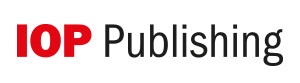

We apologize for the inconvenience...
To ensure we keep this website safe, please can you confirm you are a human by ticking the box below.
If you are unable to complete the above request please contact us using the below link, providing a screenshot of your experience.
https://ioppublishing.org/contacts/
- Artificial Intelligence
- Data Science
- Hardware & Sensors
- Machine Learning
- Agriculture
- Defense & Cyber Security
- Healthcare & Sports
- Hospitality & Retail
- Logistics & Industrial
- Office & Household
- Write for Us

Empowering children through STEAM: A Conversation with Jenny Young, CEO of…
How to secure open-source robotics, autonomous mining technologies (amt) revolutionizing mining, how dental robotics is poised to revolutionize dentistry, how is digital health revolutionizing oncology, why businesses should invest in decentralized apps, a close watch: how uk businesses benefit from advanced cctv systems, empowering small businesses: the role of it support in growth and…, product marketing: leveraging photorealistic product rendering services, everything tech: how technology has evolved and how to keep up….
- Technologies
Additive Manufacturing (AM) – Top research papers
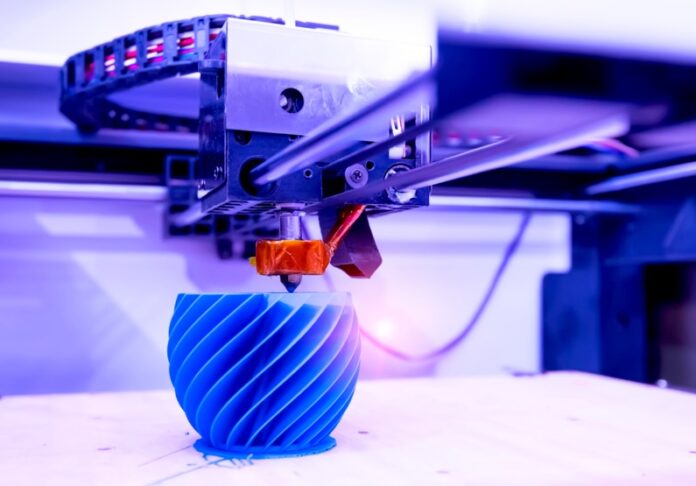
Additive manufacturing (AM) or 3D printing introduces a novel production method in design, manufacturing, and distribution to end-users. This technology provides great freedom in design for creating complex components, highly customizable products, and efficient waste minimization.
Thanks to its numerous benefits, such as time and material saving, rapid prototyping, high efficiency, and decentralized production methods, AM plays a principal role in industry 4.0 that employs the integration of smart manufacturing systems and developed information technologies.
The additive manufacturing technique was first employed by Charles Hull for the stereolithography (SLA) process in 1986. However, over three decades, many other printing methods were discovered and several improvements, transforming the manufacturing and logistics processes. This encouraged the market for more investments in various industries, such as biomedical, aerospace, and automotive.
Notably, there is a significant growth in the investment in AM technology from $4 billion in 2014 to over $21 billion by 2020. AM benefits attract much attention in manufacturing, such as mass-customized production, prototyping, sustainable production, and minimized lead time and cost.
Top research papers on Additive Manufacturing
1. Additive Manufacturing (AM) at Industry 4.0: A Review by Diogo José Horst (2018) – This paper presents the fundamental principles of 3D printing, its roles in industry 4.0 in saving time and cost, and the benefits, e.g., higher flexibility and individualization.
2. Additive manufacturing (3D printing): A review of materials, methods, applications, and challenges by Tuan D. Ngo (2018) – This paper covers the main advantage of additive manufacturing in fast prototyping and its capabilities for producing complex structures, mass customization, freedom of design, and waste minimization. It also explains the industrial revolution of additive manufacturing in aerospace, biomedical, building, and protective structures and the fast transition from conventional machining and traditional methods.
3. Methods and Materials for Smart Manufacturing: Additive Manufacturing, Internet of Things, Flexible Sensors and Soft Robotics by Arkadeep Kumar (2018) – The paper presents various additive manufacturing applications for factories in the future. It also talks about industry 4.0 and smart manufacturing systems using 3D printing and developing and innovation in manufacturing methods and material using additive manufacturing.
4. Advanced Material Strategies for Next-Generation Additive Manufacturing by Jinke Chang (2018) – This paper talks about the application of AM in various fields and industrial productions, e.g., microelectronic and biomedical devices. It also introduces the novel additive manufacturing process for multiple materials, including smart materials, biomaterials, and conductive materials.
5. Additive Manufacturing, Cloud-Based 3D Printing, and Associated Services—Overview by Felix W. Baumann (2017) – This covers the application of Cloud Manufacturing (CM) in the concept of a service-oriented approach over the internet and the historical development in the field of CM and AM in the smart manufacturing process between 2002 to 2006.
6. The role of additive manufacturing in the era of Industry 4.0 by Ugur M Dilberoglu (2017) – This paper covers the recent development of the additive manufacturing process, the benefits of additive manufacturing in design improvement, and industry 4.0. and the current technological methods and highlights in the additive manufacturing process.
7. Smart manufacturing: Characteristics, technologies, and enabling factors by Sameer Mittal (2017) – This paper reviews all published works on various applied technologies and processes related to the smart manufacturing topic and a comprehensive list of the influential factors associated with smart manufacturing and industry 4.0.
8. The rise of 3-D printing: The advantages of additive manufacturing over traditional manufacturing by Mohsen Attaran (2017) – This presents the future of additive manufacturing and identifying the challenges, technologies, and trends, the benefits of additive manufacturing compared with conventional machining and discuss its influence on the supply chain process and the potential of additive manufacturing and impact on the various industry.
9. Industrial Additive Manufacturing: A manufacturing systems perspective by Daniel R. Eyers (2017) – This paper covers the current applications of the additive manufacturing process in the industry, various methods including mechanisms, controls, and activities, and the development in industrial applications and the potentials and opportunities to improve the future of manufacturing.
10. Industrie 4.0 and Smart Manufacturing A Review of Research Issues and Application Examples by Klaus-Dieter Thoben (2017) – This paper presents an overview of smart manufacturing in industry 4.0. It also identifies the current and the future states of technology, besides offering an analysis of cyber-physical systems (CPS) and investigating the potential and applications of this system in production, design, and maintenance processes.
11. Material issues in additive manufacturing: A review by Sunpreet Singh (2017) – This paper presents a review of the biomedical applications of the additive manufacturing process, an introduction to Additive Bio-Manufacturing (ABM) technique for having a safer production, and review the helpful papers on this topic.
12. The evolution and future of manufacturing: A review by Behzad Esmaeilian (2016) – This explores a study of the manufacturing systems and all published works on this topic and the future of manufacturing processes focusing on design development sustainability issues as people, profit, planet.
13. Smart Manufacturing: Past Research, Present Findings, and Future Directions by Hyoung Seok Kang (2016) – This paper analyzes smart manufacturing in the past, current applications, and future by investigating various research papers. It also examines a new paradigm of Information and communications technology (ICT) and manufacturing technologies in industrial revolution 4.0 or smart manufacturing.
14. Additive manufacturing management: a review and future research agenda by Mojtaba khorram (2016) – This covers the multidimensional, systematic, and quantitative analysis to discover the structure of the additive manufacturing process in various scopes, including management, economic, and business. It also investigates the eight principle spectra of the research, including the additive manufacturing process, supply chain management, production design and cost model, strategies challenges, manufacturing systems, sustainability, innovation, and business model.
15. Current Standards Landscape for Smart Manufacturing Systems by Yan Lu (2016) – This report reviews the body of relevant standards upon which future smart manufacturing systems will rely. This report allows manufacturing practitioners to better understand those standards applicable to integrating smart manufacturing technologies. The report concludes that existing manufacturing standards are insufficient to fully enable smart manufacturing, especially in cybersecurity, cloud-based manufacturing services, supply chain integration, and data analytics.
16. Opportunities for Sustainable Manufacturing in Industry 4.0 by Tim Stock (2016) – This presents various sustainability issues in smart manufacturing industry 4.0 and development in sustainable manufacturing and provides solutions in the manufacturing processes.
17. Additive manufacturing and sustainability: an exploratory study of the advantages and challenges by Simon Ford (2016) – This paper covers an overview of advanced manufacturing processes and technologies such as additive manufacturing process, and benefits and challenges of the additive manufacturing process on sustainability issues in terms of business model, value chains, and innovation.
18. The status, challenges, and future of additive manufacturing in engineering by Wei Gao (2015) – This paper shares comprehensive knowledge of the additive manufacturing process, current challenges, achievements and the trend of the future, and the potential of the additive manufacturing process to achieve “print-it-all” image as the primary goal of the AM process shortly.
