sperandio, T. Amoriello, K. Carbone, M. Fedrizzi, A. Monteleone, S. Tarangioli, M. Pagano, Increasing the value of spent grains from craft microbreweries for energy purposes. Chem. Engineer. Trans. 58 , 487–492 (2017). https://doi.org/10.3303/CET1758082
R. Meyer-Pittroff, Utilization of spent brewers’ grain for energy production. Brauwelt 128 , 1156–1158 (1988)
L.D.M.S. Borel, T.S. Lira, J.A. Ribeiro, C.H. Ataíde, M.A.S. Barrozo, Pyrolysis of brewer’s spent grain: Kinetic study and products identification. Ind. Crop. Prod. 121 , 388–395 (2018). https://doi.org/10.1016/j.indcrop.2018.05.051
W. Russ, H. Mörtel, R. Meyer-Pittroff, Application of spent grains to increase porosity in bricks. Constr. Build. Mater. 19 , 117–126 (2005). https://doi.org/10.1016/j.conbuildmat.2004.05.014
H. Okamoto, K. Sato, N. Yagi, M. Inoue, S. Yamasaki, S. Ishida, J. Shibata, Development of production process of charcoal bricks from spent grain. Kagaku Kogaku Ronbun. 28 , 137–142 (2002). https://doi.org/10.1252/kakoronbunshu.28.137
S. Bedő, M. Rozbach, L. Nagy, A. Fehér, C. Fehér, Optimised Fractionation of Brewer’s Spent Grain for a Biorefinery Producing Sugars, Oligosaccharides, and Bioethanol. Processes 9 , 366 (2021). https://doi.org/10.3390/pr9020366
R. Liguori, C.R. Soccol, L. Porto de Souza Vandenberghe, A.L. Woiciechowski, V. Faraco, Second generation ethanol production from brewers’ spent grain. Energies. 8 , 2575–2586 (2015). https://doi.org/10.3390/en8042575
C. Xiros, P. Christakopoulos, Enhanced ethanol production from brewer’s spent grain by a Fusarium oxysporum consolidated system. Biotechnol. Biofuel. 2 , 1–12 (2009). https://doi.org/10.1186/1754-6834-2-4
B. Gunes, J. Stokes, P. Davis, C. Connolly, J. Lawler, Pre-treatments to enhance biogas yield and quality from anaerobic digestion of whiskey distillery and brewery wastes: A review. Renew. Sust. Energ. Rev. 113 , 109281 (2009). https://doi.org/10.1016/j.rser.2019.109281
A. Szaja, A. Montusiewicz, M. Lebiocka, M. Bis, The effect of brewery spent grain application on biogas yields and kinetics in co-digestion with sewage sludge. PeerJ 8 , e10590 (2020). https://doi.org/10.7717/peerj.10590
M. Panjičko, G.D. Zupančič, L. Fanedl, R.M. Logar, M. Tišma, B. Zelić, Biogas production from brewery spent grain as a mono-substrate in a two-stage process composed of solid-state anaerobic digestion and granular biomass reactors. J. Clean. Prod. 166 , 519–529 (2017). https://doi.org/10.1016/j.jclepro.2017.07.197
M. Dudek, K. Świechowski, P. Manczarski, J.A. Koziel, A. Białowiec, The effect of biochar addition on the biogas production kinetics from the anaerobic digestion of brewers’ spent grain. Energies 12 , 1518 (2019). https://doi.org/10.3390/en12081518
S.T. Cooray, J.J.L. Lee, W.N. Chen, Evaluation of brewers’ spent grain as a novel media for yeast growth. AMB Express 7 (1), 117 (2017). https://doi.org/10.1186/s13568-017-0414-1
S.I. Mussatto, M. Fernandes, G. Dragone, I.M. Mancilha, I.C. Roberto, Brewer’s spent grain as raw material for lactic acid production by Lactobacillus delbrueckii. Biotechnol. Lett. 29 , 1973–1976 (2007). https://doi.org/10.1007/s10529-007-9494-3
S. Shindo, T. Tachibana, Production of L-lactic acid from spent grain, a by-product of beer production. J. I. Brewing 110 , 347–351 (2004). https://doi.org/10.1002/j.2050-0416.2004.tb00631.x
J. Pejin, M. Radosavljević, S. Kocić-Tanackov, R. Marković, A. Djukić-Vuković, L. Mojović, Use of spent brewer’s yeast in L-(+) lactic acid fermentation. J. I. Brewing 125 , 357–363 (2019). https://doi.org/10.1002/jib.572
R. Singh, G. Saini, Biosynthesis of pullulan and its applications, in food and pharmaceutical industry, in Microorganisms in sustainable agriculture and biotechnology . ed. by T. Satyanarayana, B. Johri, A. Prakash (Springer, Dordrecht, The Netherlands, 2012), pp. 509–553
F. Carvalheiro, L.C. Duarte, R. Medeiros, F.M. Gírio, Xylitol production by Debaryomyces hansenii in brewery spent grain diluteacid hydrolysate: effect of supplementation. Biotechnol. Lett. 29 , 1887–1891 (2007). https://doi.org/10.1007/s10529-007-9468-5
F. Carvalheiro, L.C. Duarte, S. Lopes, J.C. Parajò, H. Pereira, F.M. Gírio, Supplementation requirements of brewery’s spent grain hydrolysate for biomass and xylitol production by Debaryomyces hansenii CCMI 941. J. Ind. Microbiol. Biotechnol. 33 , 646–654 (2006). https://doi.org/10.1007/s10295-006-0101-8
F. Carvalheiro, L.C. Duarte, S. Lopes, J.C. Parajò, H. Pereira, F.M. Gírio, Evaluation of the detoxification of brewery’s spent grain hydrolysate for xylitol production by Debaryomyces hansenii CCMI 941. Process Biochem. 40 , 1215–1223 (2005). https://doi.org/10.1016/j.procbio.2004.04.015
T.O. Femi-Ola, V.A. Atere, Citric acid production from brewers spent grain by Aspergillus niger and Saccharomyces cerevisiae. Int. J. Res. BioSci. 2 , 30–36 (2013)
R. Balakrishnan, S.K. Rajaram, S. Sivaprakasam, Biovalorization potential of agro-forestry/industry biomass for optically pure lactic acid fermentation: Opportunities and challenges, in Biovalorisation of Wastes to Renewable Chemicals and Biofuels . ed. by N.K. Rathinam, R.K. Sani (Elsevier, Cambridge, MA, USA, 2020), pp. 261–276
Chapter Google Scholar
S. Inkinen, M. Hakkarainen, A.C. Albertsson, A. Södergård, From Lactic Acid to Poly(lactic acid) (PLA): Characterization and Analysis of PLA and Its Precursors. Biomacromol 12 (3), 523–532 (2011). https://doi.org/10.1021/bm101302t
Z. Liu, Y. Wang, B. Wu, C. Cui, Y. Guo, C. Yan, A critical review of fused deposition modeling 3D printing technology in manufacturing polylactic acid parts. Int. J. Adv. Manuf. Technol. 102 , 2877–2889 (2019). https://doi.org/10.1007/s00170-019-03332-x
B. Choi, S. Yoo, S. Park, Carbon footprint of packaging films made from LDPE, PLA, and PLA/PBAT blends in South Korea. Sustainability 10 , 2369 (2018). https://doi.org/10.3390/su10072369
S.T. Cooray, W.N. Chen, Valorization of brewer’s spent grain using fungi solid-state fermentation to enhance nutritional value. J. Funct. Food. 42 , 85–94 (2018). https://doi.org/10.1016/j.jff.2017.12.027
S. Liang, C. Wan, Carboxylic acid production from brewer’s spent grain via mixed culture fermentation. Bioresource Technol. 182 , 179–183 (2015). https://doi.org/10.1016/j.biortech.2015.01.082
J. Yang, A.R. Webb, G.A. Ameer, Novel Citric Acid-Based Biodegradable Elastomers for Tissue Engineering. Adv. Mater. 16 , 511–516 (2004). https://doi.org/10.1002/adma.200306264
H. Namazi, M. Adeli, Dendrimers of citric acid and poly (ethylene glycol) as the new drug-delivery agents. Biomaterials 26 , 1175–1183 (2005). https://doi.org/10.1016/j.biomaterials.2004.04.014
I. Djordjevic, N.R. Choudhury, N.K. Dutta, S. Kumar, Synthesis and characterization of novel citric acid-based polyester elastomers. Polymer 50 , 1682–1691 (2009). https://doi.org/10.1016/j.polymer.2009.01.045
D. Gyawali, P. Nair, Y. Zhang, R.T. Tran, C. Zhang, M. Samchukov, M. Makarov, H.K.W. Kim, J. Yang, Citric acid-derived in situ crosslinkable biodegradable polymers for cell delivery. Biomaterials 31 , 9092–9105 (2010). https://doi.org/10.1016/j.biomaterials.2010.08.022
C. Demitri, R. Del Sole, F. Scalera, A. Sannino, G. Vasapollo, A. Maffezzoli, L. Ambrosio, L. Nicolais, Novel superabsorbent cellulose-based hydrogels crosslinked with citric acid. J. Appl. Polym. Sci. 110 , 2453–2460 (2008). https://doi.org/10.1002/app.28660
N. Reddy, Y. Yang, Citric acid cross-linking of starch films. Food Chem. 118 , 702–711 (2010). https://doi.org/10.1016/j.foodchem.2009.05.050
W. Woigk, C.A. Fuentes, J. Rion, D. Hegemann, A.W. van Vuure, E. Kramer, C. Dransfeld, K. Masania, Fabrication of flax fibre-reinforced cellulose propionate thermoplastic composites. Compos. Sci. Technol. 183 , 107791 (2019). https://doi.org/10.1016/j.compscitech.2019.107791
H. Shaghaleh, X. Xu, S. Wang, Current progress in production of biopolymeric materials based on cellulose, cellulose nanofibers, and cellulose derivatives. RSC Adv. 8 (2), 825–842 (2018). https://doi.org/10.1039/c7ra11157f
F. Sharif, N. Muhammad, T. Zafar, Cellulose Based Biomaterials: Benefits and Challenges, in Biofibers and Biopolymers for Biocomposites, ed. By A. Khan, S. Mavinkere Rangappa, S. Siengchin, A. Asiri (Springer, Dordrecht, The Netherlands, 2020). https://doi.org/10.1007/978-3-030-40301-0_11
C. Gauss, K.L. Pickering, L.P. Muthe, The use of cellulose in bio-derived formulations for 3D/4D printing: A review. Compos. Part C-Open Access 4 , 100113 (2021). https://doi.org/10.1016/j.jcomc.2021.100113
P. Puligundla, C. Mok, Recent advances in biotechnological valorization of brewers’ spent grain. Food Sci. Biotechnol. 30 , 341–353 (2021). https://doi.org/10.1007/s10068-021-00900-4
D.S. Tang, Y.J. Tian, Y.Z. He, L. Li, S.Q. Hu, B. Li, Optimisation of ultrasonic-assisted protein extraction from brewer’s spent grain. Czech J. Food Sci. 28 , 9–17 (2010)
D.S. Tang, G.M. Yin, Y.Z. He, S.Q. Hu, B. Li, L. Li, H.L. Liang, D. Borthakur, Recovery of protein from brewer’s spent grain by ultrafiltration. Biochem. Eng. J. 48 (1), 1–5 (2009). https://doi.org/10.1016/j.bej.2009.05.019
Q. Yanlin, Research Advance and Propect of Comprehensive Use of Brewer’s Spent Grain by Biotechnology. Food Ferment. Ind. 28 (1), 72–73 (2002)
G. Pierre, F. Sannier, R. Goude, A. Nouviaire, Z. Maache-Rezzoug, S.A. Rezzoug, T. Maugard, Evaluation of thermomechanical pretreatment for enzymatic hydrolysis of pure microcrystalline cellulose and cellulose from Brewers’ spent grain. J. Cereal Sci. 54 (3), 305–310 (2011). https://doi.org/10.1016/j.jcs.2011.06.004
P.K. Mishra, T. Gregor, R. Wimmer, Utilising Brewer’s Spent Grain as a Source of Cellulose Nanofibres Following Separation of Protein-based Biomass. Bio Resources. 12 (1), 107–116 (2016). https://doi.org/10.15376/biores.12.1.107-116
R.D. Chen, C.F. Huang, S. Hsu, Composites of waterborne polyurethane and cellulose nanofibers for 3D printing and bioapplications. Carbohyd. Polym. 212 , 75–88 (2019). https://doi.org/10.1016/j.carbpol.2019.02.025
M. Li, X. Tian, R. Jin, D. Li, Preparation and characterization of nanocomposite films containing starch and cellulose nanofibers. Ind. Crop. Prod. 123 , 654–660 (2018). https://doi.org/10.1016/j.indcrop.2018.07.043
A. Ghanbari, T. Tabarsa, A. Ashori, A. Shakeri, M. Mashkour, Thermoplastic starch foamed composites reinforced with cellulose nanofibers: Thermal and mechanical properties. Carbohyd. Polym. 197 , 305–311 (2018). https://doi.org/10.1016/j.carbpol.2018.06.017
D.M. Santos, A.L. de Bukzem, D.P.R. Ascheri, R. Signini, G.L.B. de Aquino, Microwave-assisted carboxymethylation of cellulose extracted from brewer’s spent grain. Carbohyd. Polym. 131 , 125–133 (2015). https://doi.org/10.1016/j.carbpol.2015.05.051
S.I. Mussatto, M. Fernandes, I.C. Roberto, Lignin recovery from brewer’s spent grain black liquor. Carbohyd. Polym. 70 (2), 218–223 (2007). https://doi.org/10.1016/j.carbpol.2007.03.021
S.I. Mussatto, I.C. Roberto, Chemical characterization and liberation of pentose sugars from brewer’s spent grain. J. Chem. Technol. Biotechnol. 81 , 268–274 (2006). https://doi.org/10.1002/jctb.1374
M. Mendis, S. Simsek, Arabinoxylans and human health. Food Hydrocolloid. 42 , 239–243 (2014). https://doi.org/10.1016/j.foodhyd.2013.07.022
R.S. Rao, G. Muralikrishna, Water soluble feruloyl arabinoxylans from rice and ragi: changes upon malting and their consequence on antioxidant activity. Phytochem 67 (1), 91–99 (2006). https://doi.org/10.1016/j.phytochem.2005.09.036
C. Péroval, F. Debeaufort, D. Despré, A. Voilley, Edible Arabinoxylan-Based Films. 1. Effects of Lipid Type on Water Vapor Permeability, Film Structure, and Other Physical Characteristics. J. Agr. Food Chem. 50 (14), 3977–3983 (2002). https://doi.org/10.1021/jf0116449
A. Höije, M. Gröndahl, K. Tømmeraas, P. Gatenholm, Isolation and characterization of physicochemical and material properties of arabinoxylans from barley husks. Carbohyd. Polym. 61 (3), 266–275 (2005). https://doi.org/10.1016/j.carbpol.2005.02.009
A. Höije, E. Sternemalm, S. Heikkinen, M. Tenkanen, P. Gatenholm, Material Properties of Films from Enzymatically Tailored Arabinoxylans. Biomacromol 9 (7), 2042–2047 (2008). https://doi.org/10.1021/bm800290m
Y. Zhang, L. Pitkänen, J. Douglade, M. Tenkanen, C. Remond, C. Joly, Wheat bran arabinoxylans: Chemical structure and film properties of three isolated fractions. Carbohyd. Polym. 86 (2), 852–859 (2011). https://doi.org/10.1016/j.carbpol.2011.05.036
J.S. Stevanic, E.M. Bergström, P. Gatenholm, L. Berglund, L. Salmen, Arabinoxylan/nanofibrillated cellulose composite films. J. Mater. Sci. 47 , 6724–6732 (2012). https://doi.org/10.1007/s10853-012-6615-8
G. Mandalari, C.B. Faulds, A.I. Sancho, A. Saija, G. Bisignano, R. LoCurto, K.W. Waldron, Fractionation and characterisation of arabinoxylans from brewers’ spent grain and wheat bran. J. Cereal Sci. 42 (2), 205–212 (2005). https://doi.org/10.1016/j.jcs.2005.03.001
E. Coelho, M.A.M. Rocha, A.S.P. Moreira, M.R.M. Domingues, M.A. Coimbra, Revisiting the structural features of arabinoxylans from brewers’ spent grain. Carbohyd. Polym. 139 , 167–176 (2016). https://doi.org/10.1016/j.carbpol.2015.12.006
E. Vieira, M.A.M. Rocha, E. Coelho, O. Pinho, J.A. Saraiva, I.M.P.L.V.O. Ferreira, M.A. Coimbra, Valuation of brewer’s spent grain using a fully recyclable integrated process for extraction of proteins and arabinoxylans. Ind. Crop. Prod. 52 , 136–143 (2014). https://doi.org/10.1016/j.indcrop.2013.10.012
S.F. Reis, E. Coelho, M.A. Coimbra, N. Abu-Ghannam, Influence of grain particle sizes on the structure of arabinoxylans from brewer’s spent grain. Carbohyd. Polym. 130 , 222–226 (2015). https://doi.org/10.1016/j.carbpol.2015.05.031
C. Moreirinha, C. Vilela, N.H.C.S. Silva, R.R.J. Pinto, A. Almeida, M.A.M. Rocha, E. Coelho, M.A. Coimbra, A.J.D. Silvestre, C.S.R. Freire, Antioxidant and antimicrobial films based on brewers spent grain arabinoxylans, nanocellulose and feruloylated compounds for active packaging. Food Hydrocolloid. 108 , 105836 (2020). https://doi.org/10.1016/j.foodhyd.2020.105836
C. Xiros, M. Moukouli, E. Topakas, P. Christakopoulos, Factors affecting ferulic acid release from Brewer’s spent grain by Fusarium oxysporum enzymatic system. Bioresource Technol. 100 , 5917–5921 (2009). https://doi.org/10.1016/j.biortech.2009.06.018
M.M. Moreira, S. Morais, A.A. Barros, C. Delerue-Matos, L.F. Guido, A novel application of microwave-assisted extraction of polyphenols from brewer’s spent grain with HPLC-DAD-MS analysis. Anal. Bioanal. Chem. 403 , 1019–1029 (2012). https://doi.org/10.1007/s00216-011-5703-y
C.B. Faulds, G. Mandalari, R. Locurto, G. Bisignano, K.W. Waldron, Arabinoxylan and mono- and dimeric ferulic acid release from brewers’ grain and wheat bran by feruloyl esterases and glycosyl hydrolases from Humicola insolens. Appl. Microbiol. Biot. 64 , 644–650 (2004). https://doi.org/10.1007/s00253-003-1520-3
K. Moraczewski, A. Pawłowska, M. Stepczyńska, R. Malinowski, D. Kaczor, B. Budner, K. Gocman, P. Rytlewski, Plant extracts as natural additives for environmentally friendly polylactide films. Food Pack. Shelf Life 26 , 100593 (2020). https://doi.org/10.1016/j.fpsl.2020.100593
J. Liszkowska, M. Borowicz, J. Paciorek-Sadowska, M. Isbrandt, B. Czupryński, K. Moraczewski, Assessment of photodegradation and biodegradation of RPU/PIR foams modified by natural compounds of plant origin. Polymers 12 , 33 (2020). https://doi.org/10.3390/polym12010033
K. Moraczewski, R. Malinowski, W. Sikorska, T. Karasiewicz, M. Stepczyńska, B. Jagodziński, P. Rytlewski, Composting of polylactide containing natural anti-aging compounds of plant origin. Polymers 11 , 1582 (2019). https://doi.org/10.3390/polym11101582
X.J. Wang, J.C. Qi, X. Wang, L.P. Cao, Extraction of polyphenols from barley (Hordeum vulgare L.) grain using ultrasound-assisted extraction technology. Asia. J. Chem. 25 (3), 1324–1330 (2013)
S. Spinelli, A. Conte, L. Lecce, L. Padalino, M.A. Del Nobile, Supercritical carbon dioxide extraction of brewer’s spent grain. J. Supercrit. Fluid. 107 , 69–74 (2016). https://doi.org/10.1016/j.supflu.2015.08.017
W. Routray, V. Orsat, Microwave-assisted extraction of flavonoids: a review. Food Bioprocess Technol. 5 (2), 409–424 (2012). https://doi.org/10.1007/s11947-011-0573-z
J. Steiner, S. Procopio, T. Becker, Brewer’s spent grain: Source of value-added polysaccharides for the food industry in reference to the health claims. Eur. Food Res. Technol. 241 (3), 303–315 (2015). https://doi.org/10.1007/s00217-015-2461-7
F.K. Nzekoue, S. Angeloni, L. Navarini, C. Angeloni, M. Freschi, S. Hrelia, L.A. Vitali, G. Sagratini, S. Vittori, G. Caprioli, Coffee silverskin extracts: Quantification of 30 bioactive compounds by a new HPLC-MS/MS method and evaluation of their antioxidant and antibacterial activities. Food Res. Int. 133 , 109128 (2020). https://doi.org/10.1016/j.foodres.2020.109128
A. Guglielmetti, V. D’Ignoti, D. Ghirardello, S. Belviso, G. Zeppa, Optimisation of ultrasound and microwave-assisted extraction of caffeoylquinic acids and caffeine from coffee silverskin using response surface methodology. Italian J. Food Sci. 29 (3), 409–423 (2017). https://doi.org/10.14674/IJFS-727
A. Hejna, Potential applications of by-products from the coffee industry in polymer technology – Current state and perspectives. Waste Manage. 121 , 296–330 (2021). https://doi.org/10.1016/j.wasman.2020.12.018
T. Bonifácio-Lopes, J.A. Teixeira, M. Pintado, Current extraction techniques towards bioactive compounds from brewer’s spent grain – A review. Crit. Rev. Food Sci. Nutr. 60 , 2730–2741 (2020). https://doi.org/10.1080/10408398.2019.1655632
A. Hejna, P. Kosmela, M. Kopczynska, J. Haponiuk, L. Piszczyk, Microwave Assisted Liquefaction with Crude Glycerol as a Potential Method of Brewer’s Spent Grain Utilization. Chem. Chem. Technol. 10 (4), 445–450 (2016). https://doi.org/10.23939/chcht10.04.445
L. Krišták, R. Réh, Application of Wood Composites. Appl. Sci. 11 , 3479 (2021). https://doi.org/10.3390/app11083479
S.T. Cholake, R. Rajarao, P. Henderson, R.R. Rajagopal, V. Sahajwalla, Composite panels obtained from automotive waste plastics and agricultural macadamia shell waste. J. Clean. Prod. 151 , 163–171 (2017). https://doi.org/10.1016/j.jclepro.2017.03.074
K.S. Chun, V. Subramaniam, C.M. Yeng, P.M. Meng, C.T. Ratnam, T.K. Yeow, C.K. How, Wood plastic composites made from post-used polystyrene foam and agricultural waste. J. Thermoplast. Compos. Mater. 32 , 1455–1466 (2019). https://doi.org/10.1177/0892705718799836
A. Hejna, K. Formela, M.R. Saeb, Processing, mechanical and thermal behavior assessments of polycaprolactone/agricultural wastes biocomposites. Ind. Crop. Prod. 76 , 725–733 (2015). https://doi.org/10.1016/j.indcrop.2015.07.049
A. Revert, M. Reig, V.J. Seguí, T. Boronat, V. Fombuena, R. Balart, Upgrading brewer’s spent grain as functional filler in polypropylene matrix. Polym. Compos. 38 (1), 40–47 (2015). https://doi.org/10.1002/pc.23558
M.C. Barbu, Z. Montecuccoli, J. Förg, U. Barbeck, P. Klímek, A. Petutschnigg, E.M. Tudor, Potential of Brewer’s Spent Grain as a Potential Replacement of Wood in pMDI. UF or MUF Bonded Particleboard. Polymers 13 , 319 (2021). https://doi.org/10.3390/polym13030319
P. Antov, L. Krišták, R. Réh, V. Savov, A.N. Papadopoulos, Eco-Friendly Fiberboard Panels from Recycled Fibers Bonded with Calcium Lignosulfonate. Polymers 13 , 639 (2021). https://doi.org/10.3390/polym13040639
P. Antov, G.I. Mantanis, V. Savov, Development of wood composites from recycled fibres bonded with magnesium lignosulfonate. Forests 11 (6), 613 (2020). https://doi.org/10.3390/f11060613
R. Réh, L. Krištak, J. Sedliačik, P. Bekhta, M. Božiková, D. Kunecová, V. Vozárová, E.M. Tudor, P. Antov, V. Savov, Utilization of birch bark as an eco-friendly filler in urea-formaldehyde adhesives for plywood manufacturing. Polymers 13 , 511 (2021). https://doi.org/10.3390/polym13040511
P. Klímek, R. Wimmer, P Kumar Mishra, J Kúdela, Utilizing brewer’s-spent-grain in wood-based particleboard manufacturing. J. Clean. Prod. 141 , 812–817 (2017). https://doi.org/10.1016/j.jclepro.2016.09.152
A.M. Ferreira, J. Martins, L.H. Carvalho, F.D. Magalhães, Biosourced disposable trays made of brewer’s spent grain and potato starch. Polymers 11 , 923 (2019). https://doi.org/10.3390/polym11050923
A. Hejna, J. Haponiuk, Ł Piszczyk, M. Klein, K. Formela, Performance properties of rigid polyurethane-polyisocyanurate/brewers’ spent grain foamed composites as function of isocyanate index. e-Polymers. 17 (5), 427–437 (2017). https://doi.org/10.1515/epoly-2017-0012
K. Formela, A. Hejna, Ł Zedler, M. Przybysz, J. Ryl, M.R. Saeb, Ł Piszczyk, Structural, thermal and physico-mechanical properties of polyurethane/brewers’ spent grain composite foams modified with ground tire rubber. Ind. Crop. Prod. 108 , 844–852 (2017). https://doi.org/10.1016/j.indcrop.2017.07.047
A. Hejna, M. Barczewski, K. Skórczewska, J. Szulc, B. Chmielnicki, J. Korol, K. Formela, Sustainable upcycling of brewers’ spent grain by thermo-mechanical treatment in twin-screw extruder. J. Clean. Prod. 285 , 124839 (2021). https://doi.org/10.1016/j.jclepro.2020.124839
A. Hejna, M. Barczewski, P. Kosmela, O. Mysiukiewicz, Inhibition of Polymer Photodegradation by Incorporation of Coffee Silverskin. Proceedings. 69 , 1 (2021). https://doi.org/10.3390/CGPM2020-07219
A. Hejna, Poly(ε-Caprolactone)/Brewers’ Spent Grain Composites—The Impact of Filler Treatment on the Mechanical Performance. J. Compos. Sci. 4 , 167 (2020). https://doi.org/10.3390/jcs4040167
A. Hejna, J. Korol, P. Kosmela, A. Kuzmin, A. Piasecki, A. Kulawik, B. Chmielnicki, By-products from food industry as a promising alternative for the conventional fillers for wood–polymer composites. Polymers 13 , 893 (2021). https://doi.org/10.3390/polym13060893
S. Lu, S.W. Gibb, Copper removal from wastewater using spentgrain as biosorbent. Bioresource Technol. 99 , 1509–1517 (2008). https://doi.org/10.1016/j.biortech.2007.04.024
K.S. Low, C.K. Lee, C.H. Low, Sorption of chromium (VI) by spent grain under batch conditions. J. Appl. Polym. Sci. 82 , 2128–2134 (2001). https://doi.org/10.1002/app.2058
Q. Li, L. Chai, Z. Yang, Q. Wang, Kinetics and thermodynamics of Pb(II) adsorption onto modified spent grain from aqueous solutions. Appl. Surface Sci. 255 , 4298–4303 (2009). https://doi.org/10.1016/j.apsusc.2008.11.024
N. Ishiwaki, H. Murayama, H. Awayama, O. Kanauchi, T. Sato, Development of high value uses of spent grain by fractionation technology. MBAA Tech. Quarter. 37 , 261–265 (2000)
A.I. Osman, E. O’Connor, G. McSpadden, J.K. Abu-Dahrieh, C. Farrell, A.H. Al-Muhtaseb, J. Harrison, D.W. Rooney, Upcycling brewer’s spent grain waste into activated carbon and carbon nanotubes via two-stage activation for energy and other applications. J. Chem. Technol. Biotechnol. 95 (1), 183–195 (2020). https://doi.org/10.1002/jctb.6220
J.P. Silva, S. Sousa, I. Goncalves, J.J. Porter, S. Ferreira-Dias, Modeling adsorption of acid orange 7 dye in aqueous solutions to spent brewery grains. Sep. Purif. Technol. 40 , 163–170 (2004). https://doi.org/10.1016/j.seppur.2004.02.006
P. Silva, S. Sousa, J. Rodrigues, H. Antunes, J.J. Porter, I. Goncalves, S. Ferreira-Dias, Adsorption of acid orange 7 dye in aqueous solutions by spent brewery grains. Sep. Purif. Technol. 40 , 309–315 (2004). https://doi.org/10.1016/j.seppur.2004.03.010
A. Kezerle, N. Velic, D. Hasenay, D. Kovacevic, Lignocellulosic Materials as Dye Adsorbents: Adsorption of Methylene Blue and Congo Red on Brewers’ Spent Grain. Croatica Chem. Acta 91 (1), 53–64 (2018). https://doi.org/10.5562/cca3289
J. Wu, Z. Zhang, J. Xu, X. Lu, C. Wang, H. Xu, H. Yuan, J. Zhang, Brewer’s grains with different pretreatments used as bio-adsorbents for the removal of Congo red dye from aqueous solution. BioResources 15 (3), 6928–6940 (2020)
P.C. Chiang, P. Chang, J.H. You, Innovative technology for controlling VOC emissions. J. Hazard. Mater. 31 , 19–28 (1992). https://doi.org/10.1016/0304-3894(92)87036-F
Q. Li, L. Chai, W. Qin, Cadmium(II) adsorption on esterified spent grain: equilibrium modeling and possible mechanisms. Chem. Eng. J. 197 , 173–180 (2012). https://doi.org/10.1016/j.cej.2012.04.102
K.S. Low, C.K. Lee, S.C. Liew, Sorption of cadmium and lead from aqueous solutions by spent grain. Process Biochem. 36 , 59–64 (2000). https://doi.org/10.1016/S0032-9592(00)00177-1
O.C. Izinyon, O.E. Nwosu, L.O. Akhigbe, I.R. Ilaboya, Performance evaluation of Fe (III) adsorption onto brewers’ spent grain. Niger. J. Technol. 35 , 970–978 (2016). https://doi.org/10.4314/njt.v35i4.36
L. Chai, Q. Li, Y. Zhu, Z. Zhang, Q. Wang, Y. Wang, Z. Yang, Synthesis of thiol-functionalized spent grain as a novel adsorbent for divalent metal ions. Bioresource Technol. 101 (15), 6269–6272 (2010). https://doi.org/10.1016/j.biortech.2010.03.009
D. Kukić, M. Šćiban, J. Pejin, V. Vasić, J. Prodanović, Brewer’s spent grain as a potential adsorbent of heavy metal ions from water. Zaštita materijala 57 (3), 397–403 (2016)
T.R.S. Mathias, P.P. Moretzsohn de Mello, E.F.C. Servulo, Solid wastes in brewing process: A review. J. Brewing Distill. 5 (1), 1–9 (2014). https://doi.org/10.5897/JBD2014.0043
B. Jaskula, E. Syryn, K. Goiris, G. De Rouck, F. Van Opstaele, J. De Clippeleer, G. Aerts, L. De Cooman, Hopping technology in relation to beer bitterness consistency and flavor stability. J. Am. Soc. Brew. Chem. 65 (1), 38–46 (2007). https://doi.org/10.1094/asbcj-2007-0112-03
T. Amoriello, R. Ciccoritti, Sustainability: recovery and reuse of brewing-derived by-products. Sustainability 13 , 2355 (2021). https://doi.org/10.3390/su13042355
S.I. Mussatto, Biotechnological potential of brewing industry by-products, in biotechnology for agro-industrial residues utilisation, ed. By P. Singh nee’ Nigam, A. Pandey (Springer, Dordrecht, The Netherlands, 2009), pp. 313–326. https://doi.org/10.1007/978-1-4020-9942-7_16
H.M. Esslinger, L. Narziss, Beer (Wiley-VCH Verlag GmbH & Co. KGaA, Weinheim, Germany, 2005)
S. Bedini, G. Flamini, J. Girardi, F. Cosci, B. Conti, Not just for beer: evaluation of spent hops (Humulus lupulus L.) as a source of eco-friendly repellents for insect pests of stored foods. J. Pest Sci. 88 (3), 583–592 (2015). https://doi.org/10.1007/s10340-015-0647-1
T. O’Rourke, Making the most of your hops. New Brewer 11 , 20–33 (1994)
W. RuB, R. Meyer-Pittroff, The use of phenolic protein precipitates (trub) from beer production in animal feed. Monatsschr Brauwiss 56 , 84–88 (2003)
E. Huszcza, A. Bartmanska, The implication of yeast in debittering of spent hops. Enzyme Microb. Technol. 42 , 421–425 (2008). https://doi.org/10.1016/j.enzmictec.2008.01.004
E. Huszcza, A. Bartmanska, M. Anioł, W. Mączka, A. Żołnierczyk, C. Wawrzeńczyk, Degradation of hop bitter acids by fungi. Waste Manage. 28 , 1406–1410 (2008). https://doi.org/10.1016/j.wasman.2007.06.014
B.R. Saraiva, F.A. Anjo, A.C.P. Vital, L.H.M. da Silva, C.Y.L. Ogawa, F. Sato, L.B. Coimbra, P.T. Matumoto-Pintro, Waste from brewing (trub) as a source of protein for the food industry. Int. J. Food Sci. Technol. 54 (4), 1247–1255 (2019). https://doi.org/10.1111/ijfs.14101
E.K. Nassary, E.R. Nasolwa, Unravelling disposal benefits derived from underutilized brewing spent products in Tanzania. J. Environ. Manage. 242 , 430–439 (2019). https://doi.org/10.1016/j.jenvman.2019.04.068
J. Mediouni-Ben-Jemaa, N. Tersim, K. Talebtoudert, M.L. Khouja, Insecticidal activities of oils from leaves of Laurus nobilis L. from Tunisia, Algeria and Morocco, and comparative chemical composition. J. Stored Prod. Res. 48 , 97–104 (2012). https://doi.org/10.1016/j.jspr.2011.10.003
G. Benelli, G. Flamini, A. Canale, P.L. Cioni, I. Molfetta, B. Conti, Repellence of Hyptis suaveolens whole essential oil and major constituents against adults of the granary weevil Sitophilus granarius. Bull. Insectol. 65 , 177–183 (2012)
A. Bartmańska, E. Wałecka-Zacharska, T. Tronina, J. Popłoński, S. Sordon, E. Brzezowska, J. Bania, E. Huszcza, Antimicrobial properties of spent hops extracts, flavonoids isolated therefrom, and their derivatives. Molecules 23 , 2059 (2018). https://doi.org/10.3390/molecules23082059
M. Anioł, E. Huszcza, A. Bartmanska, A. Zołnierczyk, W. Maczka, C. Wawrzenczyk, Trace analysis of hop essential oils in spent hop. J. Am. Soc. Brew. Chem. 65 , 214–218 (2007). https://doi.org/10.1094/ASBCJ-2007-0820-01
G. Laufenberg, B. Kunz, M. Nystroem, Transformation of vegetable waste into value added products: (A) the upgrading concept; (B) practical implementation. Bioresource Technol. 87 , 167–198 (2003). https://doi.org/10.1016/S0960-8524(02)00167-0
K. Fischer, H.P. Bipp, Generation of organic acids and monosaccharides by hydrolytic and oxidative transformation of food processing residues. Bioresource Technol. 96 , 831–842 (2005). https://doi.org/10.1016/j.biortech.2004.07.003
A. Grudniewska, J. Popłoński, Simple and green method for the extraction of xanthohumol from spent hops using deep eutectic solvents. Sep. Purif. Technol. 250 , 117196 (2020). https://doi.org/10.1016/j.seppur.2020.117196
F. Kuhbeck, M. Muller, W. Back, T. Kurz, M. Krottenthaler, Effect of hot trub and particle addition on fermentation performance of Saccharomyces cerevisiae. Enzyme Microb. Technol. 41 , 711–720 (2007). https://doi.org/10.1016/j.enzmictec.2007.06.007
R.B. Gilliland, Yeast reproduction during fermentation. J. I. Brewing 68 (3), 271–275 (1962). https://doi.org/10.1002/j.2050-0416.1962.tb01863.x
M.J. Lewis, T.W. Young, Brewing (Chapman and Hall, London, UK, 1995)
C. Kerby, F. Vriesekoop, An overview of the utilisation of brewery by-products as generated by british craft breweries. Beverages 3 , 24 (2017). https://doi.org/10.3390/beverages3020024
J. Liepins, E. Kovačova, K. Shvirksts, M. Grube, A. Rapoport, G. Kogan, Drying enhances immunoactivity of spent brewer’s yeast cell wall β-d-glucans. J. Biotechnol. 206 , 12–16 (2015). https://doi.org/10.1016/j.jbiotec.2015.03.024
B. Podpora, F. Świderski, A. Sadowska, R. Rakowska, G. Wasiak-Zys, Spent brewer’s yeast extracts as a new component of functional food. Czech J. Food Sci. 34 (6), 554–563 (2016). https://doi.org/10.17221/419/2015-CJFS
F.F. Jacob, M. Hutzler, F.J. Methner, Comparison of various industrially applicable disruption methods to produce yeast extract using spent yeast from top-fermenting beer production: Influence on amino acid and protein content. Eur. Food Res. Technol. 245 , 95–109 (2019). https://doi.org/10.1007/s00217-018-3143-z
E.F. Vieira, J. Carvalho, E. Pinto, S. Cunha, A.A. Almeida, I.M. Ferreira, Nutritive value, antioxidant activity and phenolic compounds profile of brewer’s spent yeast extract. J. Food Compos. Anal. 52 , 44–51 (2016). https://doi.org/10.1016/j.jfca.2016.07.006
A. Jaeger, E.K. Arendt, E. Zannini, A.W. Sahin, Brewer’s Spent Yeast (BSY), an Underutilized Brewing By-Product. Fermentation 6 , 123 (2020). https://doi.org/10.3390/fermentation6040123
L. Kruger, A.T.W. Pickerell, B. Axcell, The sensitivity of different brewing yeast strains to carbon dioxide inhibition: Fermentation and production of flavour-active volatile compounds. J. I. Brewing 98 (2), 133–138 (1992). https://doi.org/10.1002/j.2050-0416.1992.tb01100.x
M. Boateng, D.B. Okai, Y.O. Frimpong, Y.Y. Zeebone, Wet brewers’ spent grains and wet brewers’ spent yeast: problems associated with their usage and suggested solutions: A case study of the Ejisu-Juaben Municipality of Ghana. Livest. Res. Rural Develop. 27 , 5 (2015)
J. Wohlt, T. Corcione, P. Zajac, Effect of yeast on feed intake and performance of cows fed diets based on corn silage during early lactation. J. Dairy Sci. 81 , 1345–1352 (1998). https://doi.org/10.3168/jds.S0022-0302(98)75697-8
A. Vohra, P. Syal, A. Madan, Probiotic yeasts in livestock sector. Anim. Feed Sci. Technol. 219 , 31–47 (2016). https://doi.org/10.1016/j.anifeedsci.2016.05.019
B. Harlow, R. Bryant, S. Cohen, S. O’Connell, M.D. Flythe, Degradation of spent craft brewer’s yeast by caprine rumen hyper ammonia-producing bacteria. Lett. Appl. Microbiol. 63 , 307–312 (2016). https://doi.org/10.1111/lam.12623
C.A. Nugent, F.H. Tyler, The renal excretion of uric acid in patients with gout and in nongouty subjects. J. Clin. Inv. 38 (11), 1890–1898 (1959). https://doi.org/10.1172/JCI103966
R. Rakowska, A. Sadowska, E. Dybkowska, F. Świderski, Spent yeast as natural source of functional food additives. Rocz. Panstw. Zakl. Hig. 68 (2), 115–121 (2017)