RELATED ARTICLES MORE FROM AUTHOR
Empowering automation: the revolution of automated charging for agv batteries, automation testing tools for mobile, web, and desktop applications, maintaining regulatory compliance during s/4hana migrations, harnessing the power of performance tv in the digital age, top five benefits of using flutter for app development, the small business owner’s guide to automation in 2024, 7 steps to develop a new app for music lovers, 10 automation tools your devops team can’t live without, robotics process automation (rpa) in insurance.
- Privacy Policy
- Terms & Conditions
Catching additive manufacturing defects with nanoseconds to spare
T he United States is on the cusp of a manufacturing renaissance. Federal legislation has ushered in billions of dollars of investment in manufacturing capabilities that will streamline the supply chain and strengthen national security.
One capability poised to have a profound impact on the nation's industrial base is additive manufacturing, which encompasses a variety of fabrication techniques that build structures layer by layer.
"Additive manufacturing allows you to create so many different structures that are optimized for specific applications," said Vince Pagán, an experimental optics scientist and project manager at the Johns Hopkins Applied Physics Laboratory (APL) in Laurel, Maryland.
"But its Achilles' heel is that the process generates defects that can cause parts to weaken and fail, and you can't have that when they're used in critical applications like national defense, biotechnology and aerospace."
This problem remains one of the most significant obstacles to widespread adoption of additive manufacturing technology, but APL experts are addressing it by developing sensors that are fast enough to identify defects before they materialize.
"If we can identify defect formation while still in the melt state, then we have the opportunity to repair these imperfections before they result in performance-limiting flaws," said Morgan Trexler, who leads APL's Science of Extreme and Multifunctional Materials program. "We are working to make manufacturing processes more intelligent, which will inherently lead to more rapid manufacturing and trusted components."
Bubble trouble
Some of the most common defects created during additive manufacturing—and among the hardest to prevent—are keyhole formations. These appear during the process of powder bed fusion, a method of additive manufacturing that uses lasers to melt metal powders and solidify them into complex geometries.
When the lasers deposit too much energy too quickly into the melted metal or melt pool, tiny bubbles of vapor form and become trapped as the metal cools, weakening a part's structural integrity. Because they form beneath actively printed layers, keyhole defects are hard to spot in real-time—but not impossible.
"We can identify rocks below the surface of rivers from space, not because we can actually see them directly, but because we can see rapids where the water flow is disrupted," explained Steve Storck, project manager and chief scientist for manufacturing technologies in APL's Research and Exploratory Development Department.
"Similarly, if a pore is about to form in a part, then the thermal flow around it will be disrupted, which indicates a defect in the formation process. If we can measure that temperature and spectral anomalies accurately and rapidly, we should be able to tell if something is forming in, underneath or adjacent to the active melting location."
The researchers hypothesized that these keyhole defects were occurring during transitional states. If they could pause the depositing laser just before the anomaly began to form, then the molten metal could cool long enough to settle and close the vapor depression, preventing bubble formation.
"To eliminate keyhole defects, we need to be able to detect and prevent them in real time, but this all happens exceptionally fast," said Storck. "In the additive manufacturing process, solidification happens about 1 to 3,000 times faster than during traditional processes, which means conventional sensing and control methods would not work. This drove us to develop custom methods."
To find out just how long they needed to pause the laser, Li Ma, a senior engineer and additive manufacturing process modeling expert, ran a simulation using computational fluid dynamics. The simulation determined that response times faster than 10 to 20 microseconds were required to identify a thermal disruption, augment the process, and let the molten pool cool slightly without a defect forming.
"This is where the magic happens," said Pagán. "We're essentially slowing down time."
From concept to process
The team first tested conventional sensors to see if they could identify the defect signatures fast enough, but ran into optical resolution and speed limitations. This prompted a collaboration with Mark Foster, an associate professor of electrical and computer engineering with the Johns Hopkins University's Whiting School of Engineering, and several Whiting School postdoctoral students to enhance an APL-developed and patented sensor.
Melding expertise in materials science, additive manufacturing, optical engineering and data science, they added photodiodes at multiple wavelengths and increased the sample frequency to measure high spatial- and temporal-resolution data on the melt pool and its dynamics, enabling them to gather the information needed to identify the early stages of a keyhole defect on a timescale that could enable real-time repair.
With the high-speed sensor up and running, the researchers developed a control framework that could communicate between the sensor and the laser and tell the laser to shut off when the melt pool got too hot and was likely to create a defect—all within 10 to 20 millionths of a second.
Continuing to draw on expertise from across APL, Storck and Pagán reached out to developer Mike Brown, who adapted a high-speed field-programmable gate array—essentially an integrated circuit that can be programmed to meet specific needs—that was originally designed for defense purposes, to seek missiles in the sky.
"One of the unique aspects of working at APL is the ability to leverage technology from areas that seemingly do not relate; for example, we can take the knowledge and expertise we apply to missile defense—responding to measurement inputs very quickly and making adjustments even faster—and apply it to additive manufacturing," said Storck.
After integrating all the systems, the team successfully demonstrated the system's ability to respond in a mere 952 nanoseconds—less than one microsecond, or faster than the blink of an eye.
"Those were great results, because at a minimum we need to measure at least twice as fast as what's actually happening physically, so we can capture the peaks and valleys of the spectral response as it correlates to temperature," Storck explained. "Our system can measure the spectral and temperature readings and respond 10 times faster than required based on simulations of keyhole formation."
The team plans to incorporate artificial intelligence into the process to speed up the feedback loop and more accurately indicate where and how the defects are forming. Storck said this will enable real-time control and repair as APL works toward producing parts that can be trusted straight from the build.
Provided by Johns Hopkins University
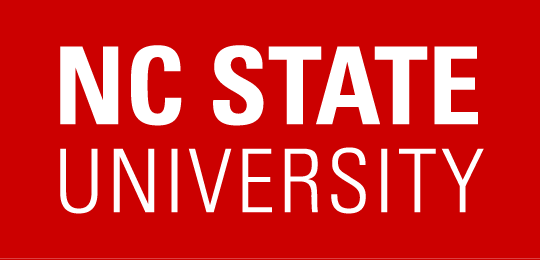
New Technique Improves Finishing Time for 3D-Printed Machine Parts
Original article: New Technique Improves Finishing Time for 3D Printed Machine Parts
Original author: Matt Shipman
North Carolina State University researchers have demonstrated a technique that allows people who manufacture metal machine parts with 3D printing technologies to conduct automated quality control of manufactured parts during the finishing process. The technique allows users to identify potential flaws without having to remove the parts from the manufacturing equipment, making production time more efficient.
“One of the reasons people are attracted to 3D printing and other additive manufacturing technologies is that these technologies allow users to quickly replace critical machine components that are otherwise difficult to make outside of a factory,” says Brandon McConnell, co-corresponding author of a paper on the work. “And additive manufacturing tools can do this as needed, rather than dealing with supply chains that can have long wait times. That usually means using 3D printing to create small batches of machine parts on demand.” McConnell is an assistant research professor in NC State’s Edward P. Fitts Department of Industrial and Systems Engineering.
After a metal machine part is printed, it requires additional finishing and has to be measured to ensure the part meets critical tolerances. In other words, every aspect of the part must be the right size. Currently, that involves taking a part out of the relevant manufacturing equipment, measuring it, and then putting it back into the manufacturing equipment to make modest adjustments.
“This may need to be done repeatedly, and can take a significant amount of time,” McConnell says. “Our work here expedites that process.”
Specifically, the researchers have integrated 3D printing, automated machining, laser scanning and touch-sensitive measurement technologies with related software to create a largely automated system that produces metal machine components that meet critical tolerances.
Here’s how it works.
When end users need a specific part, they pull up a software file that includes the measurements of the desired part. A 3D printer uses this file to print the part, which includes metal support structures. Users then take the printed piece and mount it in a finishing device using the support structure. At this point, lasers scan the mounted part to establish its dimensions. A software program then uses these dimensions and the desired critical tolerances to guide the finishing device, which effectively polishes out any irregularities in the part. As this process moves forward, the finishing device manipulates the orientation of the printed part so that it can be measured by a touch-sensitive robotic probe that ensures the part’s dimensions are within the necessary parameters.
To test the performance of the new approach, researchers manufactured a machine part using conventional 3D printing and finishing techniques, and then manufactured the same part using their new process.
“We were able to finish the part in 200 minutes using conventional techniques; we were able to finish the same part in 133 minutes using our new technique,” McConnell says. “Depending on the situation, saving 67 minutes could be incredibly important. Time is money in most professional settings. And in emergency response contexts, for example, it could be the difference between life and death.”