G.V. Marson, R.J.S. de Castro, M.P. Belleville, M. DupasHubinger, Spent brewer’s yeast as a source of high added value molecules: a systematic review on its characteristics, processing and potential applications. World J. Microbiol. Biotechnol. 36 , 95 (2020). https://doi.org/10.1007/s11274-020-02866-7
P. Puligundla, C. Mok, S. Park, Advances in the valorization of spent brewer’s yeast. Innov. Food Sci. Emerg. 62 , 102350 (2020). https://doi.org/10.1016/j.ifset.2020.102350
U. Ben-Hamed, H. Seddighi, K. Thomas, Economic Returns of Using Brewery`s Spent Grain in Animal Feed. Int. J. Econ. Manage. Eng. 5 (2), 142–145 (2011)
V. Pszczolkowski, R. Bryant, B. Harlow, G. Aiken, L. Martin, M. Flythe, Effects of spent craft brewers’ yeast on fermentation and methane production by rumen microorganisms. Adv. Microbiol. 6 , 716–723 (2016). https://doi.org/10.4236/aim.2016.69070
F.F. Jacob, L. Striegel, M. Rychlik, M. Hutzler, F.J. Methner, Spent yeast from brewing processes: a biodiverse starting material for yeast extract production. Fermentation 5 , 51 (2019). https://doi.org/10.3390/fermentation5020051
Characteristics and potential applications, I.M.P.L.V.O. Ferreira, O. Pinhoa, E. Vieira, J.G. Tavarelaa, Brewer’s Saccharomyces yeast biomass. Trends Food Sci. Technol. 21 , 77–84 (2010). https://doi.org/10.1016/j.tifs.2009.10.008
E.F. Vieira, O. Pinho, I.M. Ferreira, Bio-functional properties of sardine protein hydrolysates obtained by brewer’s spent yeast and commercial proteases. J. Sci. Food Agric. 97 , 5414–5422 (2017). https://doi.org/10.1002/jsfa.8432
E.F. Vieira, J. Van Camp, I. Ferreira, C. Grootaert, Protein hydrolysate from canned sardine and brewing by-products improves TNF-α-induced inflammation in an intestinal–endothelial co-culture cell model. Eur. J. Nutr. 57 , 2275–2286 (2017). https://doi.org/10.1007/s00394-017-1503-2
T.R.D.S. Mathias, P.F. De Aguiar, J.B.D.A.E. Silva, P.P.M. De Mello, E.F.C. Sérvulo, Brewery Waste Reuse for Protease Production by Lactic Acid Fermentation. Food Technol. Biotechnol. 55 , 218–224 92017). https://doi.org/10.17113/ftb.55.02.17.4378
D. Djokoto, V. Dzogbefia, J. Oldham, Rapid Extraction of Pawpaw Juice with the Application of Locally Produced Pectic Enzymes from Saccharomyces Cerevisiae ATCC 51712. Food Biotechnol. 20 , 31–41 (2006). https://doi.org/10.1080/08905430500523970
V.P. Dzogbefia, E. Amoke, J.H. Oldham, W.O. Ellis, Production and use of yeast pectolytic enzymes to aid pineapple juice extraction. Food Biotechnol. 15 , 25–34 (2001). https://doi.org/10.1081/FBT-100103892
J. Kawa-Rygielska, W. Pietrzak, Ethanol fermentation of very high gravity (VHG) maize mashes by Saccharomyces cerevisiae with spent brewer’s yeast supplementation. Biomass Bioenerg. 60 , 50–57 (2014). https://doi.org/10.1016/j.biombioe.2013.10.028
J. Pejin, M. Radosavljević, S. Kocić-Tanackov, R. Marković, A. Djukić-Vuković, L. Mojović, Use of spent brewer’s yeast in L-(+) lactic acid fermentation. J. I. Brewing 125 (3), 357–363 (2019). https://doi.org/10.1002/jib.572
W. Pietrzak, J. Kawa-Rygielska, Utilization of spent brewer’s yeast for supplementation of distillery corn mashes. Pol. J. Chem. Technol. 15 (4), 102–106 (2013). https://doi.org/10.2478/pjct-2013-0076
R. Spajic, R.T. Burns, L.B. Moody, D. Kralik, V. Poznic, G. Bishop, Croatian food industry by-products: co-digestion with swine manure vs. use as liquid animal feed. T. ASABE 53 (4), 1245–1250 (2010). https://doi.org/10.13031/2013
J.V. Oliveira, M.M. Alves, J.C. Costa, Biochemical methane potential of brewery by-products. Clean Technol. Envir. 20 (2), 435–440 (2018). https://doi.org/10.1007/s10098-017-1482-2
G.D.C. Gonçalves, P.K. Nakamura, D.F. Furtado, M.T. Veit, Utilization of brewery residues to produces granular activated carbon and bio-oil. J. Clean. Prod. 168 , 908–916 (2017). https://doi.org/10.1016/j.jclepro.2017.09.089
J.G. Zeikus, M.K. Jain, P. Elankovan, Biotechnology of succinic acid production and markets for derived industrial products. Appl. Microbiol. Biotechnol. 51 , 545–552 (1999). https://doi.org/10.1007/s002530051431
E. Ranucci, Y. Liu, M.S. Lindblad, A.C. Albertsson, New biodegradable polymers from renewable sources. High molecular weight poly(ester carbonate)s from succinic acid and 1,3-propanediol. Macromol Rapid Commun. 21 , 680–684 (2000). https://doi.org/10.1002/1521-3927(20000601)21:10%3c680::AID-MARC680%3e3.0.CO;2-Y
M. Rakin, M. Vukasinovic, S. Siler-Marinkovic, M. Maksimovic, Contribution of lactic acid fermentation to improved nutritive quality vegetable juices enriched with brewer’s yeast autolysate. Food Chem. 100 , 599–602 (2007). https://doi.org/10.1016/j.foodchem.2005.09.077
C.P. Champagne, H. Gaudreau, J. Conway, Effect of the production or use of mixtures of bakers or brewers’ yeast extracts on their ability to promote growth of Lactobacilli and Pediococci. Electron. J. Biotechn. 6 , 185–197 (2003)
M. Radosavljević, J. Pejin, M. Pribić, S. Kocić-Tanackov, D. Mladenović, A. Djukić-Vuković, L. Mojović, Brewing and malting technology by-products as raw materials in L-(+)-lactic acid fermentation. J. Chem. Technol. Biotechnol. 95 (2), 339–347 (2018). https://doi.org/10.1002/jctb.5878
M. Jiang, K. Chen, Z. Liu, P. Wei, H. Ying, H. Chang, Succinic Acid Production by Actinobacillus succinogenes Using Spent Brewer’s Yeast Hydrolysate as a Nitrogen Source. Appl. Biochem. Biotechnol. 160 , 244–254 (2010). https://doi.org/10.1007/s12010-009-8649-1
K.Q. Chen, J. Li, J.F. Ma, M. Jiang, P. Wei, Z.M. Liu, H.J. Ying, Succinic acid production by Actinobacillus succinogenes using hydrolysates of spent yeast cells and corn fiber. Bioresource Technol. 102 (2), 1704–1708 (2011). https://doi.org/10.1016/j.biortech.2010.08.011
Download references
This work was supported by the National Science Centre (NCN, Poland) in the frame of SONATINA 2 project 2018/28/C/ST8/00187–Structure and properties of lignocellulosic fillers modified in situ during reactive extrusion.
Author information
Authors and affiliations.
Department of Polymer Technology, Gdańsk University of Technology, Narutowicza 11/1280-233, Gdańsk, Poland
Aleksander Hejna
You can also search for this author in PubMed Google Scholar
Corresponding author
Correspondence to Aleksander Hejna .
Ethics declarations
Competing interest.
The author declares no competing interests.
Rights and permissions
Open Access This article is licensed under a Creative Commons Attribution 4.0 International License, which permits use, sharing, adaptation, distribution and reproduction in any medium or format, as long as you give appropriate credit to the original author(s) and the source, provide a link to the Creative Commons licence, and indicate if changes were made. The images or other third party material in this article are included in the article's Creative Commons licence, unless indicated otherwise in a credit line to the material. If material is not included in the article's Creative Commons licence and your intended use is not permitted by statutory regulation or exceeds the permitted use, you will need to obtain permission directly from the copyright holder. To view a copy of this licence, visit http://creativecommons.org/licenses/by/4.0/ .
Reprints and permissions
About this article
Hejna, A. More than just a beer—the potential applications of by-products from beer manufacturing in polymer technology. emergent mater. 5 , 765–783 (2022). https://doi.org/10.1007/s42247-021-00304-4
Download citation
Received : 08 June 2021
Accepted : 08 September 2021
Published : 24 September 2021
Issue Date : June 2022
DOI : https://doi.org/10.1007/s42247-021-00304-4
Share this article
Anyone you share the following link with will be able to read this content:
Sorry, a shareable link is not currently available for this article.
Provided by the Springer Nature SharedIt content-sharing initiative
- Brewing by-products
- Brewers’ spent grain
- Spent yeast
- Wood polymer composites
- Polymer materials
- Find a journal
- Publish with us
- Track your research
Information
- Author Services
Initiatives
You are accessing a machine-readable page. In order to be human-readable, please install an RSS reader.
All articles published by MDPI are made immediately available worldwide under an open access license. No special permission is required to reuse all or part of the article published by MDPI, including figures and tables. For articles published under an open access Creative Common CC BY license, any part of the article may be reused without permission provided that the original article is clearly cited. For more information, please refer to https://www.mdpi.com/openaccess .
Feature papers represent the most advanced research with significant potential for high impact in the field. A Feature Paper should be a substantial original Article that involves several techniques or approaches, provides an outlook for future research directions and describes possible research applications.
Feature papers are submitted upon individual invitation or recommendation by the scientific editors and must receive positive feedback from the reviewers.
Editor’s Choice articles are based on recommendations by the scientific editors of MDPI journals from around the world. Editors select a small number of articles recently published in the journal that they believe will be particularly interesting to readers, or important in the respective research area. The aim is to provide a snapshot of some of the most exciting work published in the various research areas of the journal.
Original Submission Date Received: .
- Active Journals
- Find a Journal
- Proceedings Series
- For Authors
- For Reviewers
- For Editors
- For Librarians
- For Publishers
- For Societies
- For Conference Organizers
- Open Access Policy
- Institutional Open Access Program
- Special Issues Guidelines
- Editorial Process
- Research and Publication Ethics
- Article Processing Charges
- Testimonials
- Preprints.org
- SciProfiles
- Encyclopedia
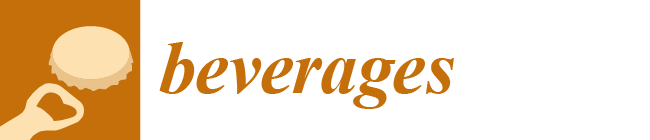
Journal Menu
- Beverages Home
- Aims & Scope
- Editorial Board
- Reviewer Board
- Topical Advisory Panel
- Instructions for Authors
- Special Issues
- Sections & Collections
- Article Processing Charge
- Indexing & Archiving
- Editor’s Choice Articles
- Most Cited & Viewed
- Journal Statistics
- Journal History
- Journal Awards
- Editorial Office
Journal Browser
- arrow_forward_ios Forthcoming issue arrow_forward_ios Current issue
- Vol. 10 (2024)
- Vol. 9 (2023)
- Vol. 8 (2022)
- Vol. 7 (2021)
- Vol. 6 (2020)
- Vol. 5 (2019)
- Vol. 4 (2018)
- Vol. 3 (2017)
- Vol. 2 (2016)
- Vol. 1 (2015)
Find support for a specific problem in the support section of our website.
Please let us know what you think of our products and services.
Visit our dedicated information section to learn more about MDPI.
Brewing and Craft Beer
- Print Special Issue Flyer
- Special Issue Editors
Special Issue Information
- Published Papers
A special issue of Beverages (ISSN 2306-5710).
Deadline for manuscript submissions: closed (28 February 2019) | Viewed by 73912
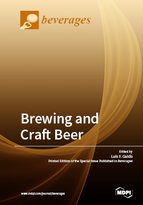
Share This Special Issue
Special issue editor.

Dear Colleagues,
Beer is a beverage with more than eight thousand years of history, and the process of brewing has not changed so much over the centuries. However, important technical advances have allowed us to produce beer in a more sophisticated and efficient way. The proliferation of specialty hop varieties has been behind the popularity of craft beers seen in the last few years around the world. Craft brewers interpret historic beer with unique styles. Craft beers are undergoing an unprecedented period of growth, and more than 150 beer styles are currently recognized.
This Special Issue, “Brewing and Craft Beer”, is aimed at giving the floor to the craft brewers who wish to share their findings, as well as to academic and industrial researchers. Coverage includes a selection of original research and current review articles related to the whole process, from cereal to beer, with particular emphasis on:
- Raw materials
- Key beer ingredients
- Specialty hops
- Malting technology
- Brewing technology
- Brewing yeast and fermentation
- Aroma and flavor
- Beer quality
- Innovative technologies
- Efficient breweries
Prof. Luis F. Guido Guest Editor
Manuscripts should be submitted online at www.mdpi.com by registering and logging in to this website . Once you are registered, click here to go to the submission form . Manuscripts can be submitted until the deadline. All submissions that pass pre-check are peer-reviewed. Accepted papers will be published continuously in the journal (as soon as accepted) and will be listed together on the special issue website. Research articles, review articles as well as short communications are invited. For planned papers, a title and short abstract (about 100 words) can be sent to the Editorial Office for announcement on this website.
Submitted manuscripts should not have been published previously, nor be under consideration for publication elsewhere (except conference proceedings papers). All manuscripts are thoroughly refereed through a single-blind peer-review process. A guide for authors and other relevant information for submission of manuscripts is available on the Instructions for Authors page. Beverages is an international peer-reviewed open access quarterly journal published by MDPI.
Please visit the Instructions for Authors page before submitting a manuscript. The Article Processing Charge (APC) for publication in this open access journal is 1600 CHF (Swiss Francs). Submitted papers should be well formatted and use good English. Authors may use MDPI's English editing service prior to publication or during author revisions.
- fermentation
- beer styles
Published Papers (10 papers)
Jump to: Research , Review
Jump to: Editorial , Review
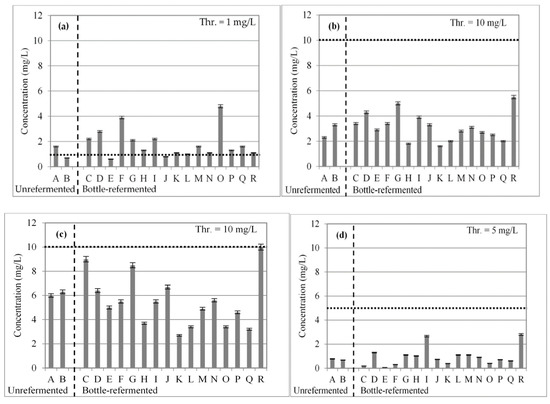
Graphical abstract
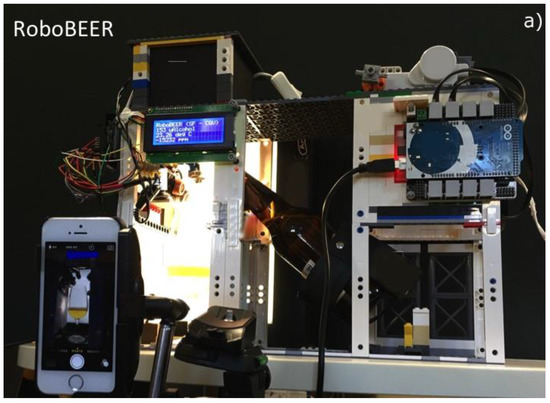
Jump to: Editorial , Research
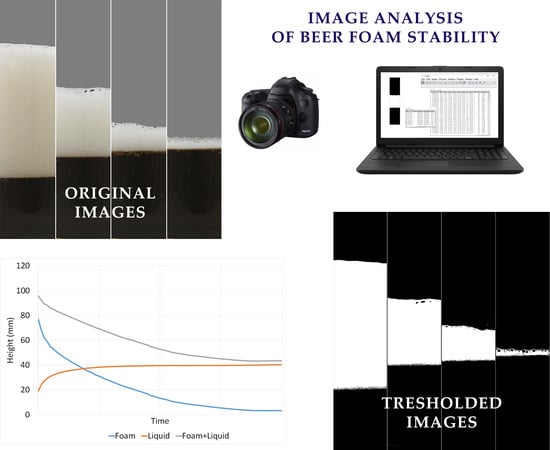
Further Information
Mdpi initiatives, follow mdpi.
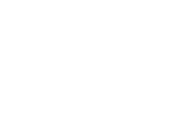
Subscribe to receive issue release notifications and newsletters from MDPI journals

An official website of the United States government
The .gov means it’s official. Federal government websites often end in .gov or .mil. Before sharing sensitive information, make sure you’re on a federal government site.
The site is secure. The https:// ensures that you are connecting to the official website and that any information you provide is encrypted and transmitted securely.
- Publications
- Account settings
Preview improvements coming to the PMC website in October 2024. Learn More or Try it out now .
- Advanced Search
- Journal List
- Wiley Open Access Collection

A Hands‐On Guide to Brewing and Analyzing Beer in the Laboratory
Florian a. thesseling.
1 Laboratory of Systems Biology, VIB Center for Microbiology, Leuven Belgium
2 Laboratory for Genetics and Genomics, Center of Microbial and Plant Genetics (CMPG), Department M2S, KU Leuven, Heverlee Belgium

Peter W. Bircham
Stijn mertens, karin voordeckers, kevin j. verstrepen.
Beer would not exist without microbes. During fermentation, yeast cells convert cereal‐derived sugars into ethanol and CO 2 . Yeast also produces a wide array of aroma compounds that influence beer taste and aroma. The complex interaction between all these aroma compounds results in each beer having its own distinctive palette. This article contains all protocols needed to brew beer in a standard lab environment and focuses on the use of yeast in beer brewing. More specifically, it provides protocols for yeast propagation, brewing calculations and, of course, beer brewing. At the end, we have also included protocols for analyses that can be performed on the resulting brew, with a focus on yeast‐derived aroma compounds. © 2019 The Authors.
INTRODUCTION
Beer brewing is intrinsically a biotechnological process: the conversion of raw materials into beer relies on many different enzymatic reactions and microbial activity. Beer is traditionally made from four key ingredients: malted cereals (barley or other), water, hops, and yeast. Each of these ingredients contributes to the final taste and aroma of beer.