The researchers note that this work focuses on printing and finishing machine parts that include circles or cylinders, such as pistons. However, the approach could be adapted for machine parts with other features.
“All of the hardware we used in this technique is commercially available, and we outline the necessary software clearly in the paper – so we feel that this new approach could be adopted and put into use almost immediately,” McConnell says. “And we are certainly open to working with partners who are interested in making use of this technique in their operations.”
The paper, “ Automatic Feature Based Inspection and Qualification for Additively Manufactured Parts with Critical Tolerances ,” is published in the International Journal of Manufacturing Technology and Management . First author of the paper is Christopher Kelly, a former graduate student at NC State who now works for Celonis, Inc. The paper was co-authored by Richard Wysk, professor emeritus in the Fitts Department of Industrial and Systems Engineering; Ola Harrysson, Edward P. Fitts Distinguished Professor in the Fitts Department of Industrial and Systems Engineering; and Russell King, the Henry L. Foscue Distinguished Professor of Industrial and Systems Engineering at NC State.
The work was done with support from the U.S. Army Research Office, under grant number W911NF1910055.
“Automatic Feature Based Inspection and Qualification for Additively Manufactured Parts with Critical Tolerances”
Authors : Christopher J. Kelly, Deloitte; Richard A. Wysk, Ola A. Harrysson, Russell E. King and Brandon M. McConnell, North Carolina State University
Published : May 1, International Journal of Manufacturing Technology and Management
DOI : 10.1504/IJMTM.2024.138337
Abstract: This work expands the capabilities of the Digital Additive and Subtractive Hybrid (DASH) system by including “geometric qualification” of mechanical products. Specifically, this research incorporates the extended Additive Manufacturing Format files (AMF-TOL) which include American Society of Mechanical Engineers (ASME) Y14.5 specifications for planes, cylinders and other features so that “in-process” inspection can be completed automatically. An example for the production of holes is provided to illustrate On-Machine-Measurement collects sample radii to estimate the size and position of finished cylindrical features. Statistical analysis was used to measure bounds for comparison to specified tolerance callouts to determine whether a part is within specification, within a user-defined level of confidence. Seven different sampling strategies were evaluated on a DASH part including the bird cage sampling strategy defined in ISO-12180. Part data was utilized to show that for large data samples no statistically significant difference in accuracy was identified for four methods. Finally, analysis shows that using the DASH process with automatic inspection is economically advantageous for low volume production runs.
- About Dr. Mladen Vouk
- Research Committees
- Office of Research and Innovation Fellows
- Stats and Rankings
- Awards and Honors
- Improving Research Infrastructure
- Research Initiatives
- Shared Core Research Facilities
- Interdisciplinary Initiatives
- Centers and Institutes
- Faculty Clusters
- ERA Project
- Institutional Training Grants
- Research Portal
- Partner With Us
- Centennial Campus
- Research Commercialization
- National Security Initiatives
- Research Visitors
- Staff Directory
New Technique Improves Finishing Time for 3D Printed Machine Parts
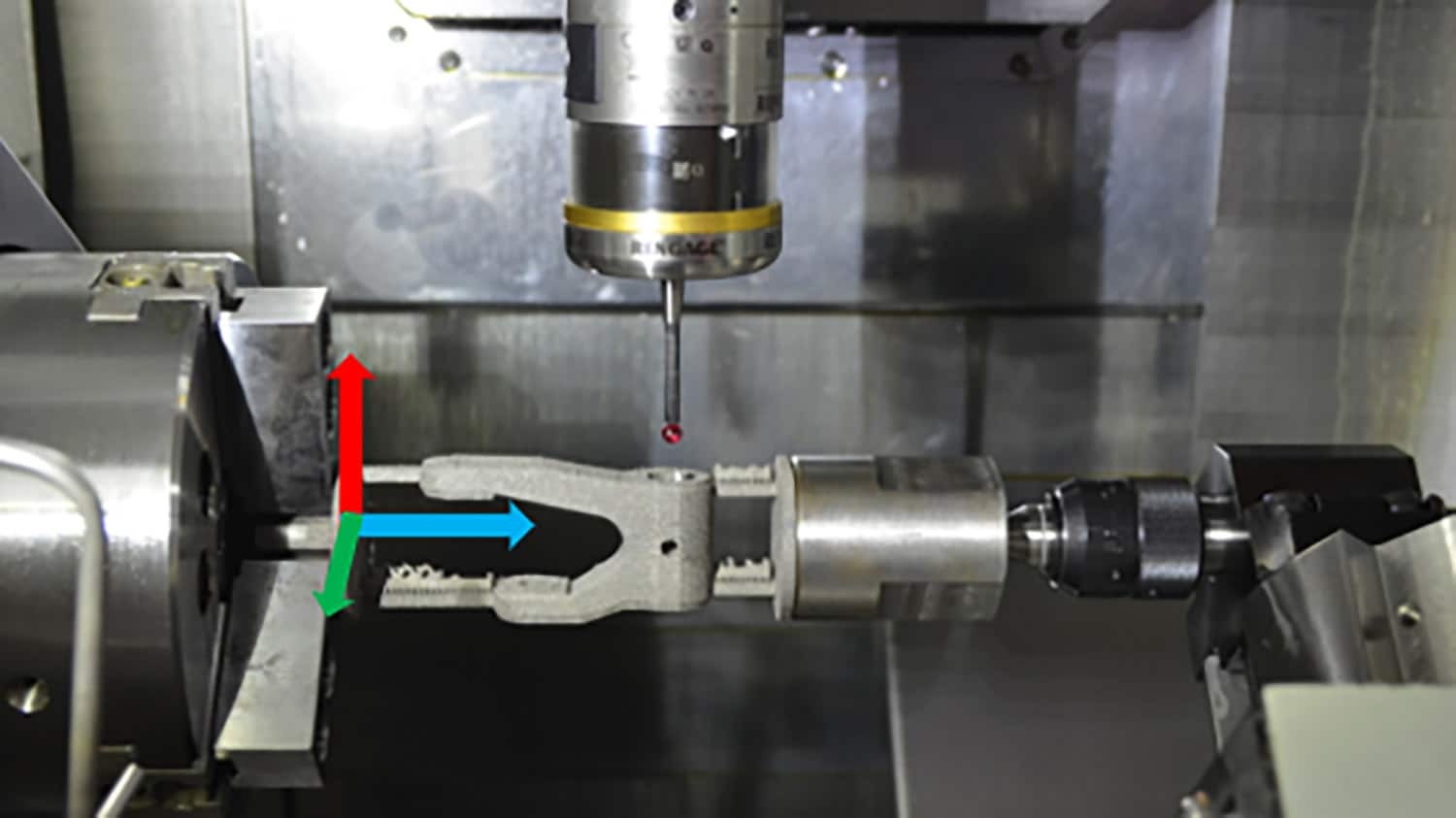
North Carolina State University researchers have demonstrated a technique that allows people who manufacture metal machine parts with 3D printing technologies to conduct automated quality control of manufactured parts during the finishing process. The technique allows users to identify potential flaws without having to remove the parts from the manufacturing equipment, making production time more efficient.
“One of the reasons people are attracted to 3D printing and other additive manufacturing technologies is that these technologies allow users to quickly replace critical machine components that are otherwise difficult to make outside of a factory,” says Brandon McConnell, co-corresponding author of a paper on the work. “And additive manufacturing tools can do this as needed, rather than dealing with supply chains that can have long wait times. That usually means using 3D printing to create small batches of machine parts on demand.” McConnell is an assistant research professor in NC State’s Edward P. Fitts Department of Industrial and Systems Engineering.
After a metal machine part is printed, it requires additional finishing and has to be measured to ensure the part meets critical tolerances. In other words, every aspect of the part must be the right size. Currently, that involves taking a part out of the relevant manufacturing equipment, measuring it, and then putting it back into the manufacturing equipment to make modest adjustments.
“This may need to be done repeatedly, and can take a significant amount of time,” McConnell says. “Our work here expedites that process.”
Specifically, the researchers have integrated 3D printing, automated machining, laser scanning and touch-sensitive measurement technologies with related software to create a largely automated system that produces metal machine components that meet critical tolerances.
Here’s how it works.
When end users need a specific part, they pull up a software file that includes the measurements of the desired part. A 3D printer uses this file to print the part, which includes metal support structures. Users then take the printed piece and mount it in a finishing device using the support structure. At this point, lasers scan the mounted part to establish its dimensions. A software program then uses these dimensions and the desired critical tolerances to guide the finishing device, which effectively polishes out any irregularities in the part. As this process moves forward, the finishing device manipulates the orientation of the printed part so that it can be measured by a touch-sensitive robotic probe that ensures the part’s dimensions are within the necessary parameters.
To test the performance of the new approach, researchers manufactured a machine part using conventional 3D printing and finishing techniques, and then manufactured the same part using their new process.
“We were able to finish the part in 200 minutes using conventional techniques; we were able to finish the same part in 133 minutes using our new technique,” McConnell says. “Depending on the situation, saving 67 minutes could be incredibly important. Time is money in most professional settings. And in emergency response contexts, for example, it could be the difference between life and death.”
The researchers note that this work focuses on printing and finishing machine parts that include circles or cylinders, such as pistons. However, the approach could be adapted for machine parts with other features.
“All of the hardware we used in this technique is commercially available, and we outline the necessary software clearly in the paper – so we feel that this new approach could be adopted and put into use almost immediately,” McConnell says. “And we are certainly open to working with partners who are interested in making use of this technique in their operations.”
The paper, “ Automatic Feature Based Inspection and Qualification for Additively Manufactured Parts with Critical Tolerances ,” is published in the International Journal of Manufacturing Technology and Management . First author of the paper is Christopher Kelly, a former graduate student at NC State who now works for Celonis, Inc. The paper was co-authored by Richard Wysk, professor emeritus in the Fitts Department of Industrial and Systems Engineering; Ola Harrysson, Edward P. Fitts Distinguished Professor in the Fitts Department of Industrial and Systems Engineering; and Russell King, the Henry L. Foscue Distinguished Professor of Industrial and Systems Engineering at NC State.
The work was done with support from the U.S. Army Research Office, under grant number W911NF1910055.
Note to Editors: The study abstract follows.
“Automatic Feature Based Inspection and Qualification for Additively Manufactured Parts with Critical Tolerances”
Authors : Christopher J. Kelly, Deloitte; Richard A. Wysk, Ola A. Harrysson, Russell E. King and Brandon M. McConnell, North Carolina State University
Published : May 1, International Journal of Manufacturing Technology and Management
DOI : 10.1504/IJMTM.2024.138337
Abstract: This work expands the capabilities of the Digital Additive and Subtractive Hybrid (DASH) system by including “geometric qualification” of mechanical products. Specifically, this research incorporates the extended Additive Manufacturing Format files (AMF-TOL) which include American Society of Mechanical Engineers (ASME) Y14.5 specifications for planes, cylinders and other features so that “in-process” inspection can be completed automatically. An example for the production of holes is provided to illustrate On-Machine-Measurement collects sample radii to estimate the size and position of finished cylindrical features. Statistical analysis was used to measure bounds for comparison to specified tolerance callouts to determine whether a part is within specification, within a user-defined level of confidence. Seven different sampling strategies were evaluated on a DASH part including the bird cage sampling strategy defined in ISO-12180. Part data was utilized to show that for large data samples no statistically significant difference in accuracy was identified for four methods. Finally, analysis shows that using the DASH process with automatic inspection is economically advantageous for low volume production runs.
This post was originally published in NC State News.
More From Office of Research and Innovation
Makerspace magic with andrea monteza, new tool pinpoints security fixes in open-source software updates, vr poses privacy risks for kids. a new study finds parents aren’t as worried as they should be..
Advertisement
Research Progress in metal additive manufacturing: Challenges and Opportunities
- Published: 12 December 2023
Cite this article
- Ashish Kumar Srivastava 1 ,
- Ajay Kumar ORCID: orcid.org/0000-0001-7306-1902 2 ,
- Parveen Kumar 3 ,
- Preeti Gautam 4 &
- Namrata Dogra 5
452 Accesses
Explore all metrics
The revolutionary changes in aircraft and automobile industries persuaded the rapid productional technology which introduced additive manufacturing of three-dimensional products. The layer-by-layer development techniques with the uniqueness of producing intricate shapes with negligible wastage of material make it famous for many sectors like defence, automobile, aircraft, medical etc. The present work focuses on different aspects of additive manufacturing especially metal additive manufacturing in terms of their microstructural and morphological aspects. It highlights the different technologies involve in additive manufacturing and discuss the advantages, opportunities and challenges associated with different types of metal additive manufacturing. At last, the present work also highlights the utilization of additive manufacturing techniques in different sectors of use.
This is a preview of subscription content, log in via an institution to check access.

Access this article
Price includes VAT (Russian Federation)
Instant access to the full article PDF.
Rent this article via DeepDyve
Institutional subscriptions

Similar content being viewed by others
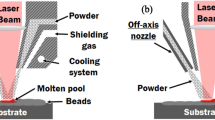
Directed Energy Deposition (DED) Process: State of the Art
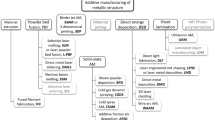
Post-Process Treatments for Additive-Manufactured Metallic Structures: A Comprehensive Review
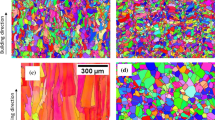
Additive manufacturing of steels: a review of achievements and challenges
Data availability.
The authors declare that the data supporting the findings of this study are available within the paper.
References:
Frazier, W.E.: Metal additive manufacturing: a review. J. Mater. Eng. Perform. 23 , 1917–1928 (2014)
Article Google Scholar
Pidge, P.A., Kumar, H.: Additive manufacturing: a review on 3D printing of metals and study of residual stress, buckling load capacity of strut members. Mater Today Proc. 21 , 1689–1694 (2020)
Ngo, T.D., Kashani, A., Imbalzano, G., Nguyen, K.T.Q., Hui, D.: Additive manufacturing (3D printing): a review of materials, methods, applications and challenges. Compos. B Eng. 143 , 172–196 (2018)
Sehrawat, S., Kumar, A., Prabhakar, M., Nindra, J.: The expanding domains of 3D printing pertaining to the speciality of orthodontics. Mater. Today Proc. 50 , 1611–1618 (2022)
Sehrawat, S., Kumar, A., Grover, S., Dogra, N., Nindra, J., Rathee, S., Dahiya, M., Kumar, A.: Study of 3D scanning technologies and scanners in orthodontics. Mater. Today Proc. 56 , 186–193 (2022)
Kumar, A., Kumar, P., Dogra, N. and Jaglan, A.: Application of incremental sheet forming (ISF) toward biomedical and medical implants. In: Handbook of Flexible and Smart Sheet Forming Techniques: Industry 40 Approaches, Wiley: New Jersey p 247 (2023)
Kumar, A., Kumar, P., Mittal, R.K., Gambhir, V.: Materials processed by additive manufacturing techniques. Adv. Addit. Manuf. 231 , 217–233 (2023)
Google Scholar
Kumar, A., Mittal, R.K., Haleem, A.: Advances in additive manufacturing artificial intelligence. Nature-Insp. Biomanufact. (2023). https://doi.org/10.1016/c2020-0-03877-6
Dumitrescu, G.C., Tanase, I.A.: 3D printing-a new industrial revolution. Knowl. Horiz. Econom. 8 , 32 (2016)
Kumar, P., Kumar, R.K., Mittal, H.S.: Integration of reverse engineering with additive manufacturing. Advances in Additive Manufacturing. doi https://doi.org/10.1016/B978-0-323-91834-3.00028-4
Springer, H., Baron, C., Mostaghimi, F., Poveleit, J., Mädler, L., Uhlenwinkel, V.: Additive manufacturing of high modulus steels: new possibilities for lightweight design. Addit. Manuf. 32 , 101033 (2020)