Beer production starts with the malting of barley (or other cereals, such as wheat, sorghum, rye, or oats). The main goal of malting is to activate enzymes within the grain. These enzymes will hydrolyze starch and other compounds within the kernels during mashing (Goldammer, 2008 ; Kunze, 2004 ). During malting, barely kernels are soaked in water and periodically aerated, the so‐called steeping and germination phase. During germination, three important groups of enzymes are activated: (i) amylases, (ii) proteases/peptidases, and (iii) beta‐glucanases. Each of these enzymes have an important function during the malting and downstream brewing process: (i) amylases convert starch, present in the barley kernels, into fermentable sugars; (ii) proteases and peptidases break down proteins and release free amino nitrogen (FAN), while (iii) beta‐glucanases degrade the endosperm cell wall, allowing other enzymes access to the endosperm. Next, in the drying and kilning phase, kernels are dried and heated. This stops germination, arrests enzymatic activity within the kernels, reduces spoilage risks, and determines the impact of malt on the final aroma and color of the beer.
The actual brewing process consists of five steps. The main goal is to convert insoluble malt or grain material into a soluble and fermentable extract.
Milling of malted grains (i) and mashing (ii)
In this step, milled grains are mixed with warm water. This mash is kept at specific temperatures and pH to ensure proper enzymatic conversion of starch and proteins. Traditionally, a starting temperature of 45°C is used. At this temperature, proteases are activated and degrade proteins to short peptides and amino acids, that will form the major nitrogen source for yeast during fermentation. The mash is then heated to 62°C‐64°C, at which starch will gelatinize and become accessible to amylases. Beta‐amylases will cleave off maltose from starch molecules. The mash is then heated to 72°C for 15‐25 min, allowing further breakdown of long chain polysaccharides by alpha‐amylases. Finally, the temperature of the mash is raised to 78°C, stopping nearly all enzymatic activity.
Modern, highly modified malts allow mashing in directly at temperatures >60°C since the protein breakdown has already been completed by the maltster.
Filtering/lautering (iii)
During this step, the insoluble fraction (spent grains) is separated from the soluble extract. The remaining extract (wort) is transferred to the boiling vessel.
Boiling (iv)
During boiling, hops and other spices are added. These contribute to bitterness and aroma of the final beer. More specifically, hops contain alpha acids and during boiling, these acids will isomerize into iso‐alpha acids, the major bittering substances in beer.
Bitter hops contain high concentrations of alpha acids (6%‐16%) and are often added at the beginning of the boil. Aroma hops have a high hop oil content (>1%), which contains 200‐250 different compounds that contribute to the characteristic aroma of hops (e.g., myrcene, linalool, and nonenal) (Kunze, 2004 ). Aroma hops are typically only added towards the end of the boil, or in the dry‐hopping of green beer to reduce the stripping of aroma‐active compounds.
Other major effects of wort boiling include protein denaturation and aggregation, concentration of the wort, stripping of off‐flavors such as dimethyl sulfide (DMS), and sanitization of the wort. The boiled wort is then transferred to a whirlpool to remove the aggregated protein and insoluble hop components (hot trub). Finally, the wort is cooled, aerated, and transferred into the fermentor, where yeast is added.
Fermentation (v)
During fermentation, yeast converts fermentable sugars into CO 2 and ethanol. At the same time, hundreds of secondary metabolites that influence the aroma and taste of beer are produced. Variation in these metabolites across different yeast strains is what allows yeast to so uniquely influence beer flavor. Examples of typical yeast‐derived beer flavors are the esters isoamylacetate (banana aroma), ethyl acetate (solvent‐like aroma), and ethyl hexanoate (pineapple aroma). For reviews on the different aroma's produced by yeast, and the biochemical pathways involved, see for example (Dzialo, Park, Steensels, Lievens, & Verstrepen, 2017 ; Pires, Teixeira, Branyik, & Vicente, 2014 )
Primary fermentation is typically completed within 10 days, and the end‐product of this fermentation is called green beer. Afterwards, most of the yeast is removed, and the green beer is transferred to a maturation tank and stored at low temperatures (−1°C to 5°C), for several days (ale beers) or up to a couple of weeks (lager beers). During this maturation, the remaining yeast are still metabolically active and can produce additional CO 2 and ethanol as well as reduce off‐flavors such as diacetyl (buttery, rancid aroma).
Many breweries have their own proprietary yeasts for their specific beers. Two main types of yeast are used in brewing: Saccharomyces cerevisiae is a top‐fermenting yeast used to make ales while Saccharomyces pastorianus is a bottom‐fermenting yeast used in lager brewing. The latter is actually a hybrid yeast and combines phenotypes of both its parental species: the high fermentation capacity of S. cerevisiae and the cold tolerance of S. eubayanus (Libkind et al., 2011 ).
Although most breweries use pure yeast cultures for fermentation, spontaneous or mixed fermentation is still used for some specialty beers. These kinds of fermentations usually involve a mix of different yeast species (and bacteria as well) that appear sequentially over time, giving the beer an added complexity. For example, Brettanomyces yeast species are commonly present during the later stages of Belgian lambic beer fermentation (Bokulich, Bamforth, & Mills, 2012 ; Steensels et al., 2015 ). Commonly referred to as Brett by brewers, these yeasts can metabolize longer chain carbohydrates and produce a number of distinctive compounds that provide the characteristic Brett aromas (barnyard, spicy, smoky, metallic etc.).
Since yeast is the driving force behind making beer, this protocol will focus on the yeast side of beer brewing. More specifically, this article describes the different steps in producing your own beer, starting from some basic brewing calculations to yeast propagation and chemical analysis of the end product. We deliberately only included one beer recipe, so you can experiment with how different yeast strains can influence beer aroma. Since the brewing world is full of its own jargon, we have included a glossary of brewing terminology and abbreviations (Table (Table1). 1 ). The methods provided in this protocol are designed to have a minimal equipment requirement and is similar to a small‐scale home brewing approach. Far more advanced brewing setups are available but are often costly and vary greatly in the specifics of usage and are, thus, beyond the scope of this protocol unit. Each of the brewing‐specific materials can be purchased from various suppliers, we have listed some of them in Table Table2 2 .
Glossary of Brewing Terms
Brewing term | Explanation |
---|---|
°P | Degree Plato; unit for the mass percentage of dissolved solids (mostly carbohydrates) in wort. By definition, wort with 1°P has the same density as a solution of 1 g sucrose in 100 g pure water. |
4‐VG | 4‐Vinyl guaiacol; phenolic compound originating from ferulic acid |
Active dried yeast | Dried yeast in granulated form with a residual water content of approx. 5% and >6 × 10 viable cells per gram. Shelf life of 3 months at room temperature without losing viability or up to 36 months at <10°C with decreasing viability. Common suppliers are Lallemand and Fermentis. |
Aeration | Dissolving of oxygen into the cold wort before fermentation. Important for the production of unsaturated fatty acids and sterols needed for yeast membrane production. |
Alpha acids | Class of hop compounds, also known as humulones. Show a low solubility in water but isomerize during the boiling process into water soluble iso‐α‐acids. |
Attenuation | The percentage of original extract consumed during fermentation. The maximum attenuation is determined by wort composition whereas the actual attenuation is largely determined by the yeast strain. |
Base malt | Malt with full enzymatic potential, cell walls, and proteins are well degraded (highly modified malt), starch has not been degraded. Usually constitutes the bulk of the grain bill. |
Brew house yield | Ratio of the extract (kg) in the cast wort and the mass of the malt used (kg) in percent. |
Cast wort | The wort obtained after the boiling process including the hot trub. |
Cold crash | Process in which the green beer is chilled to −1°C to 4°C to facilitate precipitation of cold trub. |
Cold trub (also cold break) | A precipitate of formerly dissolved proteins, polyphenols, and carbohydrates that occurs upon cooling of the boiled wort. |
Dry hopping | Addition of hops to the fermenting wort or green beer. During dry hopping predominantly aroma oils dissolve into the wort, while almost no bitterness is added. |
EBC | European Brewing Congress. The abbreviation EBC is also used as the unit for the color of malts and wort. |
Extract | Total amount of dissolved solids in the brewing water after the mashing process. |
FAN | Free Amino Nitrogen; sum of all amino acids and peptides present in wort which can be utilized by yeast |
Final gravity (FG) | Specific gravity of the beer after fermentation is finished. |
Grain bill | Amount of malt(s) that is needed to brew a beer of desired volume and gravity. |
Gravity | See specific gravity. |
Green beer | Beer at the end of the fermentation, before lagering and maturation. |
Grist | Ground malt. |
Hop utilization% | Degree to which hop alpha acids are isomerized during the boiling process. Depends on the boiling time and wort gravity. |
Hot trub (also hot break) | Insoluble material that occurs due to heat denaturation and coagulation of proteins during wort boiling. Also includes the remaining hop material. |
IBU | International Bittering Unit; 1 IBU = 1 mg/L iso‐alpha acids |
Iso‐alpha acids | Water soluble isomerization products of alpha acids. and form possible, both contribute equally to the bitterness of beer. |
Kilning | Part of the malting process in which the germinated grains are dried and, if necessary, roasted to a desired color. |
Krausen | Foam layer that occurs on ale beers (and to a lesser extent also on lager beers) during fermentation. Contains yeast cells, protein, and hop resins. |
Lautering | Process in which the insoluble fraction of a mash (afterward referred to as spent grain) is separated from the liquid fraction (wort). |
Liquid yeast | Yeast slurry/paste with a high cell density provided in tubes or sachets with 1‐2 × 10 viable cells/ml. Shelf life of 4 months at 4°C. Typical suppliers are White Labs and Wyeast. |
Malt | Cereals in which the enzymatic potential has been released by a process of germination and subsequent heat drying (kilning). |
Malt modification | Modification describes the enzymatic breakdown of biopolymers (mostly proteins and pentosanes) during malting. A highly modified malt is typically suitable for direct mash‐in at amylase rest temperatures without a previous protein rest. |
Maltster | Professional maker of malt from raw cereals by directed germination and kilning processes (see Malt). |
Mash | Mixture of grist and water, held at specific temperature for the enzymatic breakdown of proteins into FAN and of starch into the fermentable sugars that constitute wort. |
Maturation | Also referred to as conditioning. Period after the end of fermentation during which the beer is rounding off its flavor profile, e.g., by reduction of diacetyl by yeast. |
Off‐flavor | Collective term for aroma active compounds which are (usually) undesirable in beer, e.g., diacetyl, DMS, 4‐VG. |
Original gravity (OG) | Specific gravity of the finished wort before fermentation starts. |
Pitching rate | Amount of viable cells used to inoculate the wort. Benchmark for ale fermentations: 0.75 million cells/ml/°P and for lager fermentations: 1.5 million cells/ml/°P |
POF | Phenolic Off Flavor; specific off‐flavor often described as smoky, clove like, sometimes medicinal or burned plastic. Caused by phenolic compounds, most prominently 4‐vinylguaiacol (4‐VG). |
So called “brewer's and baker's” yeast. strains are typically used for ales/top‐fermented beers, e.g., Belgian specialty beers, (India) pale ale, and Hefeweizen. | |
× hybrid used for lager/bottom‐fermented beers. Shows a better cold tolerance than ale yeasts. | |
Sparging | The process of sequential addition of hot water to cast wort from the spent grains during lautering. |
Specialty malt | Malt used to adjust the body or add flavor to a beer, typically no more than 10% of the grain bill. Usually no enzymatic potential, cell walls, proteins, and starch are well degraded, free sugars are caramelized during kilning and subsequent roasting. |
Specific gravity (SG) | Ratio of the density of wort and that of pure water at the same temperature (usually 20°C). |
Spent grains | Solid fraction that remains after the lautering. |
Steeping | First step of the malting process, in which cereals are soaked in water to start germination. |
Trub | Collective term for “hot trub” and “cold trub.” |
Suppliers for Brewing Equipment
Country | Supplier Web site |
---|---|
US | |
UK | |
Germany | |
Belgium |
Please note that it is beyond the scope of this article to give you a detailed overview of all different beer brewing methods and recipes available. For this, we refer readers to, for example Goldammer, 2008 ; Snyder, 1997 , and to the Internet Resources listed at the end of the article.
Basic Protocol 1. PREPARING 20 L OF 12°P WORT STARTING FROM WHOLE GRAINS
Wort production can be broken down into three essential steps − mashing, lautering, and boiling. Making a wort recipe from scratch allows complete control of this process. This can be important if the experiments in question require specific modifications to wort composition. Here we provide a basic wort recipe starting from whole grains that can easily be scaled or modified as needed.
The recipe is for a generic ale beer with 25 international bittering units (IBU) (see also Support Protocol 1 ). It is not designed to mimic a specific beer style but rather produce a lightly hopped wort that can be used as a growth medium for fermentation assays. Individual styles are beyond the scope of this method but there are many resources and software available for developing recipes within individual beer style guidelines, see Internet Resources.
This protocol uses a single temperature infusion mash—a compromise between the different thermal optima of malt enzymes and overall efficiency. For yeast propagation and simple fermentation tests, wort prepared according to Alternate Protocol 1 can also be used.
- 70% (v/v) ethanol
- Pale ale malt
- Bittering hops (such as magnum hops 15% alpha acids)
- 200 ppm zinc stock solution (see recipe)
- Roller mill for grinding malt (optional if buying pre‐ground malts)
- 30‐L stainless steel electric kettle with stainless steel tap for use as mash tun
- Thermometer
- Brewers paddle or large spoon to stir the mash
- 30‐L stainless steel pot with tap and false bottom for use as a lauter tun
- 500‐ml beaker
- 30‐L stainless steel electric kettle with stainless steel tap for use as boil kettle
- Cooling coil with hose tap attachments
- Sterile 20‐L canister
- Four sterile 5‐L canisters
- Additional reagents and equipment for performing an iodine start test (see Support Protocol 4 )
Prepare equipment
Do not use ethanol with additives such as methyl ethyl ketone (MEK), since this is not suitable for human consumption.
See Support Protocol 2 for grain bill calculation.
Properly ground malt should have particles small enough to facilitate efficient hydration. It should also still include unshredded husks to create a stable grain bed during the lautering and prevent a stuck mash (see also Troubleshooting).
See Support Protocol 3 for the calculation of strike water temperature.
- 4 Gently stir in the grist (ground malt produced in step) ensuring that any clumps of malt are broken up.
The mash temperature can be between 63°C and 72°C. Lower temperatures will result in a highly fermentable wort, whereas higher temperatures will increase the amount of non‐fermentable sugars in the wort and can increase mouthfeel and apparent sweetness in the final beer.
See Support Protocol 4 for the iodine starch test.
During this step, residual amylases will be deactivated. The wort's viscosity is also decreased at higher temperatures, which helps in the subsequent lautering. Make sure not to heat above 80°C as this would release undigested starch to the wort.
Make sure to elevate the lauter tun, so that its contents can drain back into the cleaned electric kettle.
- 9 While the mash is settling, heat up 16 L of water in the cleaned mash tun to 78°C for use in the sparge.
The first runnings will be cloudy and full of particulate matter.
- 11 Repeat this process until the wort begins to run clear.
Start draining with a low flow rate, which can be increased as a stable grain bed settles on the false bottom of the mash tun (see also Troubleshooting). A low flow rate is needed to avoid collapse or creation of channels through the grain bed.
- 13 As the mash drains, additional sparge water can be gently added on top of the mash and continually collected as the mash drains into the boil kettle. As the lautering progresses keep track of the wort gravity (see Basic Protocol 2 or Alternate Protocol 2 ).
Note that this percentage can be adapted to match the evaporation rate of your brewing setup in the subsequent boiling step.
- 15 Turn on the kettle heating and bring the wort to a boil.
See Support Protocol 1 for calculating how much hops need to be added to reach a specific IBU.
- 17 Boil for 60 min.
- 18 Attach the sanitized cooling coil to a cold water outlet and place the coil into the brew kettle. Turn on the water and allow to run until the wort has cooled below 25°C.
- 19 Remove the cooling coil and transfer the cooled wort into a 20‐L canister and store overnight at 4°C.
- 20 Decant the wort, leaving as much of the trub behind as possible.
Ideally, the final volume should be 20 L but this will depend on the efficiency of the mashing and lautering procedures, which can be influenced by a number of factors (see Troubleshooting).
If needed, wort can be sterilized by autoclaving for 10 min at 105°C.
- 23 Optional : Centrifuge for 5 min at 1,500‐3,000 × g , 4°C, completely separate any remaining trub.
- 24 Collect the clarified wort in sterile 5 L canisters.
For long‐term storage, the wort can be kept at −20°C (>6 months).
Support Protocol 1. PREDICTING BITTERNESS
Bitterness of beer is measured in international bittering units (IBU), which represent the amount of iso‐alpha acids per liter (quantified as mg/L or ppm). Importantly, the IBU value of a beer may not match its perceived bitterness. The bitterness of a beer can be affected by, among others, the alcohol level, residual sugars or the use of roasted malts.
Estimation of the expected bitterness of a beer can be calculated using the following formula:
This is provided by the hop producer. Alpha acid% greatly depends on hop variety, harvest year, and hop storage conditions.
The hop utilization rate represents the percentage of hop alpha acids that are first solubilized in wort and later isomerized into iso‐alpha acids. This isomerization reaction requires heat and is dependent on the time of hop addition to the boil and the gravity of the wort. In Table Table3, 3 , utilization% is provided for a range of boiling times and wort gravities (Mosher & Finkel, 1993 ).
Utilization%, Adjusted From Mosher & Finkel ( 1993 )
Specific gravity | |||||||
---|---|---|---|---|---|---|---|
Boiling times | 1.030 | 1.040 | 1.050 | 1.060 | 1.070 | 1.080 | 1.090 |
Whole hops | |||||||
5 min | 5% | 5% | 4% | 4% | 3% | 3% | 3% |
15 min | 12% | 12% | 11% | 11% | 11% | 10% | 9% |
30 min | 17% | 17% | 16% | 16% | 15% | 15% | 13% |
45 min | 21% | 21% | 20% | 19% | 18% | 17% | 16% |
60 min | 24% | 23% | 23% | 22% | 21% | 20% | 18% |
90 min | 28% | 27% | 26% | 26% | 25% | 23% | 21% |
Hop pellets | |||||||
5 min | 6% | 6% | 5% | 5% | 4% | 4% | 3% |
15 min | 15% | 15% | 14% | 14% | 13% | 13% | 11% |
30 min | 22% | 21% | 21% | 20% | 19% | 18% | 16% |
45 min | 26% | 26% | 25% | 24% | 23% | 22% | 21% |
60 min | 29% | 28% | 28% | 27% | 26% | 25% | 23% |
90 min | 35% | 34% | 33% | 32% | 31% | 29% | 27% |
- V = volume of cooled wort (L)
Support Protocol 2. DETERMINING THE GRAIN BILL TO ACHIEVE A TARGET EXTRACT AND WORT VOLUME
The grain bill is the amount of malt needed to produce a wort of desired final gravity (FG) and volume. It can be estimated using the following formula
- V [L] = the desired volume of cooled wort at 20°C
Conversion of °P to SG and density at 20°C
°P (g/100 g) | SG | ρ (kg/L) | °P (g/100 g) | SG | ρ (kg/L) | °P (g/100 g) | SG | ρ (kg/L) |
---|---|---|---|---|---|---|---|---|
1 | 1.00388 | 1.00208 | 7.5 | 1.02976 | 1.02792 | 14 | 1.05684 | 1.05495 |
1.5 | 1.00583 | 1.00403 | 8 | 1.03180 | 1.02995 | 14.5 | 1.05898 | 1.05708 |
2 | 1.00779 | 1.00598 | 8.5 | 1.03385 | 1.03199 | 15 | 1.06112 | 1.05922 |
2.5 | 1.00975 | 1.00794 | 9 | 1.03590 | 1.03404 | 15.5 | 1.06327 | 1.06137 |
3 | 1.01172 | 1.00991 | 9.5 | 1.03796 | 1.03610 | 16 | 1.06543 | 1.06352 |
3.5 | 1.01370 | 1.01188 | 10 | 1.04003 | 1.03817 | 16.5 | 1.06760 | 1.06568 |
4 | 1.01568 | 1.01386 | 10.5 | 1.04211 | 1.04024 | 17 | 1.06977 | 1.06785 |
4.5 | 1.01767 | 1.01585 | 11 | 1.04419 | 104232 | 17.5 | 1.07195 | 1.07003 |
5 | 1.01967 | 1.01784 | 11.5 | 1.04628 | 1.04440 | 18 | 1.07414 | 1.07222 |
5.5 | 1.02167 | 1.01984 | 12 | 1.04838 | 1.04650 | 18.5 | 1.07634 | 1.07441 |
6 | 1.02369 | 1.02185 | 12.5 | 1.05048 | 1.04860 | 19 | 1.07855 | 1.07661 |
6.5 | 1.02570 | 1.02386 | 13 | 1.05260 | 1.05071 | 19.5 | 1.08076 | 1.07882 |
7 | 1.02773 | 1.02589 | 13.5 | 1.05472 | 1.05283 | 20 | 1.08298 | 1.08104 |
- °P [% w/w] = the desired wort gravity expressed in degrees Plato
The brewhouse yield displays the relation between the mass of the malt used and the mass of the dissolved extract obtained in the wort (Kunze, 2004 ). It is unique to each brewing setup and recipe and has to be determined empirically. However, a 60% brewhouse yield can be used as a reasonable estimate to calculate the first brew. For more details on brewhouse yield estimation, see Kunze ( 2004 ).
For example, for 20 L of a 12°P wort with a brew house yield of 60%, we need the following amount of malt:
g r a i n b i l l k g = 4.2 k g .
Support Protocol 3. CALCULATE REQUIRED STRIKE WATER TEMPERATURE
The required temperature of the strike water can be determined according to (Holle & Klimovitz, 2003 ):
- GW [kg] = the weight of the added malt/grain
- WW [kg] = the weight of the added strike water
- TM [°C] = wanted final temperature of the mash
- TG [°C] = the temperature of the added malt/grain
Support Protocol 4. IODINE TEST FOR SACCHARIFICATION OF MASH
During mashing, complex carbohydrates need to be hydrolyzed into sugars that can be consumed by yeast, such as glucose, maltose, and maltotriose. This protocol describes how to check if the starch has been sufficiently hydrolyzed.
- Mash solution (see Basic Protocol 1 )
- Lugol's iodine solution (see recipe)
- White porcelain bowl
Avoid any grain particles as these can lead to a false‐positive reaction.
Yellow‐amber red colors indicate complete conversion of starch (Fig. (Fig.1C). 1 C). Blue/black indicates incomplete conversion of starch (Fig. (Fig.1A‐B) 1 A‐B) and the mash should be given an additional 15 min to complete.

Iodine starch test. ( A ) Positive starch result, sample from the beginning of a mash. ( B ) Partial starch conversion mid‐mash. ( C ) Negative starch result, complete starch conversion from the end of a mash.
Alternate Protocol 1. DRIED MALT EXTRACTRECIPE OF 12°P WORT (1 L)
This recipe is ideal for yeast propagation and simple tests (e.g., characterization of the basic fermentation properties of different yeasts). Dried malt extract (DME) wort offers a very reproducible recipe that can be easily prepared without the specialized equipment required for an all grain‐derived wort (see Basic Protocol 1 ). DME, or spray malt, is available in a range of colors and comes in hopped and unhopped varieties. Here we use light unhopped DME, but the recipe can be readily adapted using other DME variants. This recipe can also be used as a concentrated stock if lower gravity wort is required (e.g., for yeast propagation).
- Light unhopped dry malt extract [DME: color: 8 European Brewery Convention (EBC)]
- Deionied water
- Zinc chloride solution at 200 ppm (see recipe)
- 5‐L bottle
- Sterile 500‐ml buckets/bottles to centrifuge wort
- Density meter (e.g., Anton Paar DMA 35)
During autoclaving, proteins precipitate and the wort needs to be clarified afterwards. Because of this, twice the volume is prepared to ensure there is sufficient clarified wort harvested in the end.
Do not autoclave at 121°C as this temperature facilitates maillard reactions between carbohydrates and amino‐compounds.
This will assist protein precipitation and improve the clarity of the final wort.
- 4 Gently decant the wort into sterile 500‐ml centrifuge buckets, leaving as much of the trub behind as possible.
- 5 Centrifuge for 5 min at 1,500‐3,000 × g , 4°C. Collect 1 L of the clarified wort.
- 6 Measure gravity of the wort with the density meter and dilute to 12°P using sterile water if required (see Basic Protocol 2 or Alternate Protocol 2 ).
Zinc is required as a co‐factor for a number of enzymes, such as alcohol dehydrogenase (Kunze, 2004 ), and is commonly added as supplement to wort.
Basic Protocol 2. EXTRACT MEASUREMENT OF WORT USING A HYDROMETER
For brew house calculations, brewers mostly rely on measurements of the wort's or beer's density. Traditionally, wort density is measured using a hydrometer. A hydrometer is a floating body with a thicker bulb for a base, sometimes containing a thermometer, and a thinner upper stem holding a mass percentage (°P) or specific gravity (SG) scale, or both. The value can be determined as the point to which the stem sinks into the fluid. A hydrometer will sink deeper into a sample with lower density and vice versa.
The extract measured before fermentation is called original extract (OE) if measured in °P, or original gravity (OG) if measured as SG. Extract values measured after the fermentation is finished are called residual extract (RE) or FG, respectively.
Here, we give a protocol to determine wort extract using a hydrometer.
- Wort sample (see Basic Protocol or Alternate Protocol 1 )
- 250‐ml glass bottle with screwcap
- Cooling bath at 20°C
- 200‐ml glass cylinder
Close the bottle to avoid evaporation during the cooling process.
Avoid foam on top of the wort sample.
Make sure no gas bubbles adhere to the hydrometer.
Hydrometers for brewing are often calibrated to be read at the highest point of the meniscus which forms around the stem, whereas hydrometers for laboratory use are calibrated to be read at the lowest point of the meniscus. Please check the user manual.
Alternate Protocol 2. EXTRACT MEASUREMENT OF WORT USING AN OSCILLATING U‐TUBE DENSITY METER
A faster and easier way of measuring the wort's extract is using a density meter. The working principle of a density meter is based on an oscillating U‐tube made of glass. The U‐tube gets excited to vibrate at its characteristic frequency. Depending on the density of the tested sample this characteristic frequency changes, which allows calculation of the actual density (DMA 35 Portable Density Meter, Instruction Manual, Anton Paar GmbH, Austria, 2009). This calculation is automatically done by density meter devices for beverage use (e.g., Anton Paar DMA 35).
- Wort sample (see Basic Protocol 1 or Alternate Protocol 1 )
- Warm tap water (40°C)
- Demineralized water
- 100‐ml glass bottle with screwcap
- Plastic syringes
Close the bottle to avoid evaporation.
- 2 Rinse the density meter's U‐tube with your sample before you take the actual measurement. To this end, aspire the liquid into the U‐tube using the built‐in sample tube and pump lever or inject the sample with a plastic syringe. Then dispose of the liquid by depressing the pump lever.
- 3 Take the actual sample in the same way as for the rinsing step; the device will automatically show the density value or any other derived value by choice (e.g., °P or SG).
- 4 Take additional two readings to ensure consistency.
- 5 Rinse the U‐tube with warm tap water followed by a final rinse with demineralized water.
Basic Protocol 3. YEAST PROPAGATION FROM CRYO CULTURES, AGAR PLATES, OR SLANTS
Because of the tremendous effect of yeast‐produced aroma compounds on the quality and character of the final beer, selecting an appropriate yeast is an important and crucial step during brewing. Nowadays, different brewing yeasts are commercially available. These yeast strains are most often supplied as liquid yeast slurries or as active dried yeast powder. For use of these yeast starter cultures, we refer to the manufacturers protocol. Another option is to start from a yeast strain that is stored at −80°C (see Support Protocol 5 ). The protocol below describes how to propagate yeast from such a cryo culture to sufficient cell densities to pitch it into 20 L of a 12°P wort. Using the protocol below, a cell density of ∼2 × 10 8 cells per ml with a viability >95% can usually be obtained. Please check Critical Parameters and Troubleshooting if the cell counts are much lower than expected.
- Yeast strain of choice, as cryo culture or streaked on an agar plate or agar slant
- YPD (2% glucose) agar plate
- Liquid wort (see Alternate Protocol 1 ) or YP maltose (see recipe)
- Sterile wooden sticks (toothpicks work fine) or inoculation loop
- Sterile test tubes
- Shaking incubator
- Test tube rotator
- Sterile Erlenmeyer flasks
This step can be skipped if starting from agar plate or slant.
For lager yeasts, incubate at 20°C rather than 30°C.
Make sure to not thaw and refreeze the cryo culture, since this will significantly reduce cell viability.
Avoid starting with a single colony as this will increase the risk of selecting a colony that may have acquired a deleterious mutation.
Use either wort or YP maltose as starter medium.
If starting from a commercially available yeast strain, check instructions of yeast supplier for exact temperatures to grow your yeast at.
Starter wort gravity is advised to be between 7 and 10°P when brewing a beer with an OE of 10 to 14°P.
Note that using medium containing only glucose as the carbon source is not recommended as the yeast will need time to adapt metabolism to utilize the maltose in wort. The pH of the starter wort should be around 5.
Support Protocol 5. STORING ANY YEAST STRAIN AT −80°C BY MAKING A GLYCEROL STOCK
For the long‐term storage of any yeast strain we recommend glycerol cryo stocks stored at −80°C.
- Dried yeast
- YPD (2% glucose) agar plates
- YPD (2% glucose) liquid medium
- 50% glycerol (sterile)
- Inoculation loop/sterile wooden sticks
- 2‐ml Cryo‐vials (Corning, REF 430659)
- −80°C freezer
When starting from (commercially available) liquid cultures or dried yeast
- 1 Rehydrate dried yeast following the manufacturers protocol.
- 2 Dilute liquid (rehydrated) yeast culture to ∼2‐3 × 10 3 cells/ml (see Basic Protocol 4 ).
For commercially available strains, check the manufacturers protocol for recommended incubation temperatures.
When yeast strains are already streaked on agar plates
- 5 Submerge the inoculation loop/wooden stick in 5 ml YPD liquid medium in test tube and gently shake yeast cells free.
- 7 Transfer 500 µl of the overnight culture into a cryo‐vial.
- 8 Mix gently with the same amount of 50% glycerol (final glycerol concentration 25%).
- 9 Freeze to −80°C until further use.
Basic Protocol 4. ASSESSING YEAST CELL NUMBER AND VIABILITY
Prior to pitching the starter culture, most brewers check cell count and cell viability. One of the most widely used methods in the brewing industry requires a microscope, hemacytometer, and staining with methylene blue or methylene violet (Smart, Chambers, Lambert, Jenkins, & Smart, 1999 ). All yeast cells take up the dye, but only viable cells can reduce it to a colorless product.
This protocol describes how to determine the total number of cells in a liquid culture, as well as the number of viable cells, using a methylene violet staining protocol. Cell counts and viability measurements can also be automated, for example by using a TC20 Automated Cell Counter (Bio‐Rad).
- Deionized water
- Yeast sample
- 0.9% (w/v) NaCl solution or PBS
- 0.1 M glycine buffer, pH 10.6 (adjusted with NaOH)
- Methylene violet solution (0.1% w/v), filter sterilized
- Hemacytometer (Neubauer‐improved type) with cover slips or, alternatively, Kova Glasstic slides (e.g., from FisherScientific)
- Lint‐free paper tissues (e.g., Kimwipes)
- Brightfield microscope (total magnification of 400×)
Prepare the cell count
This step is not required when using Kova Glasstic slides.
Try to have around 100 cells or less per microscope field. Usually a 1:100 or a 1:1000 dilution of your original culture works well. Dilute your samples in 0.9% (w/v) NaCl solution or PBS.
If yeast cells are flocculent, make your dilution using EDTA (50 mM, pH 7.0). This will break up the clumps and will allow you to count the individual cells.
- 3 Mix the sample well by pipetting up and down several times.
- 4 Dilute methylene violet stock solution 1/10 using 0.1 M glycine buffer at pH 10.6.
- 5 Add 1 ml of a methylene violet solution to 1 ml of (diluted) yeast culture.
- 6 Mix the sample well by pipetting up and down several times.
Count the yeast cells
The sample will almost immediately be pulled under the coverslip by capillary action.
When using Kova Glasstic slides, simply pipette 6.6 µl (the maximum volume of each chamber) of the well‐mixed sample onto the slides.
- 8 Place the hemacytometer on the microscopy stage.
- 9 Locate yeast cells and focus the microscope.
If yeast cells are evenly distributed over sample, you can count within the five numbered squares (see Fig. Fig.2 2 ).

A hemacytometer is used to count the number of cells in a suspension. It basically comprises a chamber, created by a grid of perpendicular lines that are carved into glass (upper panel). The area bound by each line, as well as the volume of each chamber that is created in this way, is known. By counting cells in a specific area of the grid (e.g.,, in the five indicated areas in lower panel), it is possible to calculate the original overall cell concentration in the suspension that was pipetted in the chamber.
Be consistent when counting and make sure to establish a counting protocol (e.g., always count cells on upper and right edge, and never on lower and left edge of the squares).
For the Kova Glasstic slides: Count 10 of the small grids.
Dead cells stain violet, viable (or at least metabolically active) cells do not have a color. In general, pale violet cells and budding yeast cells are considered as viable cells.
- 12 Determine fraction of viable cells.
This equation is for a Neubauer‐improved type of hemacytometer.
This calculation assumes you only counted 5 of the in total 25 squares of the counting chamber. Each counted square holds the precise volume of 4 nl. If you counted 5 squares, you have the cell count for a total volume of 20 nl. To determine cell count in 1 ml, multiply by 50,000 (5 × 10 4 ). Please note that volume of counting chamber is given for a Neubauer‐improved hemocytometer. If you are using a different type, please check the manufacturer's instructions on how to determine total cell count. For example, for the Kova Glasstic slides, the volume of each small grid is 0.011 µl; so, if you counted 10 small grids, you have determined the total number of cells in 0.11 µl.
When calculating the dilution factor, take into account that the addition of methylene blue also dilutes your cells twofold.
Basic Protocol 5. BATCH FERMENTATION AND BOTTLING OF BEER (12 L)
This protocol describes how to perform batch fermentations and how to bottle the resulting beer.
- Wort (see Basic Protocol 1 )
- Propagated yeast with sufficiently high cell number (see Basic Protocol 3 )
- Priming sugar (Sigma)
- Sterile 15‐L bottle
- Air pump with 0.2 µm filter attached and sterile tubing (e.g., Eheim 400 air pump)
- Balance (min. 0.1 g resolution)
- Sanitized automatic siphon with bottle filling attachment/bottling wand
- Sanitized/aseptic beer bottles (330 ml)
- Crown capper (with 26‐mm attachment)
- Sanitized crown caps 26 mm
- Incubator that can maintain 20°C
Fermentation of the wort
- 1 Transfer 12 L of 12°P wort into a 15‐L sterile bottle and bring to the desired fermentation temperature.
- 2 Count the yeast cell concentration in the starter culture (see Basic Protocol 4 ).
Alternatively, the starter culture can be centrifuged for 5 min at room temperature, to facilitate this step.
Maintain a sufficiently low flow rate to prevent excessive foaming of the wort.
For the given protocol, this sums up to 108 billion cells and 216 billion cells, respectively.
Avoid temperature shocking the yeast by keeping the starter culture in a range of ±2°C of the wort/fermentation temperature.
The exact fermentation temperature depends on your experimental setup.
Maturation of the beer
Degas samples before measuring gravity (see Support Protocols 6 ‐ 7 ).
Alternatively, fermentation progress can be tracked by monitoring weight loss, using a balance. This process will take around 6 days for an ale and 10 days for a lager.
The 16°C step during maturation of lager beer allows the yeast to reduce the off‐flavor diacetyl (buttery, rancid aroma) to acetoin and, subsequently, 2,3‐butanediol.
During the cold temperature step (also referred to as “cold crash”) yeast cells, as well as cold trub, settle to the bottom of the fermentation vessel, allowing them to be easily separated from the finished beer.
Bottling the beer
The siphon should not be lowered completely to avoid disturbing the sediment layer at the bottom of the fermenter.
Avoid splashing or excessive air contact of the green beer during steps 10 and 11, as this will lead to oxidation of the final beer.
For a standard pale ale beer, a carbonation level of 0.5 g CO 2 /100 g beer is often suggested. For fermentation at 20°C, add 2.37 g of pure glucose per bottle of 330 ml.
See the Internet Resources to calculate the amount of sugar needed for other conditions.
- 13 Place a sanitized crown cap on each bottle and crown bottles following the manufacturer's instructions.
- 14 Allow secondary fermentation in the bottles to complete for 14 days at 20°C.
Basic Protocol 6. SMALL‐SCALE FERMENTATIONS AND SAMPLING
Here we describe a setup for small lab‐scale fermentations that can be useful when comparing a large number of yeast strains or brewing variables. Often it is useful to take samples throughout the fermentation process to measure metabolite production or track fermentation kinetics. Here we describe a setup where samples can be taken while avoiding contamination of the beer (see also Fig. Fig.3). 3 ). Full‐scale industrial fermentations take place under very different physical conditions compared to lab‐scale fermentations. There is a number of ways to mimic the conditions of larger scale fermentations. One method is to simply use stirred fermentations. This protocol is described below.

Schematic of small‐scale fermentation setup in bottles. The 2‐µm filters allow gas escape as CO 2 is produced during fermentation. The septum allows for sampling without risk of contamination.
- Saline solution (0.9% w/v NaCl)
- Dual port GL45 screw caps (e.g., Duran, cat. no. 1129750)
- 12‐mm silicone septa (e.g., Duran, cat. no. 292460503)
- GL14 screw caps with aperture (e.g., Duran, cat. no. 292270508)
- Straight tubing connector for GL14 cap (Duran, cat. no. 292550603)
- Silicone tubing (5 mm internal, 8 mm external diameter)
- 25‐mm PTFE 0.2‐µm filters with female luer lock
- 4‐mm polypropylene hose barbs with male luer lock
- Rubber stopper with airlock
- 30‐mm magnetic stir bars
- Sterile cylinder (250 ml; to transfer wort to bottles)
- Sterile conical centrifugation tubes (50 ml)
- 250‐ml GL45 glass bottles for fermentations (GL45 thread)
- Magnetic stir plates (e.g., IKA RT 15)
- 70‐mm, 20‐G needles with luer adapter
- Syringes with luer adapter
Preparation of the fermentation vessels
- On one port, place a silicone septum and secure it with a GL14 screw cap with aperture.
- To the other port, attach a tubing connector and secure with a GL14 screw cap with aperture.
- Attach 50‐mm silicone tubing to the tubing connector and insert a hose barb into the free end.
If sampling is not required throughout the experiment, a simple rubber stopper with airlock can be used instead. In this case, the stopper and airlock should be sanitized with 70% ethanol prior to use.
- 2 Autoclave bottles with magnetic stir bar and caps for 20 min at 121°C.
This protocol can be scaled up to use glass bottles of any size. Do not use more than 80% of the total volume to avoid over foaming. In some cases, with yeast that ferment heavily or produce large krausen even smaller volumes should be used.
Inoculate the ferementations with yeast
- 4 Prepare a 50 ml yeast starter culture as described in Basic Protocol 3 (steps 1 to 5).
- 5 Transfer the yeast starter into a 50‐ml conical centrifuge tube.
- 6 Centrifuge for 3 min at 1,500‐3,000 × g , room temperature.
- 7 Discard the supernatant.