Buchanan, C., Gardner, L.: Metal 3D printing in construction: a review of methods, research, applications, opportunities and challenges. Eng. Struct. 180 , 332–348 (2019)
Kumar, P., Kumar, R.K., Mittal, H.S.: Printing file formats for additive manufacturing technologies, In (Ed) Advances in Additive Manufacturing, pp.217–233, https://doi.org/10.1016/B978-0-323-91834-3.00006-5
Kumar, A., Kumar, P., Mittal, R.K., Singh, H.: Preprocessing and postprocessing in additive manufacturing, In: Advances in Additive Manufacturing, pp. 217–233. doi https://doi.org/10.1016/B978-0-323-91834-3.00005-3
Javaid, M., Haleem, A.: Current status and applications of additive manufacturing in dentistry: a literature-based review. J Oral Biol Craniofac Res. 9 , 179–185 (2019)
Savolainen, J., Collan, M.: How additive manufacturing technology changes business models?–Review of literature. Addit. Manuf. 32 , 101070 (2020)
Singh, R., Gupta, A., Tripathi, O., Srivastava, S., Singh, B., Awasthi, A., Rajput, S.K., Sonia, P., Singhal, P., Saxena, K.K.: Powder bed fusion process in additive manufacturing: an overview. Mater Today Proc. 26 , 3058–3070 (2020)
Lowke, D., Dini, E., Perrot, A., Weger, D., Gehlen, C., Dillenburger, B.: Particle-bed 3D printing in concrete construction–possibilities and challenges. Cem. Concr. Res. 112 , 50–65 (2018)
Paolini, A., Kollmannsberger, S., Rank, E.: Additive manufacturing in construction: a review on processes, applications, and digital planning methods. Addit. Manuf. 30 , 100894 (2019)
Sames, W.J., List, F.A., Pannala, S., Dehoff, R.R., Babu, S.S.: The metallurgy and processing science of metal additive manufacturing. Int. Mater. Rev. 61 , 315–360 (2016)
Vyas, C., Poologasundarampillai, G., Hoyland, J., Bartolo, P.: 3D printing of biocomposites for osteochondral tissue engineering. In: Biomedical Composites, pp. 261–302. Elsevier, New Jersey (2017)
Chapter Google Scholar
Duda, T., Raghavan, L.V.: 3D metal printing technology. IFAC-PapersOnLine. 49 , 103–110 (2016)
Srivastava, A.K., Nag, A., Dwivedi, S., Dixit, A.R., Hloch, S.: Effect of eggshell powder on the microstructural and thermal behavior of Al7075/waste eggshell surface composites produced by solid-state friction stir processing developed for potential thermal applications. Int. J. Adv. Manuf. Tech. 127 , 1243–1261 (2023)
Hahnlen, R., Dapino, M.J.: NiTi–Al interface strength in ultrasonic additive manufacturing composites. Compos. B Eng. 59 , 101–108 (2014)
Hehr, A., Dapino, M.J.: Interfacial shear strength estimates of NiTi–Al matrix composites fabricated via ultrasonic additive manufacturing. Compos. B Eng. 77 , 199–208 (2015)
Kumar, N., Singh, R.K., Srivastava, A.K., Nag, A., Petru, J., Hloch, S.: Surface modification and parametric optimization of tensile strength of Al6082/SiC/waste material surface composite produced by friction stir processing. Coatings 12 , 1909 (2022)
Srivastava, A.K., Dwivedi, S., Nag, A., Kumar, D., Dixit, A.R., Hloch, S.: Microstructural, mechanical and tribological performance of a magnesium alloy AZ31B/Si3N4/eggshell surface composite produced by solid-state multi-pass friction stir processing. Mater. Chem. Phys. 301 , 127694 (2023)
Raj, R., Dixit, A.R., Singh, S.S., Paul, S.: Print parameter optimization and mechanical deformation analysis of alumina-nanoparticle doped photocurable nanocomposites fabricated using vat-photopolymerization based additive technology. Addit Manuf. 60 , 103201 (2022). https://doi.org/10.1016/j.addma.2022.103201
Medellin, A., Du, W., Miao, G., Zou, J., Pei, Z., Ma, C.: Vat photopolymerization 3d printing of nanocomposites: a literature review. J. Micro-Nano-Manuf. 7 , 031006 (2019)
Taheri, H., Shoaib, M.R.B.M., Koester, L.W., Bigelow, T.A., Collins, P.C., Bond, L.J.: Powder-based additive manufacturing-a review of types of defects, generation mechanisms, detection, property evaluation and metrology. Int. J. Addit. Subtr. Mater. Manuf. 1 , 172–209 (2017)
Saboori, A., Aversa, A., Marchese, G., Biamino, S., Lombardi, M., Fino, P.: Application of directed energy deposition-based additive manufacturing in repair. Appl. Sci. 9 , 3316 (2019)
Raj, R., Dixit, A.R., Łukaszewski, K., Wichniarek, R., Rybarczyk, J., Kuczko, W., Górski, F.: Numerical and experimental mechanical analysis of additively manufactured ankle-foot orthoses. Materials 15 , 6130 (2022)
Buswell, R.A., De Silva, W.R.L., Jones, S.Z., Dirrenberger, J.: 3D printing using concrete extrusion: a roadmap for research. Cem. Concr. Res. 112 , 37–49 (2018)
Raj, R., Dixit, A.R.: direct ink writing of carbon-doped polymeric composite ink: a review on its requirements and applications. 3D Print. Addit. Manuf. 10 , 828–854 (2022). https://doi.org/10.1089/3dp.2021.0209
Le, T.T., Austin, S.A., Lim, S., Buswell, R.A., Gibb, A.G.F., Thorpe, T.: Mix design and fresh properties for high-performance printing concrete. Mater. Struct. 45 , 1221–1232 (2012)
Nerella, V.N., Mechtcherine, V.: Virtual sliding pipe rheometer for estimating pumpability of concrete. Constr. Build. Mater. 170 , 366–377 (2018)
Raj, R., Krishna, S.V.V., Desai, A., Sachin, C., Dixit, A.R.: Print fidelity evaluation of PVA hydrogel using computational fluid dynamics for extrusion dependent 3D printing. Conf. Ser. Mater. Sci. Eng. 1225 , 012009 (2022)
Gülcan, O., Günaydın, K., Tamer, A.: The state of the art of material jetting—a critical review. Polym. (Basel) 13 , 2829 (2021)
Ferrari, A., Baumann, M., Coenen, C., Frank, D., Hennen, L., Moniz, A., Torgersen, H., Torgersen, J., van Bodegom, L., van Duijne, F.: Additive bio-manufacturing: 3D printing for medical recovery and human enhancement: study, European Parliament, (2018)
Challagulla, N.V., Rohatgi, V., Sharma, D., Kumar, R.: Recent developments of nanomaterial applications in additive manufacturing: a brief review. Curr. Opin. Chem. Eng. 28 , 75–82 (2020)
Bandyopadhyay, A., Traxel, K.D.: Invited review article: Metal-additive manufacturing—Modeling strategies for application-optimized designs. Addit. Manuf. 22 , 758–774 (2018)
Lewandowski, J.J., Seifi, M.: Metal additive manufacturing: a review of mechanical properties. Annu. Rev. Mater. Res. 46 , 151–186 (2016)
Hong, D., Chou, D.-T., Velikokhatnyi, O.I., Roy, A., Lee, B., Swink, I., Issaev, I., Kuhn, H.A., Kumta, P.N.: Binder-jetting 3D printing and alloy development of new biodegradable Fe-Mn-Ca/Mg alloys. Acta Biomater. 45 , 375–386 (2016)
Cramer, C.L., Nandwana, P., Yan, J., Evans, S.F., Elliott, A.M., Chinnasamy, C., Paranthaman, M.P.: Binder jet additive manufacturing method to fabricate near net shape crack-free highly dense Fe-6.5 wt.% Si soft magnets. Heliyon 5 , 11 (2019)
Wheat, E., Vlasea, M., Hinebaugh, J., Metcalfe, C.: Sinter structure analysis of titanium structures fabricated via binder jetting additive manufacturing. Mater. Des. 156 , 167–183 (2018)
Salmi, M., Ituarte, I.F., Chekurov, S., Huotilainen, E.: Effect of build orientation in 3D printing production for material extrusion, material jetting, binder jetting, sheet object lamination, vat photopolymerisation, and powder bed fusion. Int. J. Collabor. Enterp. 5 , 218–231 (2016)
Tang, Y., Zhou, Y., Hoff, T., Garon, M., Zhao, Y.F.: Elastic modulus of 316 stainless steel lattice structure fabricated via binder jetting process. Mater. Sci. Technol. 32 , 648–656 (2016)
Lu, S.L., Meenashisundaram, G.K., Wang, P., Nai, S.M.L., Wei, J.: The combined influence of elevated pre-sintering and subsequent bronze infiltration on the microstructures and mechanical properties of 420 stainless steel additively manufactured via binder jet printing. Addit. Manuf. 34 , 101266 (2020)
Miyanaji, H., Yang, L.: Equilibrium saturation in binder jetting additive manufacturing processes: theoretical model vs experimental observations. In: 2016 International Solid Freeform Fabrication Symposium, University of Texas at Austin, (2016)