- 8 Resuspend the cell pellet in 5 ml saline solution and take a cell count (see Basic Protocol 4 ).
The exact pitch rate will depend on your experimental conditions. The given protocol will lead to total pitch rates of 1.35 billion cells for ale yeasts and 2.7 billion cells for lager yeasts.
- 10 Attach caps and place fermentation bottles on magnetic stir plates with stirring at 200 rpm in an incubator set to 20°C.
Monitor and sample the fermentations
The fermentation is complete when the mass of fermentation bottles stays constant.
Sanitize septa with 70% ethanol prior to sampling.
If monitoring fermentation progression by weight loss, be sure to take measurements before and after sampling.
Basic Protocol 7. SPECTROPHOTOMETRIC METHOD TO DETERMINE THE COLOR OF WORT AND BEER
In Europe, the color of a beer is specified in European Brewery Convention (EBC). EBC can be converted to standard reference method (SRM), which is used in the Americas.
EBC can be measured spectrophotometrically at 430 nm wavelength and is defined as:
- d = the dilution factor of the wort/beer sample
- A 430 nm = the measured absorbance at λ ex = 430 nm.
- Wort (from Basic Protocol 1 ) or degassed beer (see Basic Protocols 5 and/or 6 )
- Membrane filter (0.45 µm)
- Syringe membrane filter
- Small plastic vessel to collect filtered samples
- 10‐mm cuvettes
- Spectrophotometer
- 1 Filter 5 ml of wort or degassed beer through a 0.45‐µm membrane filter (see Support Protocol 7 or 8).
- 2 Fill a 10‐mm cuvette with demineralized water, place it in the spectrophotometer, and capture a blank measurement at 430 nm.
Make sure the measured value is within the linear range of the spectrophotometer. In case the measured absorbance is too high, use a dilution of the sample.
- 4 Calculate the EBC value using Equation 6.
Basic Protocol 8. SPECTROPHOTOMETRIC MEASUREMENT OF BITTERNESS
The following protocol describes the procedure to determine the IBU of a sample spectrophotometrically.
- Degassed beer sample (see Basic Protocols 5 and/or 6 )
- 6 M hydrochloric acid
- Iso‐octane for UV spectroscopy (e.g., from Sigma‐Aldrich)
- 50‐ml Erlenmeyer flask with ground glass joint and glass stopper
- Orbital shaker
- UV spectrophotometer
- 1‐cm silica cuvettes
- 1 Transfer 10 ml of a degassed beer sample into a 50‐ml Erlenmeyer flask with ground glass joint. (see Support Protocols 6 ‐ 7 ).
- 2 Add 0.5 ml hydrochloric acid and 20 ml iso‐octane to this sample.
- 3 Close the flask with a glass stopper.
The shaking frequency and duration have to be optimized for the given equipment by repeated measurement of samples from the iso‐octane layer at an absorption wavelength of 275 nm of until no further increase can be detected.
- 5 Measure pure iso‐octane at 275 nm as a blank.
- 6 Measure the absorbance of the iso‐octane layer of each sample at 275 nm.
where A 275 nm = the absorbance of a sample at λ ex = 275 nm.
Basic Protocol 9. APPARENT ATTENUATION OF A FERMENTATION
The degree of attenuation achieved at the end of a fermentation is an important characteristic of the yeast strain used. In contrast to the wort, which can be considered as a mixture of water and dissolved extract, the final beer also includes alcohol. Therefore, only the apparent attenuation can be determined based on density measurements.
The apparent degree of attenuation (ADA) can be determined as follows:
- ADA [%] = apparent degree of attenuation
- RE [°P] = residual extract
- OE [°P] = original extract
Basic Protocol 10. ESTIMATED ALCOHOL CONTENT OF A BEER
Based on the OG of the wort before fermentation and the FG after fermentation, the alcohol content of a beer can be predicted using:
- ABV [% (v/v)] = percentage alcohol by volume
- OG [SG] = original gravity
- FG [SG] = final gravity
For this calculation gravity values in °P have to be converted to SG using Table Table4. 4 . Specialized equipment, such as an Anton Paar Alcolyzer, can be used to measure alcohol content of the final beer.
Basic Protocol 11. GC MEASUREMENT OF VOLATILE ESTERS
During fermentation, yeast produces hundreds of secondary metabolites that influence the aroma and taste of beer. Variation in these metabolites across different yeast strains is what allows yeast to so uniquely influence beer flavor. The protocol below describes how to detect several of the most important yeast‐produced flavor compounds, namely ester compounds.
- Internal standard (10× stock solution of 2‐heptanol = 129.8 ppm in EtOH)
External Standards for GC Measurements
Compound | Aroma | Expected retention time (min) | Stock concentration (µg/L) |
---|---|---|---|
Ethyl acetate | Fruity, sweet | 3.000 | 100 |
Ethyl propionate | Fruity, sweet, rum, juicy, grape, pineapple | 4.070 | 2 |
Propyl acetate | Fruity, solvent, sweet | 4.410 | 1 |
Ethyl isobutyrate | Fruity, sweet, ethereal, alcoholic, fusel | 4.182 | 0.25 |
Isobutyl acetate | Fruity, sweet, tropical | 5.315 | 0.5 |
Ethyl butyrate | Sweet, fruity, pineapple | 5.950 | 2.5 |
Ethyl 2‐methyl butyrate | Green, apple, sweet, fruity, tropical | 6.380 | 0.5 |
Ethyl isovalerate | Sweet, fruity, metallic, green, pineapple | 6.800 | 0.5 |
Isoamyl acetate | Fruity, banana, pear, pine apple | 8.585 | 12.5 |
Pentyl acetate | Fruity, pear, apple | 10.263 | 0.25 |
Ethyl hexanoate (caproate) | Sweet, fruity, pineapple, waxy | 12.138 | 1.25 |
Hexyl acetate | Fruity, green, apple, banana, sweet, apple, pear | 13.440 | 0.25 |
Ethyl octanoate (caprylate) | Fruity, sweet, wine, pineapple, creamy, fatty, mushroom | 18.600 | 5 |
Octyl acetate | Floral, green, earthy, mushroom, herbal, waxy | 19.925 | 0.25 |
Phenetyl acetate | Floral, rose, sweet, honey, tropical, fruity | 30.200 | 3 |
Ethyl decanoate (caprate) | Sweet, fruity, waxy, apple, grape | 24.816 | 2 |
Ethyl valerate | Fruity, sweet, apple, pineapple, green, tropical | 9.000 | 1 |
- NaCl (e.g., from VWR or Sigma‐Aldrich)
- Degassed samples (see Support Protocols 6 ‐ 7 )
- GC vials (Headspace screw neck vials with precision thread, ND18; e.g., VWR International)
- Chemical hood
- Headspace autosampler (PAL system, CTC analytics)
- HS‐GC‐FID‐FPD system (e.g., from Shimadzu)
- Chromatography column (e.g., DB‐WAXETR column (length: 30 m, internal diameter: 0.25 mm, layer thickness: 0.5 mm) from Agilent Technologies)
- Analysis software (e.g., GC solutions software, Shimadzu)
Prepare this in volumetric 10‐ml flasks.
Make sure to properly close stock solutions to prevent evaporation.
Prepare this in a volumetric 10‐ml flask.
Dilutions used to generate standard curve are: 1:2; 1:3; 1:4; 1:8; 1:9; 1:27; 1:81; 1:162
Prepare each dilution in triplicate.
Adding NaCl increases the efficiency of extraction of less volatile compounds in the sample.
Make sure to immediately close and cool each vial to minimize evaporation of volatile compounds.
Dilute the internal standard in water.
The internal standard allows calculation of the actual concentrations of each compound after analysis.
Final concentration of the internal standard in each sample is 2.44 ppm.
For external standards, measure the triplicates of each dilution.
- Temperature split‐splitless injector: 250°C
- Temperature FID: 250°C
- Temperature injection needle: 105°C
- Flow rate carrier gas (N 2 ): 2.6 ml/min
- Sample heating: 70°C for 25 min
Temperature Profile Oven for GC Measurements
Time (min) | Temperature (°C) |
---|---|
0.0 | 50°C |
5.0 | Increase by 4°C/min to a final T of 80°C |
20.0 | Increase by 5°C/min to a final T of 200°C |
44 | 200°C for 3 min |
- 6 Use data acquisition software to acquire chromatograms and interpolate concentrations of esters in samples from standard curve.
Basic Protocol 12. SPECTROPHOTOMETRIC MEASUREMENT OF PHENOLIC OFF‐FLAVORS
During the mashing process, hydroxycinnamic acids such as ferulic acid are released into the wort by hydrolase activity. Decarboxylation of ferulic acid by yeast during fermentation leads to formation of 4‐vinyl guaiacol (4‐VG). 4‐VG has a very distinctive smoky, clove‐like aroma. In most beers, except for particular styles such as Belgian witbier and German hefeweizen, 4‐VG is considered a yeast‐derived off‐flavor; often also referred to as a phenolic off‐flavor (POF).
The protocol below tests the capacity of a yeast strain to convert ferulic acid to 4‐VG (Mertens et al., 2017 ). The method measures absorbance of ferulic acid before and after fermentation as a measure of ferulic acid consumption, inferring 4‐VG production.
Please note that this protocol only tests the capacity of a yeast strain to produce 4‐VG. Whether 4‐VG will actually be produced during fermentation or perceived in the final beer, depends on many additional factors.
- Yeast strain(s) of interest
- POF + control strain: e.g., SafAle WB‐06 (Fermentis)
- POF ‐ control strain: e.g., SafLager W34/70 (Fermentis)
- YPD (see recipe)
- Ferulic acid, 50 g/L (500×) stock concentration in 96% EtOH
- 96‐well plates (e.g., CellStar 96‐well plates, flat bottom)
- Aluminum seals for 96‐well plates (e.g., silverseal aluminum seals, Greiner BioOne)
- Incubator at 30°C
- Plateshaker (e.g., Heidolph Titramax 1000)
- Plate reader (e.g., Tecan Infinity Pro)
Test each strain of interest in biological and technical replicate.
Ferulic acid is added to YPD medium after autoclaving.
Include non‐inoculated wells as blanks.
- 2 Seal the plates with aluminum seal.
- 3 Incubate for 5 days on a shaking platform (900 rpm) in an incubator at 30°C.
- 4 Centrifuge the plates for 3 min at 1,500‐3,000 × g , room temperature.
- 5 Transfer 100 µl of the supernatant into a new 96‐well plate.
Strains are considered POF + if the measured amount of ferulic acid is below the 90% confidence interval of the blank.
Support Protocol 6. DEGASSING OF SAMPLES
Every sample taken during or after the fermentation process needs to be degassed prior further analysis due to the CO 2 produced during fermentation. This is to avoid false results due to gas bubbles adhering to measuring devices (e.g., hydrometer) or emerging during an actual measurement (e.g., density meter). For all protocols handling fermenting wort or beer, this degassing protocol should be followed.
- Sample to degass
- 0.5‐L Erlenmeyer flasks with stopper
- Plastic funnel
- Filter papers (e.g., MACHERY‐NAGEL MN 713 ¼)
- 1 Transfer 150‐200 ml of the sample into a 0.5‐L Erlenmeyer flask.
An overpressure might develop within the flask during this step. Release the pressure carefully by lifting the stopper from time to time.
- 3 Pour the sample through the funnel containing a filter paper. Discard the first 20 ml of flow through and collect the rest into the second Erlenmeyer flask.
- 4 Repeat steps 1 and 2 until the sample appears sufficiently degassed.
Support Protocol 7. DEGASSING OF SMALLER VOLUMES
This protocol can be used when smaller volumes (<5 ml) need to be degassed prior to analyses.
- 20‐ml plastic syringe (sterile)
- Stopper for plastic syringe
- 1 Aspirate ∼5 ml of your fermenting wort or beer using a (sterile) plastic syringe.
- 2 Close the syringe using a stopper
- 3 Pull the lever of the syringe to create an under pressure within the syringe and wait until no more CO 2 degasses from the liquid.
- 4 Release the CO 2 carefully from the syringe without losing any sample volume.
- 5 Repeat steps 1 to 4 until the sample appears sufficiently degassed.
REAGENTS AND SOLUTIONS
Lugol's iodine 1% (w/v).
- Weigh 0.1 g iodine (I 2 ) and 0.2 g potassium iodide (KI).
- Dissolve the solids in 30 ml dH 2 O.
- Store up to 1 year at room temperature
- Weigh 10 g yeast extract and 20 g bactopeptone into a 1‐L bottle
- Add 900 ml of dH 2 O
- Sterilize by autoclaving for 20 min at 121°C
- After autoclaving, add 100 ml of a 20% (w/v) maltose stock solution
- Store up to several weeks at room temperature
- Weigh 10 g yeast extract and 20 g bactopeptone in a 1‐L bottle
- Add 900 ml of dH 2 O
- After autoclaving, add 100 ml of a 20% (w/v) d ‐glucose stock solution
Zinc chloride solution
- Prepare a stock solution of zinc chloride at 200 ppm (417 mg/L ZnCl 2 ).
- Store up to several at room temperature
Background Information
Humans have been brewing beer and other fermented beverages in one way or another since prehistoric times (Barnett, 1998 , 2000 , 2003 ; Hornsey & Royal Society of Chemistry (Great Britain), 2003 ). Initially, the production of beer was a spontaneous, uncontrolled process that relied on microbes that were haphazardly present, for example on the raw materials or instruments used for brewing. Because of this spontaneous process, fermentation results often varied a lot, with big differences in flavor and aroma between different fermentation rounds. To create more consistency and predictability in the quality of the end product, early brewers started using a small fraction of a previously fermented batch to inoculate a new batch; effectively (but unknowingly) recycling the microbes from the previous fermentation round to the next. This practice is called backslopping and promoted adaptation of microbes to the fermentation environment, a process called domestication. This backslopping, and the concomitant domestication, ultimately resulted in the present‐day yeast strains that are used in current breweries (Fay et al., 2019 ; Gallone et al., 2018 ; Gallone et al., 2016 ; Goncalves et al., 2016 ; Hornsey & Royal Society of Chemistry (Great Britain), 2003 ; Liti et al., 2009 ). The process of backslopping is not used often anymore in modern‐day breweries. Instead, brewers preserve pure yeast strains as frozen stock and only recycle their yeast from one fermentation to another for a couple of batches before starting a new culture from their frozen stock.
It was not until 1680 that yeast was discovered by Antonie van Leeuwenhoek, and even later that Pasteur discovered that fermentation was carried out by yeast cells. Many scientific breakthroughs were made during brewing‐related research. For example, in 1883, Emil Christian Hansen, while working at the Carlsberg Laboratory in Copenhagen, developed a method that allowed the isolation of pure yeast cultures (Barnett & Lichtenthaler, 2001 ; Hornsey & Royal Society of Chemistry (Great Britain), 2003 ).
Recently, there is an increasing customer demand for product diversity, both in terms of flavor and alcohol content. Driven by this demand, brewers and researchers are currently investigating the potential of alternative yeast species (both Saccharomyces and non‐ Saccharomyces ) for creating beers with different, more complex flavor profiles as well as the production of flavorful low/no alcohol beers (Gibson et al., 2017 ; Steensels & Verstrepen, 2014 ).
Critical Parameters and Troubleshooting
Stuck mash during lautering.
A stuck mash is a common issue during lautering. Follow the tips below to avoid or solve the problem.
- Grind the malt in a way that the husks are still intact, and the starch body is broken up but not ground too finely. Avoid a high flour percentage.
- Allow the solid to settle down properly before starting the lautering process (∼20‐30 min).
- Do not lauter too fast. It helps to fully open the tap of the lauter tun two to three times in the beginning for a short time (∼2 s) to remove bigger particles from underneath the false bottom. Afterwards open the tap just far enough to get a constant flow of 0.5 L/min.
- If the flow rate starts to decrease, do not open the tap further to compensate. This will most likely just worsen the problem by contracting the filter bed. Instead, lower the flow rate and carefully cut the filter bed with a long knife in a rhombus like pattern. Don't cut all the way down to the false bottom.
- If everything fails and the mash is stuck: Close the tap completely, stir up the filter bed completely, and start all over again.
Yeast cell number is too low after propagation
A number of factors can be checked/adjusted to increase yeast cell number during propagation.
- Make sure to use “fresh” yeast as much as possible: Do not use agar plates or slants older than 4 weeks and re‐streak from a cryo stock.
- Always pick multiple colonies from an agar plate to avoid starting from cells that may have acquired deleterious mutations.
- Aerate larger volumes of propagation medium and stir or shake during incubation to keep the yeast in suspension.
- Some yeast strains grow slower than others. In this case extend the incubation time.
- Incubate each strain at its optimal temperature.
Low yield of brew
If the yield of the brew was low (less wort or less extract than expected) there a number of factors that can be adjusted to compensate for this.
- A finer malt grind can improve the yield. Beware that this can also lead to lautering problems (see above).
- Monitor the pH of your mash. Allow the mash to equilibrate and take the first measurement after 10 min. A typical mash pH should be between 5.2 and 5.5, but depends on the malt and water used. If necessary, adjust the pH by titrating with food grade hydrochloric/lactic acid or sodium hydroxide.
- Allow the trub to settle as much as possible before removing the wort from it.
- If there was more than the expected 20 L of wort then the boil may not have been strong enough. Adjust by boiling more vigorously, increasing the duration or by reducing the sparge water volume.
- Moderately modified malts may require multiple mash temperature steps for complete saccharification, using highly modified malts helps to avoid this.
- Last resort: Increase the amount of malt in the recipe to compensate the low efficiency.
Fermentation stops unexpectedly early
Typically, beer yeast strains reach an ADA of ∼80%, based on the sugar composition of the wort. Check the following points if the fermentation stops with an unexpectedly low ADA.
- Check if the yeast strain is able to ferment maltotriose. Strains unable to ferment this trisaccharide typically yield ADA around 60%.
Streaking a strain on YP maltotriose plates and checking for growth is a quick test to see if strains can use maltotriose.
- If propagating yeast from a cryo culture; make sure to aerate both the propagation medium and the wort before fermentation. Insufficient aeration limits cell membrane production can lead to unhealthy yeast.
- Increasing fermentation temperature towards the end of the fermentation by 2°C can support the yeast to completely finish fermentation.
- A lack of FAN can also lead to a stuck fermentation. Moderately modified malts may require a protease rest while mashing to release enough FAN to the wort.
Off‐flavors are present in final beer
See Table Table7 7 .
Off‐Flavors
Off‐flavor | Aroma | Potential cause | Possible solution |
---|---|---|---|
4‐VG | Cloves, smoky, spicy | Contamination of the fermentation with POF yeast | After fermentation, clean all brewing equipment thoroughly. Check under microscope for potential contamination of starter culture |
DMS | Cooked corn, cabbage | Boiling was too short or not thorough enough. Cooling took too long. | Increase boiling time or vigor. Cool hot wort as fast as possible. Do not cover pot during the boil. |
Diacetyl | Buttery, rancid | Maturation was not long enough | Increase time and temperature of maturation |
Trans‐2‐nonenal | Cardboard, stale, oxidized | Oxidation of the finished beer | Minimize exposure of the finished beer to oxygen, especially while bottling. Keep the finished beer dark and cold. |
Acetaldehyde | Fruity, green apple, sharp | Overpitching yeast. Some yeast strains produce more acetaldehyde. Maturation not long enough. | Increase time of maturation to give yeast sufficient time to reduce acetaldehyde to ethanol. |
H S | Rotten egg, foul | FAN content of wort too low | Increase time of maturation, to allow H S to evaporate. Can be stripped by bubbling CO through the green beer. |
Acetic acid | Vinegar, sharp, sour | Contamination of wort or beer with bacteria (e.g., acetobacter) in combination with too high oxygen content. | Clean equipment thoroughly. Minimize oxygen exposure of the finished beer. |
Understanding Results
Production of wort and fermentation with any beer yeast strain.
The basic protocols produce 20 L of a wort with 12°P. The wort can subsequently be used for two purposes as described here: (i) Batch fermentation of the wort using a single yeast strain with the aim to bottle beer for later use, or (ii) small‐scale fermentation experiments, which allow monitoring the fermentation characteristics of multiple yeast strains at a time. Using the calculations and techniques given in these protocols, up or downscaling of the process is possible. Please check the Critical Parameters and Troubleshooting section if unanticipated results occur.
Measurement of beer/fermentation characteristics
Basic Protocols 8 to 12 enable the reader to determine several standard characteristics of beer (color, bitterness, alcohol content, apparent attenuation, and volatile composition). All of these characteristics are influenced by multiple parameters of the brewing process, e.g., malt composition, fermentation temperature, hop variety, and yeast. Using controlled conditions, a standard wort, and consistent fermentation conditions, these measurements can be used to characterize a given yeast strain. These measurements can be further used, for example, to check if the resulting beer falls within specific style guidelines.
Table Table8 8 lists values for typical color, bitterness, alcohol, and attenuation values for a few common beer styles, which can be used for comparison (Brücklmeier, 2018 ).
Values for Typical Color, Bitterness, ABV, and Attenuation Values for Some Common Beer Styles (Brücklmeier, 2018 )
Style | Fermentation | Color (EBC) | Bitterness (IBU) | ABV [% (v/v)] | Attenuation [%] |
---|---|---|---|---|---|
Bavarian Helles | Lager | 8 to 12 | 16 to 25 | 5.0 to 6.0 | 76 to 82 |
Bohemian Pilsner | Lager | 5 to 15 | 30 to 45 | 4.0 to 5.5 | 64 to 68 |
Dubbel | Ale | 30 to 75 | 20 to 35 | 6.0 to 8.0 | ∼79 |
Pale ale | Ale | 10 to 25 | 20 to 40 | 4.0 to 5.5 | 71 to 80 |
GC‐based analysis
The proposed gas chromatography method explained in Basic Protocol 11 allows to determine concentrations of 17 different yeast related aroma active compounds. If the compounds are present in beer below the detection limit of the used setup, they are often represented as “not detected” (N.D.). Table Table9 9 contains data of these aroma compounds for two “standard” types of beer, as well as the concentration ranges in beer reported for each of these compounds, found in literature (Chemists).
Expected Concentrations of Aroma Active Yeast Metabolites a
Compound | Reported concentration range (PPM) | Example standard American ale (mg/L) | Example standard lager (mg/L) |
---|---|---|---|
Ethyl acetate | 5‐50 | 21.89 | 17.98 |
Ethyl propionate | 0.03‐0.2 | 0.06 | 0.05 |
Propyl acetate | 0.01‐0.012 | 0.03 | 0.02 |
Ethyl isobutyrate | 0.002‐0.01 | N.D. | N.D. |
Isobutyl acetate | 0.01‐0.8 | 0.06 | 0.03 |
Ethyl butyrate | 0.004‐0.4 | 0.20 | 0.11 |
Ethyl 2‐methyl butyrate | 0.0004‐0.003 | N.D. | N.D. |
Ethyl isovalerate | 0.0006‐0.007 | N.D. | 0.00 |
Isoamyl acetate | 0.3‐8 | 0.93 | 1.10 |
Pentyl acetate | 0.006‐0.015 | 0.01 | 0.01 |
Ethyl hexanoate (caproate) | 0.008‐1.5 | 0.19 | 0.19 |
Hexyl acetate | 0.003‐0.015 | 0.01 | N.D. |
Ethyl octanoate (caprylate) | 0.04‐1.5 | 1.12 | 0.68 |
Octyl acetate | 0.03‐0.05 | N.D. | N.D. |
Phenetyl acetate | 0.03‐1.5 | 0.14 | 0.26 |
Ethyl decanoate (caprate) | 0.01‐1 | 0.28 | 0.53 |
Ethyl valerate | 0.001‐0.01 | N.D. | N.D. |
a In column two, the measured concentration ranges for 17 different yeast‐related aroma active compounds in beer are represented (according to Chemists). As an example, the obtained results of a standard, laboratory‐brewed American ale (OE = 13°P, fermented at 20°C with a commercial American ale yeast) and lager beer (OE = 12°P, fermented at 12°C with a standard lager yeast) are reported.
b Abbreviations: N.D., not detected.
Spectrophotometric measurement of phenolic off‐flavors
Basic Protocol 12 enables the reader to test whether a given yeast strain has the capability of transforming the malt derived compound ferulic acid to 4‐VG. In contrast to the protocols before, this experiment does not include the production of a wort but can be performed in standard YPD medium. Production of POF is undesirable for most beer yeast strains and beer styles, with a few exceptions. In some Belgian specialty beers POF is tolerated and for Wit as well as German Hefeweizen, it is even a characteristic of the style.
Time Considerations
An example brewing experiment, following the recipes and protocols given in this article, would look similar to the following:
- Streak yeast strain from stock (15 min of work, 2 days incubation).
- Prepare wort (1 day initial preparation, 30‐60 min clarification)
- Grow yeast strain to sufficiently high densities and pitch into wort: Pre‐growth (1 day) and propagation (1‐2 days)
- Fermentations: 6 days for ale beer, 10 days for lager beer.
- Maturation: 3 days for ale beer, 12 days for lager beer.
- Bottling: 30‐60 min
- Secondary fermentation in bottle: 14 days.
- Flavor analyses using GC protocol: 53 min/sample.
Acknowledgments
FAT is supported by the 3rd Hydrophobin Chair and ASBC Research Council Grant 2019. KV is supported by a FWO postdoctoral fellowship. Original research in the lab of KV is supported by KU Leuven Program Financing, European Research Council (ERC) Consolidator Grant CoG682009, VIB, European Molecular Biology Organization (EMBO) Young Investigator program, FWO, and VLAIO (Vlaams Agentschap Innoveren en Ondernemen).
Thesseling, F. A. , Bircham, P. W. , Mertens, S. , Voordeckers, K. , & Verstrepen, K. J. (2019). A hands‐on guide to brewing and analyzing beer in the laboratory . Current Protocols in Microbiology , 54 , e91. doi: 10.1002/cpmc.91 [ PMC free article ] [ PubMed ] [ CrossRef ] [ Google Scholar ]
Literature Cited
- Barnett, J. A. (1998). A history of research on yeasts. 1: Work by chemists and biologists 1789–1850 . Yeast , 14 ( 16 ), 1439–1451. doi: 10.1002/(SICI)1097-0061(199812)14:16<1439::AID-YEA339>3.0.CO;2-Z. [ PubMed ] [ CrossRef ] [ Google Scholar ]
- Barnett, J. A. (2000). A history of research on yeasts 2: Louis Pasteur and his contemporaries, 1850‐1880 . Yeast , 16 ( 8 ), 755–771. doi: 10.1002/1097-0061(20000615)16:8<755::AID-YEA587>3.0.CO;2-4. [ PubMed ] [ CrossRef ] [ Google Scholar ]
- Barnett, J. A. (2003). Beginnings of microbiology and biochemistry: The contribution of yeast research . Microbiology , 149 ( Pt 3 ), 557–567. doi: 10.1099/mic.0.26089-0 [ PubMed ] [ CrossRef ] [ Google Scholar ]
- Barnett, J. A. , & Lichtenthaler, F. W. (2001). A history of research on yeasts 3: Emil Fischer, Eduard Buchner and their contemporaries, 1880–1900 . Yeast , 18 ( 4 ), 363–388. doi: 10.1002/1097-0061(20010315)18:4<363::AID-YEA677>3.0.CO;2-R. [ PubMed ] [ CrossRef ] [ Google Scholar ]
- Bokulich, N. A. , Bamforth, C. W. , & Mills, D. A. (2012). Brewhouse‐resident microbiota are responsible for multi‐stage fermentation of American coolship ale . PLoS One , 7 ( 4 ), e35507. doi: 10.1371/journal.pone.0035507 [ PMC free article ] [ PubMed ] [ CrossRef ] [ Google Scholar ]
- Brücklmeier, J. (2018). Bier brauen: Grundlagen, Rohstoffe, Brauprozess . Stuttgart, Germany: Verlag Eugen Ulmer. [ Google Scholar ]
- Chemists, A. S. O. B. , from American Society of Brewing Chemists . Retrieved from http://methods.asbcnet.org/Flavors_Database.aspx
- Dzialo, M. C. , Park, R. , Steensels, J. , Lievens, B. , & Verstrepen, K. J. (2017). Physiology, ecology and industrial applications of aroma formation in yeast . FEMS Microbiology Reviews , 41 ( Suppl_1 ), S95–S128. doi: 10.1093/femsre/fux031. [ PMC free article ] [ PubMed ] [ CrossRef ] [ Google Scholar ]
- Fay, J. C. , Liu, P. , Ong, G. T. , Dunham, M. J. , Cromie, G. A. , Jeffery, E. W. , … Dudley, A. M. (2019). A polyploid admixed origin of beer yeasts derived from European and Asian wine populations . PLoS Biology , 17 ( 3 ), e3000147. doi: 10.1371/journal.pbio.3000147. [ PMC free article ] [ PubMed ] [ CrossRef ] [ Google Scholar ]
- Gallone, B. , Mertens, S. , Gordon, J. L. , Maere, S. , Verstrepen, K. J. , & Steensels, J. (2018). Origins, evolution, domestication and diversity of Saccharomyces beer yeasts . Current Opinion in Biotechnology , 49 , 148–155. doi: 10.1016/j.copbio.2017.08.005. [ PubMed ] [ CrossRef ] [ Google Scholar ]
- Gallone, B. , Steensels, J. , Prahl, T. , Soriaga, L. , Saels, V. , Herrera‐Malaver, B. , … Verstrepen, K. J. (2016). Domestication and divergence of Saccharomyces cerevisiae beer yeasts . Cell , 166 ( 6 ), 1397–1410.e1316. doi: 10.1016/j.cell.2016.08.020 [ PMC free article ] [ PubMed ] [ CrossRef ] [ Google Scholar ]
- Gibson, B. , Geertman, J. A. , Hittinger, C. T. , Krogerus, K. , Libkind, D. , Louis, E. J. , … Sampaio, J. P. (2017). New yeasts‐new brews: Modern approaches to brewing yeast design and development . FEMS Yeast Research , 17 ( 4 ). doi: 10.1093/femsyr/fox038. [ PubMed ] [ CrossRef ] [ Google Scholar ]
- Goldammer, T. (2008). The Brewer's handbook (2nd ed.). Clifton, VA: Apex Publishers. [ Google Scholar ]
- Goncalves, M. , Pontes, A. , Almeida, P. , Barbosa, R. , Serra, M. , Libkind, D. , … Sampaio, J. P. (2016). Distinct domestication trajectories in top‐fermenting beer yeasts and wine yeasts . Current Biology , 26 ( 20 ), 2750–2761. doi: 10.1016/j.cub.2016.08.040. [ PubMed ] [ CrossRef ] [ Google Scholar ]
- Holle, S. R. , & Klimovitz, R. (2003). A Handbook of Basic Brewing Calculations . St. Paul, Minn.: Master Brewers Association of the Americas. [ Google Scholar ]
- Hornsey, I. S. & Royal Society of Chemistry (Great Britain) . (2003). A History of beer and brewing . Cambridge, UK: Royal Society of Chemistry. [ Google Scholar ]
- Kunze, W. (2004). Technology brewing and malting . Berlin, Germany: Versuchs‐ und Lehranstalt für Brauerei. [ Google Scholar ]
- Libkind, D. , Hittinger, C. T. , Valerio, E. , Goncalves, C. , Dover, J. , Johnston, M. , … Sampaio, J. P. (2011). Microbe domestication and the identification of the wild genetic stock of lager‐brewing yeast . Proceedings of the National Academy of Sciences of the United States of America , 108 ( 35 ), 14539–14544. doi: 10.1073/pnas.1105430108. [ PMC free article ] [ PubMed ] [ CrossRef ] [ Google Scholar ]
- Liti, G. , Carter, D. M. , Moses, A. M. , Warringer, J. , Parts, L. , James, S. A. , … Louis, E. J. (2009). Population genomics of domestic and wild yeasts . Nature , 458 ( 7236 ), 337–341. doi: 10.1038/nature07743. [ PMC free article ] [ PubMed ] [ CrossRef ] [ Google Scholar ]
- Mertens, S. , Steensels, J. , Gallone, B. , Souffriau, B. , Malcorps, P. , & Verstrepen, K. J. (2017). Rapid screening method for phenolic off‐flavor (POF) production in yeast . Journal of the American Society of Brewing Chemists , 75 ( 4 ), 15. doi: 10.1094/ASBCJ-2017-4142-01. [ CrossRef ] [ Google Scholar ]
- Mosher, R. , & Finkel, C. (1993). The Brewer's companion: A Source‐Book for the Small‐Scale Brewer . Seattle, WA: Alephenalia Publications. [ Google Scholar ]
- Pires, E. J. , Teixeira, J. A. , Branyik, T. , & Vicente, A. A. (2014). Yeast: The soul of beer's aroma—A review of flavour‐active esters and higher alcohols produced by the brewing yeast . Applied Microbiology and Biotechnology , 98 ( 5 ), 1937–1949. doi: 10.1007/s00253-013-5470-0. [ PubMed ] [ CrossRef ] [ Google Scholar ]
- Smart, K. A. , Chambers, K. M. , Lambert, I. , Jenkins, C. , & Smart, C. A. (1999). Use of methylene violet staining procedures to determine yeast viability and vitality . Journal of the American Society of Brewing Chemists , 57 ( 1 ), 18–23. doi: 10.1094/ASBCJ-57-0018. [ CrossRef ] [ Google Scholar ]
- Snyder, S. (1997). The Brewmaster's Bible: The Gold Standard for Home Brewers . New York, NY: William Morrow Cookbooks. [ Google Scholar ]
- Steensels, J. , Daenen, L. , Malcorps, P. , Derdelinckx, G. , Verachtert, H. , & Verstrepen, K. J. (2015). Brettanomyces yeasts–From spoilage organisms to valuable contributors to industrial fermentations . International Journal of Food Microbiology , 206 , 24–38. doi: 10.1016/j.ijfoodmicro.2015.04.005. [ PubMed ] [ CrossRef ] [ Google Scholar ]
- Steensels, J. , & Verstrepen, K. J. (2014). Taming wild yeast: Potential of conventional and nonconventional yeasts in industrial fermentations . Annual Review of Microbiology , 68 , 61–80. doi: 10.1146/annurev-micro-091213-113025. [ PubMed ] [ CrossRef ] [ Google Scholar ]
Internet Resources
Brew recipe developer software, free of charge.
BeerSmith, commercial Web site.
- http://brewrecipedeveloper.de/?setlang=en
- http://beersmith.com/
- Beersmith.com
- Brewersfriend.com
- Beerandbrewing.com
- Homebrewersassociation.org
Web sites with recipes for different beer types
This database gives an overview of the different aroma compounds in beer including their concentration range in beer.
- http://methods.asbcnet.org/Flavors_Database.aspx
- Interlibrary Loan and Scan & Deliver
- Course Reserves
- Purchase Request
- Collection Development & Maintenance
- Current Negotiations
- Ask a Librarian
- Instructor Support
- Library How-To
- Research Guides
- Research Support
- Study Rooms
- Research Rooms
- Partner Spaces
- Loanable Equipment
- Print, Scan, Copy
- 3D Printers
- Poster Printing
- OSULP Leadership
- Strategic Plan
Beer Research Guide: Past Paper Topics
- Library Research
- Archival Research
- Craft Brewing
- Education/Organizations
- Ingredients
- People/Business
- Places/Eras
- Prohibition
- Styles/Recipes
- Technology/Sustainability
- Scholar Interviews
- Past Paper Topics
Introduction
Students in past HST 417: History of Beer and Brewing classes have worked on a variety of topics. Some examples on this page are broad and in need of focus, but give you an idea of what has been researched and written about in the past.
- NOTE: these are topics, not thesis statements.
Remember to be specific when choosing a topic and writing a thesis statement. Narrow to a place or time frame, compare companies or styles, and make sure there are primary sources to support your research.
- If you are measuring success of a company, change in a demographic, or the impact of a law make sure you have measurements for evaluating. Success might mean more production or more awards won. Change in a demographic might mean fewer 20-25 year old consumers are drinking beer. The impact of Prohibition might have meant an increase in soda factories or closing of family businesses.
- For example, if you are interested in beer advertising, considering narrowing it to changes in beer advertising in American print media in the 1980s. There is a section on this guide to get you started , are print primary sources in the Oregon Hops and Brewing Archives collections, and are digitized newspaper materials in the New York TimesMachine and advertisements in the Digital Public Library of America .
ADVERTISING AND MEDIA Impact of television advertising on midsize or small breweries. Government regulation of beer advertising. Alcohol advertising and adolescent drinking. Music in beer commercials. History of logos, branding, and trademarking of early beer brands.
BIOGRAPHY Fred Eckhardt and his influence on homebrewing / craft beer in Oregon. Joseph Owades and his influence on the creation of light beer. History of the Anheuser-Busch family. Carrie Nation and her work during Prohibition.
BUSINESS History of X Brewing Company. History of consumer boycotts and labor disputes at the Coors Brewing Company.
CHURCH Relationship of religion and beer. Brewing and Catholic Saints. Monastic influence on beer and brewing. Medieval guilds, economics, and the Catholic Church. History of brewing and the Grimbergen Abbey. Prohibition and anti-Catholic sentiment.
CIDER History of Cider making in Oregon. Cider production in America (or England, or Europe). Gender and cider production in Colonial Chesapeake. History of orchard planting and management for cider apples.
CULTURE Pub culture in England. Saloons in American culture during the 19th and early 20th century. Intersection of beer and politics. Indigenous brewing in America (or Mexico). Cultural and economic significance of Viking beer. Comparison of Oktoberfest festivals in America and Germany. Japanese drinking rituals. Frontier alcohol culture: beer in Alaska-Yukon Territory mining towns. Baseball and beer. Study of race or class in brewing industry. Beer production and consumption in the COVID-19 era.
FOOD Beer in cooking. Beer and food pairings.
GENDER Women's role in the Temperance movements. Women in modern craft beer. Women and beer production in ancient eras (e.g., Mesopotamia, Jaihu, China, Egypt). Women, beer, and witches. History of the Pink Boots Society Representation of women in beer advertising in the 1950s. History of Japanese women and sake production.
HEALTH Changes in perceived health benefits of fermented foods or drinks. Hangover cures. Preventative properties of hops compounds.
HOMEBREWING Women and home brewing in Colonial America. Home brewing in the middle ages in England. Historical brewing processes used in modern home brewing.
HOPS Willamette Valley hop growing and the link to growth of craft beer in Oregon. History of hop research in Corvallis, Oregon (OSU, USDA). Impact of the Cascade hop on the popularity of India Pale Ale. History of Chinese hop growers in the Pacific Northwest. Study of the demographics of hop pickers on the West Coast between 1860 and 1920. History of hop research in England. Study of how monks used hops in beer. Pest management in hops / Insect ecology and hops.
IMMIGRATION Impact of German and Austrian immigrants on Mexico’s brewing history. German immigration and the impact on 19th century American beer styles.
INDUSTRY Impact of the Industrial Revolution on brewing in Chicago. Workers and the industrialization of brewing in London. Impact of the three-tier system on microbreweries.
OREGON Change in local media coverage of Oregon beer between the 1980s and the present. Impact of breweries on Bend’s local economy. History of brewing and saloons in Junction City. The Weinhard family and 19th century brewing in Oregon. 19th century German and Swiss immigrant brewers in Portland. History of Great Western Malting Company.