Bai, Y., Wagner, G., Williams, C.B.: Effect of bimodal powder mixture on powder packing density and sintered density in binder jetting of metals. In: 2015 International Solid Freeform Fabrication Symposium, University of Texas at Austin, (2015)
Kumar, A.Y., Wang, J., Bai, Y., Huxtable, S.T., Williams, C.B.: Impacts of process-induced porosity on material properties of copper made by binder jetting additive manufacturing. Mater. Des. 182 , 108001 (2019)
Do, T., Kwon, P., Shin, C.S.: Process development toward full-density stainless steel parts with binder jetting printing. Int. J. Mach. Tools Manuf 121 , 50–60 (2017)
Stevens, E., Schloder, S., Bono, E., Schmidt, D., Chmielus, M.: Density variation in binder jetting 3D-printed and sintered Ti-6Al-4V. Addit. Manuf. 22 , 746–752 (2018)
Meteyer, S., Xu, X., Perry, N., Zhao, Y.F.: Energy and material flow analysis of binder-jetting additive manufacturing processes. Procedia CIRP 15 , 19–25 (2014)
Jansson, A., Edholm, O.: Scale factor and shrinkage in additive manufacturing using binder jetting, (2016)
Miyanaji, H., Momenzadeh, N., Yang, L.: Effect of printing speed on quality of printed parts in binder jetting process. Addit. Manuf. 20 , 1–10 (2018)
Wang, Y., Zhao, Y.F.: Investigation of sintering shrinkage in binder jetting additive manufacturing process. Procedia Manuf. 10 , 779–790 (2017)
Gibson, I., Rosen, D., Stucker, B., Gibson, I., Rosen, D., Stucker, B.: Directed energy deposition processes, additive manufacturing technologies: 3D Printing. Rapid Prototyp. Direct Digital Manuf.. 25 , 245–268 (2015)
Lek, Y.Z., Wang, C., Shen, X., Chen, Z., Ramamurty, U., Zhou, K.: Additive manufacturing of corrosion-resistant maraging steel M789 by directed energy deposition. Mater. Sci. Eng. A 857 , 144032 (2022)
Dass, A., Moridi, A.: State of the art in directed energy deposition: from additive manufacturing to materials design. Coatings 9 , 418 (2019)
Stender, M.E., Beghini, L.L., Sugar, J.D., Veilleux, M.G., Subia, S.R., Smith, T.R., San Marchi, C.W., Brown, A.A., Dagel, D.J.: A thermal-mechanical finite element workflow for directed energy deposition additive manufacturing process modeling. Addit. Manuf. 21 , 556–566 (2018)
Ertay, D.S., Vlasea, M., Erkorkmaz, K.: Thermomechanical and geometry model for directed energy deposition with 2D/3D toolpaths. Addit. Manuf. 35 , 101294 (2020)
MacDonald, E., Wicker, R.: Multiprocess 3D printing for increasing component functionality. Science 353 , aaf2093 (2016)
Ahmad, N., Packirisamy, G., Dutta, R.: 3D Printing Technology in Nanomedicine. Elsevier, New Jersey (2019)
Huang, Y., Wu, D., Zhao, D., Niu, F., Zhang, H., Yan, S., Ma, G.: Process optimization of melt growth alumina/aluminum titanate composites directed energy deposition: effects of scanning speed. Addit. Manuf. 35 , 101210 (2020)
Feenstra, D.R., Cruz, V., Gao, X., Molotnikov, A., Birbilis, N.: Effect of build height on the properties of large format stainless steel 316L fabricated via directed energy deposition. Addit. Manuf. 34 , 101205 (2020)
Liu, Z., Li, Z., Wang, Q., Wang, Y., Yang, L., Nie, X., Geng, P., Wu, Z., Tang, S., Yang, L.: Multimaterial additive manufacturing manipulator for fabricating magnetoelectric pressure sensors. Sci China Technol Sci. 65 , 2542–2550 (2022)
Li, B., Han, C., Lim, C.W.J., Zhou, K.: Interface formation and deformation behaviors of an additively manufactured nickel-aluminum-bronze/15-5 PH multimaterial via laser-powder directed energy deposition. Mater. Sci. Eng., A 829 , 142101 (2022)
Baykasoğlu, C., Akyildiz, O., Tunay, M., To, A.C.: A process-microstructure finite element simulation framework for predicting phase transformations and microhardness for directed energy deposition of Ti6Al4V. Addit. Manuf. 35 , 101252 (2020)
Yao, X.X., Ge, P., Li, J.Y., Wang, Y.F., Li, T., Liu, W.W., Zhang, Z.: Controlling the solidification process parameters of direct energy deposition additive manufacturing considering laser and powder properties. Comput. Mater. Sci. 182 , 109788 (2020)
Liu, S., Shin, Y.C.: Prediction of 3D microstructure and phase distributions of Ti6Al4V built by the directed energy deposition process via combined multi-physics models. Addit. Manuf. 34 , 101234 (2020)
Xia, Z., Xu, J., Shi, J., Shi, T., Sun, C., Qiu, D.: Microstructure evolution and mechanical properties of reduced activation steel manufactured through laser directed energy deposition. Addit. Manuf. 33 , 101114 (2020)
Liu, Z., Zhang, H.-C., Peng, S., Kim, H., Du, D., Cong, W.: Analytical modeling and experimental validation of powder stream distribution during direct energy deposition. Addit. Manuf. 30 , 100848 (2019)
Xie, L., Guo, H., Song, Y., Liu, C., Wang, Z., Hua, L., Wang, L., Zhang, L.-C.: Effects of electroshock treatment on microstructure evolution and texture distribution of near-β titanium alloy manufactured by directed energy deposition. Mater Charact 161 , 110137 (2020)
Wei, H.L., Liu, F.Q., Liao, W.H., Liu, T.T.: Prediction of spatiotemporal variations of deposit profiles and inter-track voids during laser directed energy deposition. Addit. Manuf. 34 , 101219 (2020)
Svetlizky, D., Zheng, B., Buta, T., Zhou, Y., Golan, O., Breiman, U., Haj-Ali, R., Schoenung, J.M., Lavernia, E.J., Eliaz, N.: Directed energy deposition of Al 5xxx alloy using laser engineered net shaping (LENS®). Mater. Des. 192 , 108763 (2020)
Jinoop, A.N., Kumar, V.A., Paul, C.P., Ranjan, R., Bindra, K.S.: Hot deformation behavior of Hastelloy-X preforms built using directed energy deposition based laser additive manufacturing. Mater. Lett. 270 , 127737 (2020)
Lu, N., Lei, Z., Hu, K., Yu, X., Li, P., Bi, J., Wu, S., Chen, Y.: Hot cracking behavior and mechanism of a third-generation Ni-based single-crystal superalloy during directed energy deposition. Addit. Manuf. 34 , 101228 (2020)
Aversa, A., Saboori, A., Librera, E., de Chirico, M., Biamino, S., Lombardi, M., Fino, P.: The role of directed energy deposition atmosphere mode on the microstructure and mechanical properties of 316L samples. Addit. Manuf. 34 , 101274 (2020)
Ansari, M., Martinez-Marchese, A., Huang, Y., Toyserkani, E.: A mathematical model of laser directed energy deposition for process mapping and geometry prediction of Ti-5553 single-tracks. Materialia (Oxf). 12 , 100710 (2020)
Sun, Z., Guo, W., Li, L.: In-process measurement of melt pool cross-sectional geometry and grain orientation in a laser directed energy deposition additive manufacturing process. Opt. Laser Technol. 129 , 106280 (2020)
Manjunath, B.N., Vinod, A.R., Abhinav, K., Verma, S.K., Sankar, M.R.: Optimisation of process parameters for deposition of colmonoy using directed energy deposition process. Mater Today Proc. 26 , 1108–1112 (2020)
Lin, F., Sun, W.: Warping analysis in laminated object manufacturing process. J. Manuf. Sci. Eng. 123 , 739–746 (2001)
Y. Suping, T. Murakame, N. Nakajima, Accuracy study on laminated object manufacturing for the metallic functional parts with complex surface. In: The Proceedings of 10th Annual Solid Freeform Fabrication Symposium, Austin of Texas, USA, Aug, (1999)
Ahn, D., Kweon, J.-H., Choi, J., Lee, S.: Quantification of surface roughness of parts processed by laminated object manufacturing. J. Mater. Process. Technol. 212 , 339–346 (2012)
Krinitcyn, M., Fu, Z., Harris, J., Kostikov, K., Pribytkov, G.A., Greil, P., Travitzky, N.: Laminated object manufacturing of in-situ synthesized MAX-phase composites. Ceram. Int. 43 , 9241–9245 (2017)
Liu, S., Ye, F., Liu, L., Liu, Q.: Feasibility of preparing of silicon nitride ceramics components by aqueous tape casting in combination with laminated object manufacturing. Mater. Des. 66 , 331–335 (2015)
Das, A., Madras, G., Dasgupta, N., Umarji, A.M.: Binder removal studies in ceramic thick shapes made by laminated object manufacturing. J. Eur. Ceram. Soc. 23 , 1013–1017 (2003)
Zhang, G., Chen, H., Yang, S., Guo, Y., Li, N., Zhou, H., Cao, Y.: Frozen slurry-based laminated object manufacturing to fabricate porous ceramic with oriented lamellar structure. J. Eur. Ceram. Soc. 38 , 4014–4019 (2018)
Shu, X., Wang, R.: Thermal residual solutions of beams, plates and shells due to laminated object manufacturing with gradient cooling. Compos. Struct. 174 , 366–374 (2017)
Guo, H., Gingerich, M.B., Headings, L.M., Hahnlen, R., Dapino, M.J.: Joining of carbon fiber and aluminum using ultrasonic additive manufacturing (UAM). Compos. Struct. 208 , 180–188 (2019)
Friel, R.J., Harris, R.A.: Ultrasonic additive manufacturing–a hybrid production process for novel functional products. Procedia CIRP. 6 , 35–40 (2013)
Kuo, C.-H., Sridharan, N., Han, T., Dapino, M.J., Babu, S.S.: Ultrasonic additive manufacturing of 4130 steel using Ni interlayers. Sci. Technol. Weld. Joining 24 , 382–390 (2019)
Sridharan, N., Wolcott, P., Dapino, M., Babu, S.S.: Microstructure and texture evolution in aluminum and commercially pure titanium dissimilar welds fabricated using ultrasonic additive manufacturing. Scr. Mater. 117 , 1–5 (2016)
Sridharan, N., Gussev, M., Seibert, R., Parish, C., Norfolk, M., Terrani, K., Babu, S.S.: Rationalization of anisotropic mechanical properties of Al-6061 fabricated using ultrasonic additive manufacturing. Acta Mater. 117 , 228–237 (2016)
Sriraman, M.R., Gonser, M., Fujii, H.T., Babu, S.S., Bloss, M.: Thermal transients during processing of materials by very high power ultrasonic additive manufacturing. J. Mater. Process. Technol. 211 , 1650–1657 (2011)
Yan, S., Chen, L., Yob, A., Renshaw, D., Yang, K., Givord, M., Liang, D.: Multifunctional metal matrix composites by friction stir additive manufacturing. J. Mater. Eng. Perform. 31 , 6183–6195 (2022)
Patel, M., Chaudhary, B., Murugesan, J., Jain, N.K.: Additive manufacturing of AA6063-ZrO 2 composite using friction stir surface additive manufacturing. Trans. Indian Inst. Met. 76 , 581–588 (2023)
Shen, Z., Chen, S., Cui, L., Li, D., Liu, X., Hou, W., Chen, H., Sun, Z., Li, W.Y.: Local microstructure evolution and mechanical performance of friction stir additive manufactured 2195 Al-Li alloy. Mater Charact 186 , 111818 (2022)
Jha, K.K., Kesharwani, R., Imam, M.: Microstructural and micro-hardness study on the fabricated Al 5083-O/6061-T6/7075-T6 gradient composite component via a novel route of friction stir additive manufacturing. Mater Today Proc. 56 , 819–825 (2022)
Ardalanniya, A., Nourouzi, S., Aval, H.J.: Fabrication of the laminated Al-Zn-Cup/Al-Zn composite using friction stir additive manufacturing. Mater Today Commun. 27 , 102268 (2021)
He, C., Li, Y., Zhang, Z., Wei, J., Zhao, X.: Investigation on microstructural evolution and property variation along building direction in friction stir additive manufactured Al–Zn–Mg alloy. Mater. Sci. Eng. A 777 , 139035 (2020)
Roodgari, M.R., Jamaati, R., Aval, H.J.: Fabrication of a 2-layer laminated steel composite by friction stir additive manufacturing. J. Manuf. Process. 51 , 110–121 (2020)
Murr, L.E.: Metallurgy principles applied to powder bed fusion 3D printing/additive manufacturing of personalized and optimized metal and alloy biomedical implants: an overview. J. Market. Res. 9 , 1087–1103 (2020)
Phadke, N., Raj, R., Srivastava, A.K., Dwivedi, S., Dixit, A.R.: Modeling and parametric optimization of laser powder bed fusion 3D printing technique using artificial neural network for enhancing dimensional accuracy. Mater Today Proc. 56 , 873–878 (2022)
Goodridge, R., Ziegelmeier, S.: Powder bed fusion of polymers. In: Laser Additive Manufacturing, pp. 181–204. Elsevier, New Jersey (2017)
Sun, S., Brandt, M., Easton, M.: Powder bed fusion processes: an overview. Laser Addit. Manuf. 1 , 55–77 (2017)
Garmendia, X., Chalker, S., Bilton, M., Sutcliffe, C.J., Chalker, P.R.: Microstructure and mechanical properties of Cu-modified AlSi10Mg fabricated by laser-powder bed fusion. Mater. (Oxf). 9 , 100590 (2020)
Morgan, D., Agba, E., Hill, C.: Support structure development and initial results for metal powder bed fusion additive manufacturing. Procedia Manuf. 10 , 819–830 (2017)
Kalms, M., Narita, R., Thomy, C., Vollertsen, F., Bergmann, R.B.: New approach to evaluate 3D laser printed parts in powder bed fusion-based additive manufacturing in-line within closed space. Addit. Manuf. 26 , 161–165 (2019)
Liu, Y., Blunt, L., Zhang, Z., Rahman, H.A., Gao, F., Jiang, X.: In-situ areal inspection of powder bed for electron beam fusion system based on fringe projection profilometry. Addit. Manuf. 31 , 100940 (2020)
Ronneberg, T., Davies, C.M., Hooper, P.A.: Revealing relationships between porosity, microstructure and mechanical properties of laser powder bed fusion 316L stainless steel through heat treatment. Mater. Des. 189 , 108481 (2020)
Queva, A., Guillemot, G., Moriconi, C., Metton, C., Bellet, M.: Numerical study of the impact of vaporisation on melt pool dynamics in laser powder bed fusion-application to IN718 and Ti–6Al–4V. Addit. Manuf. 35 , 101249 (2020)
Bodner, S.C., van De Vorst, L.T.G., Zalesak, J., Todt, J., Keckes, J.F., Maier-Kiener, V., Sartory, B., Schell, N., Hooijmans, J.W., Saurwalt, J.J.: Inconel-steel multilayers by liquid dispersed metal powder bed fusion: microstructure, residual stress and property gradients. Addit. Manuf. 32 , 101027 (2020)
Zhao, J.-R., Hung, F.-Y., Lui, T.-S.: Microstructure and tensile fracture behavior of three-stage heat treated inconel 718 alloy produced via laser powder bed fusion process. J. Market. Res. 9 , 3357–3367 (2020)
Li, B., Han, C., Vivegananthan, P., Kripalani, D.R., Tian, Y., Bartolo, P.J.D.S., Zhou, K.: Refined microstructure and ultrahigh mechanical strength of (TiN+ TiB)/Ti composites in situ synthesized via laser powder bed fusion. Addit. Manuf. Lett. 3 , 100082 (2022)
Zhang, F., Saleh, E., Vaithilingam, J., Li, Y., Tuck, C.J., Hague, R.J.M., Wildman, R.D., He, Y.: Reactive material jetting of polyimide insulators for complex circuit board design. Addit. Manuf. 25 , 477–484 (2019)
Simonelli, M., Aboulkhair, N., Rasa, M., East, M., Tuck, C., Wildman, R., Salomons, O., Hague, R.: Towards digital metal additive manufacturing via high-temperature drop-on-demand jetting. Addit. Manuf. 30 , 100930 (2019)
Reijonen, J., Revuelta, A., Riipinen, T., Ruusuvuori, K., Puukko, P.: On the effect of shielding gas flow on porosity and melt pool geometry in laser powder bed fusion additive manufacturing. Addit. Manuf. 32 , 101030 (2020)
Qiu, T., Palagi, S., Fischer, P.: 3D-printed soft microrobot for swimming in biological fluids. In: 2015 37th Annual International Conference of the IEEE Engineering in Medicine and Biology Society (EMBC), IEEE, 2015: pp. 4922–4925
Download references
The present research work has not received external funding.
Author information
Authors and affiliations.
Department of Mechanical Engineering, Muzaffarpur Institute of Technology, Muzaffarpur, 842003, Bihar, India
Ashish Kumar Srivastava
Department of Mechanical Engineering, School of Engineering & Technology, JECRC University, Jaipur, 303905, Rajasthan, India
Department of Mechanical Engineering, Rawal Institute of Engineering and Technology, Faridabad, 121004, Haryana, India
Parveen Kumar
Faculty of Mechanical Engineering, VŠB - Technical University of Ostrava, Ostrava, Poruba, 70800, Czech Republic
Preeti Gautam
Department of Orthodontics, Faculty of Dental Sciences, SGT University, Gurugram, Haryana, India
Namrata Dogra
You can also search for this author in PubMed Google Scholar
Corresponding author
Correspondence to Ajay Kumar .
Ethics declarations
Conflict of interest.