PLACE Study of craft brewing on the West Coast (looking at 2-4 companies or cities). Beer and the revitalization of small towns in the Pacific Northwest. Alaska Brewing Company, Yukon Brewing Company and craft beer in Alaska/Canada. Beer in Asheville, North Carolina. Impact of brewing industry on regional identities in Bohemia and Germany. Beer-making in Scandinavia.
PROHIBITION Adaptation of breweries in New York during Prohibition. Impact of Prohibition on American beer consumption. Key people in the Woman’s Christian Temperance Union and/or Anti-Saloon League. Near beer and/or needle beer. Bootlegging during Prohibition. Prohibition and organized crime in Chicago Prohibition outside of the United States.
STYLE Chicha and maize beers in the South American Andes. Sake in Japan in the 20th century. History of Marzen beers. Development of the taxonomy of beer styles. India Pale Ale in Oregon (see also standard bitter IPA, aromatic IPA, Hazy IPA in New England). Czech brewing styles in America. Porter and early industrial brewing in Britain. Gluten free beer in Africa.
SUSTAINABILITY Use of geothermal energy and other renewable resources in brewing. The influence and impact of sustainability practices at of Hopworks Urban Brewery. Using waste water to brew beer. Sustainability efforts and marketing in the brewing industry.
TECHNOLOGY Evolution of equipment technologies for beer production History of carbonation in beer. Evolution of the beer can. Impact of bottling technology on beer distribution. Artificial refrigeration and American lager beer in the late 19th century. Carlsberg Laboratory and its impact on scientific brewing.
WAR Growth in the Japanese brewing industry after WWII. Canned beer and the Vietnam war. Beer in American soldier’s rations during WWII. Brewing in Germany in World War I and World War II. The growth of Anheuser-Busch after WWII (1945-1980).
- << Previous: Scholar Interviews
- Last Updated: May 13, 2024 2:54 PM
- URL: https://guides.library.oregonstate.edu/beer_research
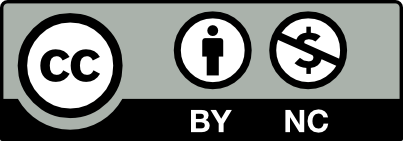
Contact Info
121 The Valley Library Corvallis OR 97331–4501
Phone: 541-737-3331
Services for Persons with Disabilities
In the Valley Library
- Oregon State University Press
- Special Collections and Archives Research Center
- Undergrad Research & Writing Studio
- Graduate Student Commons
- Tutoring Services
- Northwest Art Collection
Digital Projects
- Oregon Explorer
- Oregon Digital
- ScholarsArchive@OSU
- Digital Publishing Initiatives
- Atlas of the Pacific Northwest
- Marilyn Potts Guin Library  
- Cascades Campus Library
- McDowell Library of Vet Medicine

Academia.edu no longer supports Internet Explorer.
To browse Academia.edu and the wider internet faster and more securely, please take a few seconds to upgrade your browser .
- We're Hiring!
- Help Center
Beer consumption trends, craft brewing industry
- Most Cited Papers
- Most Downloaded Papers
- Newest Papers
- Last »
- Craft beer Follow Following
- Beer Follow Following
- Beer and Brewery History Follow Following
- Beer (Alcohol Studies) Follow Following
- Craft Beer Marketing Research Follow Following
- Production of Beer Using New Technology and Yeast with Recent Research Follow Following
- Beer Market Trends Follow Following
- Brewing Follow Following
- Archaeology of Beer and Cereal Fermentation Follow Following
- Cultural history of beer Follow Following
Enter the email address you signed up with and we'll email you a reset link.
- Academia.edu Publishing
- We're Hiring!
- Help Center
- Find new research papers in:
- Health Sciences
- Earth Sciences
- Cognitive Science
- Mathematics
- Computer Science
- Academia ©2024

An official website of the United States government
The .gov means it’s official. Federal government websites often end in .gov or .mil. Before sharing sensitive information, make sure you’re on a federal government site.
The site is secure. The https:// ensures that you are connecting to the official website and that any information you provide is encrypted and transmitted securely.
- Publications
- Account settings
- My Bibliography
- Collections
- Citation manager
Save citation to file
Email citation, add to collections.
- Create a new collection
- Add to an existing collection
Add to My Bibliography
Your saved search, create a file for external citation management software, your rss feed.
- Search in PubMed
- Search in NLM Catalog
- Add to Search
Alcohol research and the alcoholic beverage industry: issues, concerns and conflicts of interest
Affiliation.
- 1 Department of Community Medicine and Health Care, University of Connecticut School of Medicine, Farmington, CT 06030-6325, USA. [email protected]
- PMID: 19133913
- DOI: 10.1111/j.1360-0443.2008.02433.x
Aims: Using terms of justification such as 'corporate social responsibility' and 'partnerships with the public health community', the alcoholic beverage industry (mainly large producers, trade associations and 'social aspects' organizations) funds a variety of scientific activities that involve or overlap with the work of independent scientists. The aim of this paper is to evaluate the ethical, professional and scientific challenges that have emerged from industry involvement in alcohol science.
Method: Source material came from an extensive review of organizational websites, newspaper articles, journal papers, letters to the editor, editorials, books, book chapters and unpublished documents.
Results: Industry involvement in alcohol science was identified in seven areas: (i) sponsorship of research funding organizations; (ii) direct financing of university-based scientists and centers; (iii) studies conducted through contract research organizations; (iv) research conducted by trade organizations and social aspects/public relations organizations; (v) efforts to influence public perceptions of research, research findings and alcohol policies; (vi) publication of scientific documents and support of scientific journals; and (vii) sponsorship of scientific conferences and presentations at conferences.
Conclusion: While industry involvement in research activities is increasing, it constitutes currently a rather small direct investment in scientific research, one that is unlikely to contribute to alcohol science, lead to scientific breakthroughs or reduce the burden of alcohol-related illness. At best, the scientific activities funded by the alcoholic beverage industry provide financial support and small consulting fees for basic and behavioral scientists engaged in alcohol research; at worst, the industry's scientific activities confuse public discussion of health issues and policy options, raise questions about the objectivity of industry-supported alcohol scientists and provide industry with a convenient way to demonstrate 'corporate responsibility' in its attempts to avoid taxation and regulation.
PubMed Disclaimer
Similar articles
- Public health, academic medicine, and the alcohol industry's corporate social responsibility activities. Babor TF, Robaina K. Babor TF, et al. Am J Public Health. 2013 Feb;103(2):206-14. doi: 10.2105/AJPH.2012.300847. Epub 2012 Dec 13. Am J Public Health. 2013. PMID: 23237151 Free PMC article. Review.
- Vested interests in addiction research and policy. Alcohol industry use of social aspect public relations organizations against preventative health measures. Miller PG, de Groot F, McKenzie S, Droste N. Miller PG, et al. Addiction. 2011 Sep;106(9):1560-7. doi: 10.1111/j.1360-0443.2011.03499.x. Epub 2011 Jul 22. Addiction. 2011. PMID: 21781203
- The alcohol industry and public interest science. Stenius K, Babor TF. Stenius K, et al. Addiction. 2010 Feb;105(2):191-8. doi: 10.1111/j.1360-0443.2009.02688.x. Epub 2009 Oct 5. Addiction. 2010. PMID: 19804465
- Funding food science and nutrition research: financial conflicts and scientific integrity. Rowe S, Alexander N, Clydesdale F, Applebaum R, Atkinson S, Black R, Dwyer J, Hentges E, Higley N, Lefevre M, Lupton J, Miller S, Tancredi D, Weaver C, Woteki C, Wedral E; International Life Sciences Institute (ILSI) North America Working Group on Guiding Principles. Rowe S, et al. Nutr Rev. 2009 May;67(5):264-72. doi: 10.1111/j.1753-4887.2009.00188.x. Nutr Rev. 2009. PMID: 19386030 Review.
- Changing conclusions on secondhand smoke in a sudden infant death syndrome review funded by the tobacco industry. Tong EK, England L, Glantz SA. Tong EK, et al. Pediatrics. 2005 Mar;115(3):e356-66. doi: 10.1542/peds.2004-1922. Pediatrics. 2005. PMID: 15741361
- Under the influence: system-level effects of alcohol industry-funded health information organizations. Maani N, Ci van Schalkwyk M, Petticrew M. Maani N, et al. Health Promot Int. 2023 Dec 1;38(6):daad167. doi: 10.1093/heapro/daad167. Health Promot Int. 2023. PMID: 38097395 Free PMC article.
- Qualitative study of states' capacity to support alcohol prevention policies during the COVID-19 pandemic in the USA. Haley SJ, Peddireddy S, El-Harakeh A, Akasreku B, Riibe D. Haley SJ, et al. Drug Alcohol Rev. 2023 Sep;42(6):1358-1374. doi: 10.1111/dar.13714. Epub 2023 Jul 15. Drug Alcohol Rev. 2023. PMID: 37452762 Free PMC article.
- Evolution of the major alcohol companies key global policy vehicle through the prism of tax records 2011-19. Lesch M, McCambridge J. Lesch M, et al. Global Health. 2023 May 25;19(1):34. doi: 10.1186/s12992-023-00933-w. Global Health. 2023. PMID: 37226209 Free PMC article.
- Industry involvement in evidence production for genomic medicine: A bibliometric and funding analysis of decision impact studies. Parker G, Hunter S, Hogarth S, Miller FA. Parker G, et al. PLoS One. 2023 Apr 27;18(4):e0285122. doi: 10.1371/journal.pone.0285122. eCollection 2023. PLoS One. 2023. PMID: 37104298 Free PMC article.
- Distilling the distillers: examining the political activities of the Distilled Spirits Council of the United States. Lesch M, McCambridge J. Lesch M, et al. Global Health. 2023 Mar 29;19(1):22. doi: 10.1186/s12992-023-00923-y. Global Health. 2023. PMID: 36991443 Free PMC article.
Publication types
- Search in MeSH
Related information
- Cited in Books
- PubChem Compound
- PubChem Substance
Grants and funding
- P50 AA03510/AA/NIAAA NIH HHS/United States
LinkOut - more resources
Full text sources.
- Ovid Technologies, Inc.
- MedlinePlus Health Information
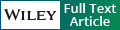
- Citation Manager
NCBI Literature Resources
MeSH PMC Bookshelf Disclaimer
The PubMed wordmark and PubMed logo are registered trademarks of the U.S. Department of Health and Human Services (HHS). Unauthorized use of these marks is strictly prohibited.

- SUGGESTED TOPICS
- The Magazine
- Newsletters
- Managing Yourself
- Managing Teams
- Work-life Balance
- The Big Idea
- Data & Visuals
- Reading Lists
- Case Selections
- HBR Learning
- Topic Feeds
- Account Settings
- Email Preferences
Why Are Companies That Lose Money Still So Successful?
- Vijay Govindarajan,
- Shivaram Rajgopal,
- Anup Srivastava,
- Aneel Iqbal,
- Elnaz Basirian

New research on how to identify investments that produce delayed but real profits — not just those that produce short-term accounting profits.
In a well-functioning capital market, profits should be the sole criterion for firm survival; that is, firms reporting losses should disappear. Of late, however, loss-making firms are highly sought after by investors — often more than some profitable firms. Unicorns, or startups with valuations exceeding a billion dollars, are examples of such loss-making firms. What has changed over time? When and why did losses lose their meaning? The authors’ series of new research papers provide some answers, guiding managers to make the right investments: those that produce delayed but real profits — not just those that produce short-term accounting profits but decimate shareholder wealth in long run.
In 1979, psychologists Daniel Kahneman and Amos Tversky famously posited that losses loom larger than gains in human decision-making. For example, a dollar of loss affects our behavior more than a dollar of profits . Likewise, when a firm announces losses, its stock price declines more dramatically than it increases for the same dollar amount of profits. Investors abandon and lenders tend to stop financing loss-making firms , which then start restructuring their business lines and laying off employees. Some firms go even further, conducting M&A transactions without substance and “managing earnings” to report profits instead of a loss.
- Vijay Govindarajan is the Coxe Distinguished Professor at Dartmouth College’s Tuck School of Business, an executive fellow at Harvard Business School, and faculty partner at the Silicon Valley incubator Mach 49. He is a New York Times and Wall Street Journal bestselling author. His latest book is Fusion Strategy: How Real-Time Data and AI Will Power the Industrial Future . His Harvard Business Review articles “ Engineering Reverse Innovations ” and “ Stop the Innovation Wars ” won McKinsey Awards for best article published in HBR. His HBR articles “ How GE Is Disrupting Itself ” and “ The CEO’s Role in Business Model Reinvention ” are HBR all-time top-50 bestsellers. Follow him on LinkedIn . vgovindarajan
- Shivaram Rajgopal is the Roy Bernard Kester and T.W. Byrnes Professor of Accounting and Auditing and Vice Dean of Research at Columbia Business School. His research examines financial reporting and executive compensation issues and he is widely published in both accounting and finance.
- Anup Srivastava holds Canada Research Chair in Accounting, Decision Making, and Capital Markets and is a full professor at Haskayne School of Business, University of Calgary. In a series of HBR articles, he examines the management implications of digital disruption. He specializes in the valuation and financial reporting challenges of digital companies. Follow Anup on LinkedIn .
- Aneel Iqbal is an assistant professor at Thunderbird School of Global Management, Arizona State University. He examines the accounting measurement and financial disclosures for new-economy firms and incorporates his wide-ranging industry experience into his research and teaching. He is a seasoned accounting and finance professional with diverse experience in auditing, financial analysis, business advisory, performance management, and executive training. Follow Aneel on LinkedIn .
- Elnaz Basirian is a PhD student at the Haskayne School of Business. She examines the influence and role of intangibles in accounting and finance, aimed at improving valuation and market efficiency. She brings a decade of work experience in international financial markets. Follow Elnaz on LinkedIn .
Partner Center

COMMENTS
This boom in craft beer was reflected by the emergence of a large number of research papers that delve into both the demand and supply side. Due to the proliferation of articles, ... (p. 47). Neolocalism manifests itself "in the craft beer industry, mainly through a marketing strategy that uses names and symbols that refer to the locality ...
Beer is produced comm ercially by the controlled fermentation of wort, a liquid-rich in sugars, nitrogenous compounds, sulphur compounds and trace elements extracted from malted barley ...
In recent years, the craft beer (CB) industry has gained impetus and has experienced significant growth in scientific publications. This study aims to present a systematic review of the literature on CB in areas related to economic and business sciences.,Based on the data from Scopus, Web of Science and a set of articles not indexed to these ...
Fig. 2 shows that the field of craft beers is still under-explored (584 papers), but research is increasing exponentially (R 2: 0.8998) with the maximum peak in 2020 (110 papers).A bibliometric study detected that researchers focused more on craft beer science since 2015, but participation is transient (in many cases with only one publication per author), indicating that the field of study is ...
The importance of beer and brewing is indicated in many review papers with topics related to the subject area: demand [8], rice beer -Indian contribution [9], brewing/beer [10], craft beer [11 ...
During the twentieth century, the consolidation of large multi-national beer companies and the homogenization of the specified beer types have led to a considerable growth in the beer industry. However, the growing demand by consumers of a single and distinctive product, with a higher quality and better sensory complexity, is allowing for a new resurgence of craft beer segment in recent years ...
Beer is the most popular alcoholic beverage in the world, and its popularity is continuously growing. Currently, global beer production is estimated at around 2 billion hectoliters. Nevertheless, the increasing production capacity implicates the rising issue of generated by-products—brewers' spent grain, spent hops, spent yeast, and wastewater. They are generated in massive amounts, so ...
José Álvarez-García 3, and Cristiana Oliveira4. Abstract. Beer is popular worldwide and it is considered the oldest and most consumed alcoholic beverage in the world. At the global. production ...
In 2018, Millennials accounted for 11% of our households and craft volume accounted for 12% of beer sold. Therefore, Millennials' intrinsic preferences account for at most 0.7% of the 2018 craft share. Even if every. 16As established in section 6.1, the instruments are not under-powered.
Feature papers represent the most advanced research with significant potential for high impact in the field. A Feature Paper should be a substantial original Article that involves several techniques or approaches, provides an outlook for future research directions and describes possible research applications. ... Beer is a beverage with more ...
Footnote 6 However, despite the considerable volume of work published on this industry (which we discuss in later sections), the future evolution of the brewing industry presents a number of challenging research issues. One topic is the extent to which growing beer consumption in Latin America and Eastern markets will alter the geographic ...
2.1. Introduction. Beer is the most consumed alcoholic beverage in the world (Colen and Swinnen, 2016), yet globalization, economic growth, regulations, and health concerns are important factors affecting the demand for alcoholic beverages and leading toward a worldwide convergence in alcohol consumption patterns in regard to beer, wine, and spirits (Anderson et al., 2018, Colen and Swinnen ...
Finnish beer market, and contrast these discussions with how AI can be used to positively impact the beer brewing process now and in the future. In essence, the research presented here aims to bridge the gap between the beer industry and AI technology. The scope of this thesis will be limited to the Finnish beer market, and the end product of
Beer brewing is intrinsically a biotechnological process: the conversion of raw materials into beer relies on many different enzymatic reactions and microbial activity. ... most brewers check cell count and cell viability. One of the most widely used methods in the brewing industry requires a microscope, hemacytometer, and staining with ...
Call for Papers - Beer Places: The Micro-Geographies of Craft Beer. This will be a book that focuses on landscapes and geographical regions through the story of beer. We are interested in exploring the micro-geographies and micro-cultures of beer and place. Beer helps to tell stories about urban renewal,... more. Download. by Ellis Jones and +1.
The craft beer industry is experiencing continued revenue growth as the larger, mature beer ... traditional beer. Implications of this paper's findings have relevance to local brewing companies, ... Some research even suggests that craft beer tourism could be a form of sustainable tourism (Slocum, 2016). ...
Taking into account the data obtained, it is observed that Scopus overlaps WoS by 88.29% and vice versa by 79.03% in the Craft Beer area. This difference in coverage can be explained by two factors: different indexing policies and differences in the journals included in the database. %TO = 100. WoS ∩ Scopus .
20% of the total beer sales. However, the craft beer industry of South Africa is still in its infancy with an estimated market share of around two per cent of the total beer market. Even though the craft beer industry in South Africa is growing, there are only limited academic research on craft beer breweries in South Africa.
Students in past HST 417: History of Beer and Brewing classes have worked on a variety of topics. Some examples on this page are broad and in need of focus, but give you an idea of what has been researched and written about in the past. NOTE: these are topics, not thesis statements. Remember to be specific when choosing a topic and writing a ...
The brewing industry is undergoing dramatic changes in many countries, with increasing numbers of craft breweries challenging the traditional duo- or oligopolies of major corporations in niche markets. So far, little research has addressed the craft beer industry and this paper is one of the first to actually focus on the breweries and their ...
Consequently, the beer market in India is projected to grow at a CAGR above 7.6% during . African Journal of Hospitality, Tourism and Leisure, Volume 8 (4) - (2019) ISSN: 2223-814X ... young and affluent population is a major contributor to the positive growth outlook of beer market in India (Research and Markets, 2018). Alongside, the culture ...
While industry involvement in research activities is increasing, it constitutes currently a rather small direct investment in scientific research, one that is unlikely to contribute to alcohol science, lead to scientific breakthroughs or reduce the burden of alcohol-related illness. ... The aim of this paper is to evaluate the ethical ...
PEST analysis from the view point of industry following some preliminary research and discussions with key stakeholders in order to: ... The beer market volume is set to rise to 1155 million liters by 2011 an increase of 37.4% from 2006. North India is enjoying beer like never before. During the first quarter of 2006, nearly 5
The authors' series of new research papers provide some answers, guiding managers to make the right investments: those that produce delayed but real profits — not just those that produce short ...