The authors declare no competing interests.
Additional information
Publisher's note.
Springer Nature remains neutral with regard to jurisdictional claims in published maps and institutional affiliations.
Rights and permissions
Springer Nature or its licensor (e.g. a society or other partner) holds exclusive rights to this article under a publishing agreement with the author(s) or other rightsholder(s); author self-archiving of the accepted manuscript version of this article is solely governed by the terms of such publishing agreement and applicable law.
Reprints and permissions
About this article
Srivastava, A.K., Kumar, A., Kumar, P. et al. Research Progress in metal additive manufacturing: Challenges and Opportunities. Int J Interact Des Manuf (2023). https://doi.org/10.1007/s12008-023-01661-6
Download citation
Received : 06 August 2023
Accepted : 13 November 2023
Published : 12 December 2023
DOI : https://doi.org/10.1007/s12008-023-01661-6
Share this article
Anyone you share the following link with will be able to read this content:
Sorry, a shareable link is not currently available for this article.
Provided by the Springer Nature SharedIt content-sharing initiative
- Additive manufacturing
- Metal additive manufacturing
- Powder-based fusion
- Sheet lamination
- Material jetting
- Direct energy deposition
- Binder jetting
- Find a journal
- Publish with us
- Track your research

IMAGES
COMMENTS
This review will help readers understand the different aspects of additive manufacturing and explore new avenues for future research. Manufacturing processes The evolution of industries depends on innovative and cutting-edge research activities associated with manufacturing processes, materials, and product design.
In today's era, additive manufacturing (AM) is attracting unparalleled attention across the globe. From initial obscurity, today there is practically no sphere of life untouched by this technology. The quantum of research in this field has witnessed overwhelming growth which in turn leads to impressive newer developments at almost regular intervals. AM has emerged from rapid prototyping and ...
Affiliated with America Makes, the National Additive Manufacturing Innovation Institute Additive Manufacturing is the peer-reviewed journal that provides academia and world-leading industry with high quality research papers and reviews in additive manufacturing. The journal aims to acknowledge the innovative nature of additive manufacturing and its broad applications to outline the current and ...
Additive manufacturing (AM), often referred to as rapid prototyping, freeform fabrication, or 3D printing, is defined as "the process of joining materials to make objects from 3D model data, usually layer upon layer, as opposed to subtractive manufacturing methodologies" like machining [1, 2].Various industries refer to the practice of swiftly producing a physical model of a part or system ...
Progress in Additive Manufacturing is a scientific journal focusing on original and significant research in established and emerging Additive Manufacturing processes and technologies. Offers a platform for academia and industry with technically and industrially relevant results. Encourages manuscripts that discuss the contribution to the ...
This comprehensive article examines the most recent advances and problems in additive manufacturing. The present paper provides a concise overview of the fundamental principles and methodologies of additive manufacturing. Subsequently, it delves into the latest advancements and cutting-edge technologies in this domain, including multimedia ...
This paper aims to present a survey of state of the art in 3D printing technology, covering the most popular processes, materials, developments, and industrial applications. ... This new technology makes it easier and faster to build complex structures. ... A bibliometric indicators analysis of additive manufacturing research trends from 2010 ...
This Special Issue aims to present the latest developments in additive manufacturing processes, optimizations, new additive processes, rapid tooling, and applications from industry using metal powders as raw materials. 2. Contributions.
The last two decades have seen enormous gains in industrial adoption of additive manufacturing (AM) technologies. Its layer-wise approach to fabrication offers designers the opportunity to create structures with unique performance advantages over their traditionally manufactured counterparts, and have created new manufacturing business models and supply chains. While today's AM technologies ...
Additive Manufacturing (AM) is, undoubtedly, one of the most promising and potentially disruptive technologies of the Industry 4.0 era, able to transform the traditional manufacturing paradigm and fuel the generally accepted and necessary shift towards the conceptualisation, design and adoption of sustainable and circular business models. The objective of this paper is to contribute to the ...
Additive Manufacturing Research and Innovation Laboratory, Tennessee Tech University, Cookeville, TN 38505, USA. 2. ... This unique paper is structured to provide the latest trends and practices collected from a number of reliable sources. The keywords used in the literature review process are framed around energy, AM, and their combinations.
ce/papers - Proceedings in Civil Engineering offers rapid publication of proceedings of scientific conferences, workshops & events. Abstract Additive manufacturing (AM) is the industrial production name for 3D printing, a computer-controlled process that creates three dimensional objects by deposing materials, usually in layers...
Abstract. Additive Manufacturing (AM) processes (1) Fused deposition modeling (FDM) (2) Stereo. lithography (SLA) (3) Selective laser sintering /melting (S LM) (4) Laminated object. Manufacturing ...
The main objective of the paper is to classify the latest knowledge for the researchers, along with highlighting challenges and future research directions in this field. Despite the rapid growth in the past decade, the industrial adoption of additive manufacturing has not still been achieved due to certain limitations.
this review describes the latest research conducted on petroleum-based polymers and their composites ... Additive manufacturing (AM) is designated as one of the revo-lutionary innovations since the 19th century, which signi - ... including 420 peer-reviewed journal papers, 21 book chapters, and 53 patents. She was the editor or co-editor of 4
5. Conclusions The present paper presents an updated review of the latest trends in additive manufacturing. Initially, a straightforward presentation of additive manufacturing and its advantages and limitations was introduced. And, finally, some of the newest advances and research topics in additive manufacturing were presented.
In recent years, Additive Manufacturing (AM), also called 3D printing, has been expanding into several industrial sectors due to the technology providing opportunities in terms of improved functionality, productivity, and competitiveness. While metal AM technologies have almost unlimited potential, and the range of applications has increased in recent years, industries have faced challenges in ...
Now researchers are stepping towards 4D printing which uses "Intelligent" materials which can be coded. Research work is being done on different Additive manufacturing techniques and different materials and process parameters. This paper is a review of Additive manufacturing and it's latest trends.
Top research papers on Additive Manufacturing. 1. Additive Manufacturing (AM) at Industry 4.0: A Review by Diogo José Horst (2018) - This paper presents the fundamental principles of 3D printing, its roles in industry 4.0 in saving time and cost, and the benefits, e.g., higher flexibility and individualization. 2.
Melding expertise in materials science, additive manufacturing, optical engineering and data science, they added photodiodes at multiple wavelengths and increased the sample frequency to measure ...
"And additive manufacturing tools can do this as needed, rather than dealing with supply chains that can have long wait times. That usually means using 3D printing to create small batches of machine parts on demand." McConnell is an assistant research professor in NC State's Edward P. Fitts Department of Industrial and Systems Engineering.
2. Classification of additive manufacturing technologies. There are many AM processes commercially available today. Different researchers have classified AM techniques in different ways [3, 6, [50], [51], [52]].One of the common classifications is on the basis of ASTM-F42 committee guidelines according to which AM can be classified into seven categories.
Machine learning (ML) has recently found compelling applications in the manufacturing and materials industries. With this state-of-the-art technology, materials innovation, critical simulations for product manufacture, and predictive insights that can significantly improve overall production efficiency are all being accomplished. Implementing a robust ML model promises to minimise the need for ...
"One of the reasons people are attracted to 3D printing and other additive manufacturing technologies is that these technologies allow users to quickly replace critical machine components that are otherwise difficult to make outside of a factory," says Brandon McConnell, co-corresponding author of a paper on the work. "And additive ...
Ultrasonic additive manufacturing (UAM) is a new subclass of LOM which combines ultrasonic metal seam welding and CNC milling in the lamination process [29]. UAM is the only additive manufacturing method that is capable of construction of metal structures at low temperature [30, 31]. LOM has been used in various industries such as paper ...
The dusting potential of three batches of the additive was determined using the Stauber-Heubach method and showed values in the range 1-3 mg/m 3. The same batches were tested for particle size distribution by laser diffraction method; the results showed that all particles had a diameter above 250 μm. 18. 3.1.3 Manufacturing process
The revolutionary changes in aircraft and automobile industries persuaded the rapid productional technology which introduced additive manufacturing of three-dimensional products. The layer-by-layer development techniques with the uniqueness of producing intricate shapes with negligible wastage of material make it famous for many sectors like defence, automobile, aircraft, medical etc. The ...
This paper provides a comprehensive review of metal additive manufacturing in the aerospace industry (from industrial/popular as well as technical literature). This provides a current state of the art, while also summarizing the primary application scenarios and the associated commercial and technical benefits of additive manufacturing in these ...
This could lead to additive manufacturing material and property options that do not exist today. These research areas provide exciting opportunities for further exploitations of mechanical alloying technology, and powder metallurgy in general, towards developing new products to meet the needs of customers in global markets such as within the ...